Fig. 26.1
Normal parietal (a, b) and visceral (c, d) pleura as shown by white-light thoracoscopy (WLT) and autofluorescence thoracoscopy (AT), respectively
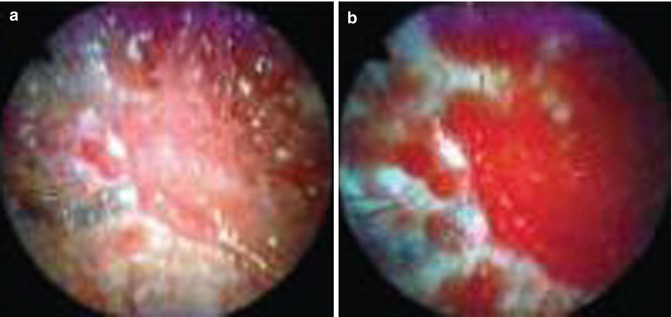
Fig. 26.2
Breast cancer metastasis to the parietal pleura as shown by (a) white-light thoracoscopy (WLT) and (b) autofluorescence thoracoscopy (AFT). During WLT the colour of the malignant tissue is light pink. During AFT the malignant tissue is deep red, whereas the normal tissue has turned white. The line between the normal and malignant tissue is well demarcated (From Chrysanthidis and Janssen (2005))
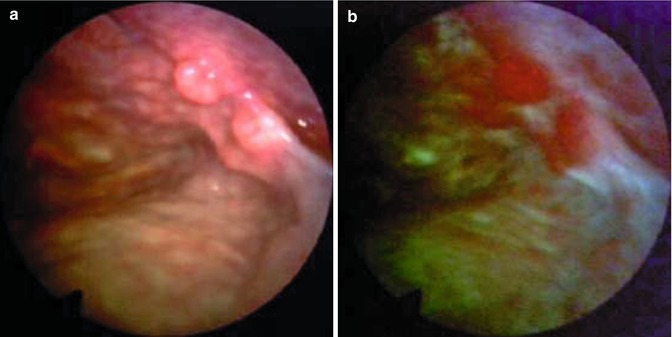
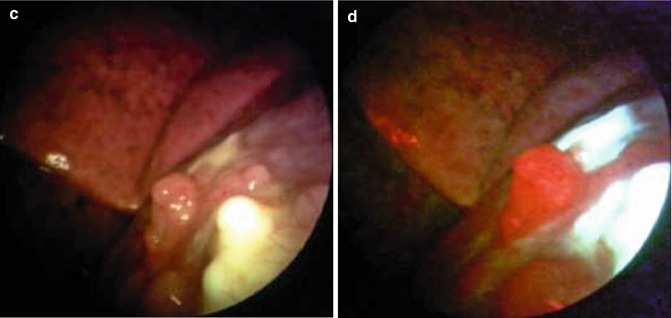
Fig. 26.3
Malignant pleural mesothelioma detected in occupational asbestos-exposed patient by white-light source (a, c) and autofluorescence thoracoscopy (b, d) (true positive) (Courtesy D Fielding, Department of Thoracic Medicine, Royal Brisbane and womens’ Hospital, Brisbane, Queensland, Australia)
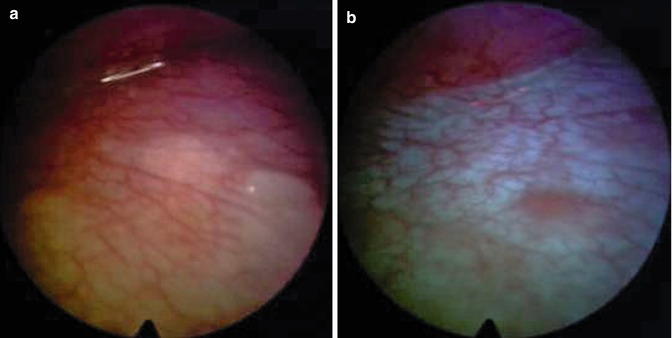
Fig. 26.4
Malignant pleural mesothelioma. True negative on the diaphragm assessed by white-light source (a) and autofluorescence thoracoscopy (b)
The study of Chrysanthidis and Janssen had two important limitations: (a) patients had extended pleural disease at the time of medical thoracoscopy was done and therefore it was easy to diagnose malignancy with WLT-guided biopsies alone. However, the findings using blue-light mode were more obvious, and the same malignant lesions were more precisely mapped during AFVT; (b) biopsies were obtained from a rather restricted number of sites on the pleural space, and it was practically impossible to have specimens from the entire pleural surface with the goal of obtaining a reliable value of false negatives to precisely estimate the sensitivity of the method (e.g. the ability to find a lesion if there was one).
26.3.2.2 The Initial Experience
The initial experience of performing AFVT, using the same DAFE® fluorescence endoscopy system, was also recently published (Belák et al. 2007). According to the researchers, the tissue autofluorescence response originates mostly from the subepithelial tissue, and local hypertrophy “obstructs” the penetration of the blue-violet light into the tissue. As a response to the decreased intensity of the excitation light, the intensity of the autofluorescence emission is also decreased.
The resulting indirect autofluorescence image represents the following: (a) healthy tissue with physiologic concentration of collagen and elastic fibres presenting with a white-green colour; (b) a low-density blue colour from deeper layer (1–2 mm) tissue with increased concentration of haemoglobin or inflammatory infiltrates; (c) a black or grey colour from tissue with increased superficial concentration of haemoglobin [e.g. stained with blood tissues], as well as superficially localised vessels; and (d) a vivid high-density blue foci from tissue with a roughened surface due to hyperplastic inflammatory processes (pachypleuritis, adhesions) and malignant or dysplastic processes.
The AFVT technique is reported to be more precise in locating pleural disorders. In another group of 43 patients who underwent AFVT in 1 year – from March 2007 to March 2008 – a malignant diagnosis was made in 30 cases with 9 cases of inflammatory pleural disease and 4 cases of diffuse lung impairment.
Both these autofluorescence videothoracoscopy studies have provided initial or preliminary results. The authors underline the necessity of conducting further studies using AFVT in order to clarify the real, precise value of this method in clinical practice and to include more cases with earlier stage malignant pleural disease.
26.3.3 Fluorescence Detection of Pleural Malignancies Using 5-ALA
In 2006, a feasibility study of the impact of 5-aminolaevulinic acid (ALA)-mediated fluorescence diagnosis (FD) on the diagnostic workup and staging of various malignancies during VATS was published by researchers from the Netherlands Cancer Institute/Antoni van Leeuwenhoek Hospital and the Laser Center in Academic Medical Center of University of Amsterdam (Baas et al. 2006).
The theoretical basis of the study was focused on the known metabolic pathway of 5-ALA into haemoglobin, where the rate-limiting step is the presence of ferrochelatase. The expression of this enzyme, required in the final stage of transformation of 5-ALA to haemoglobin, is found to be decreased in malignant and inflamed cells, leading to an accumulation of Pp-IX, which has photodynamic properties. The increase in concentration of intracellular Pp-IX and the associated differences in pharmacokinetics between abnormal cells and normal cells facilitate the use of 5-ALA for diagnostic or therapeutic purposes. 5-ALA can be administered systemically (per os or intravenous) or locally into the pleural space and has relatively few side effects – skin photosensitivity for 24–36 h and a transient rise of liver enzymes.
For the purposes of FD (PDD), 5-ALA was administered with ample amounts of water 3–4 h prior to thoracoscopy. Three dosage groups were defined: 1,500 mg for patients with a weight less than 60 kg, 2,000 mg for patients 60–80 kg and 2,500 mg for patients with a weight more than 80 kg. Fluorescence images were obtained using the D-Light® autofluorescence system (Karl Storz – Tuttlingen, Germany), and both illumination and observation of the tissue of interest were achieved with a rigid endoscope integrated with a long-pass filter (cut-off wavelength at 470 nm). The camera had a white-light mode and a blue-light mode, in which the integration time could be increased to correct the relative low intensity of the fluorescent light. An additional low-pass filter (>550 nm) was placed between the endoscope and the camera to further increase the contrast of the images.
From January 2003 to January 2005, 25 patients with a non-diagnosed pleural effusion were included in the analyses. All these patients underwent FD thoracoscopy under general anaesthesia. A final diagnosis was obtained in 24 patients. The definitive diagnosis included 15 cases of malignant mesothelioma, 5 cases of metastases (bronchoalveolar, tongue, mammary, oesophagus and malignancy of unknown origin), 3 cases of plaques with or without inflammatory changes and 1 case of empyema. The undiagnosed case was conformed as a mesothelioma after 6 months of follow-up.
In total, 111 biopsy specimens were obtained from parietal, visceral and diaphragmatic pleura during the examination, and an impressive classification into three equal groups was subsequently formed: (a) both FD and white-light imaging (WLI) results were positive in 37 biopsies, 7 of which were found to be false positives; (b) FD and WLI results were negative in 37 biopsies, 13 of which were proven false negatives; and (c) in the remaining 37 biopsies, there was a discrepancy between observations with WLI and FD. In 26 of the discrepant biopsies, fluorescence detection was positive and white light was negative, with 19 true positive and 7 false positive results. FD was negative in 8 biopsies, the majority of which were taken from a patient with desmoplastic mesothelioma. WLI results were positive over FD in 11 biopsies, with 8 true positive and 3 false positive results.
A detailed analysis of the findings in the 37 biopsies specimens with discrepancies between WLI and FD imaging results showed that there was no overall diagnostic improvement obtained by using FD. Therefore, the researchers demonstrated only a limited benefit of 5-ALA fluorescence for the individual patient. However, for the staging of mesothelioma, it was clear that in four patients, FD upstaged the patient due to improved visualisation of lesions on the visceral pleura which could not be seen using WLI alone, and it was felt that the whole staging process was facilitated by the fluorescence thoracoscopy.
In the future, higher doses of 5-ALA could be administered for imaging if local photodynamic therapy is also indicated for the treatment of the identified tumours (Stanzel et al. 2000; Baas et al. 2006). To date, this option has not been investigated due to the limited access of the thoracoscope in the chest cavity and the diffuse growth of pleural malignancies.
26.4 Fluorescein-Enhanced Autofluorescence Thoracoscopy in Primary Spontaneous Pneumothorax
Marc Noppen and colleagues performed the first prospective, controlled study of in vivo visualisation of abnormal lung regions which may play an important role in the pathophysiology of primary spontaneous pneumothorax (PSP). This study used fluorescein-enhanced autofluorescence thoracoscopy (FEAT) compared to white-light thoracoscopy (WLT) in patients with PSP and in normal control subjects (Noppen et al. 2006).
FEAT was conceptualised to try to visualise abnormal areas at the visceral lung surface and identify sites of potential air leakage. The idea of using a “dye” to make air leaks visible during thoracoscopy was first discussed by C. Boutin in his reference book “Practical Thoracoscopy” (Boutin et al. 1991). However, the results of fluorescein inhalation in the setting of white-light illumination for detecting air leaks were disappointing (Boutin C and M Noppen – unpublished observations). Illumination with blue light, taking advantage of the physical fluorescence properties of fluorescein, appeared to dramatically increase its sensitivity.
Sodium fluorescein is a relatively low-molecular-weight, highly water-soluble compound, which when exposed to light of wavelength 465–490 nm [blue light, corresponding to the wavelength used by the DAFE® system] emits light at a wavelength of 520–530 nm [green-yellow light, also detectable by the DAFE® system]. During 30 years of clinical use in the diagnosis and treatment of retinal vascular disorders, the intravenous injection of fluorescein has been shown to be safe. Inhalation of fluorescein had not been reported in controlled series prior to 2004, when the same researchers administered a fluorescein aerosol to a patient with PSP, followed by WLT and FEAT (Noppen et al. 2004). This first case report demonstrated several areas of subpleural fluorescein accumulation not detected by normal WLT. In the subsequent study, all subjects inhaled an aerosolised 10 % fluorescein solution for approximately 10 min under normal tide volume conditions. The fluorescein aerosol was delivered via a pressure-driven nebuliser, attached to a mask. All patients underwent total intravenous anaesthesia, with single-lumen intubation and high-frequency jet ventilation via an endotracheal tube.
During a 6-month period, 12 consecutive patients presented with recurrent and/or persistent (air leak for more than 4 days) PSP. These patients were suitable for thoracoscopic treatment and were enrolled in the study. In addition, 17 control subjects were recruited during the same period. These subjects were referred for bilateral thoracoscopic sympathicolysis for the treatment of essential hyperhidrosis (16 cases) or idiopathic pathologic flushing/social phobia syndrome (1 case). PSP lungs showed significantly more abnormalities at WLT when compared with normal lungs, including the presence of emphysema-like changes (ELCs) like blebs and/or bullae. FEAT showed low-grade anthracotic lesions in the majority of normal lungs. However, in contrast high-grade lesions with extensive subpleural fluorescein accumulation and fluorescein leakage were exclusively found in patients presenting with PSP. It is important to note that these high-grade lesions, which may be considered as possible sites where air leakage can originate, were not necessarily associated with blebs or bullae or other associated abnormalities visible at WLT. These findings suggest that the observed high-grade FEAT phenomena, limited to PSP lungs only, may be proof that PSP patients suffer from a more extensive disease process of parenchymal inflammation and destruction than previously thought. It also confirms that ELC rupture is not necessary for every case of PSP. Finally these finding have major therapeutic implications relating to the definitive treatment of the ELC, as in many cases abnormalities were found distant from the blebs and bullae, which were previously thought to be the primary culprits of air leakage in PSP.
Nevertheless, the pathophysiological significance of these observations remains to be further explored and analysed, while it is important to acknowledge the limitations inherent to this technique: (a) the FEAT data can only be obtained in a semiquantitative fashion, because the fluorescence measurements are planar measurements with the light intensity dependent on the local concentrations of fluorophore and on the depth of penetration of the fluorophore in the lung tissue; (b) the semiquantitative scoring is entirely unblinded, as the thoracoscopist was fully aware of whether the patient had suffered from a PSP or was a control subject, and this highlights the need for improved score blinding and a more objective method of fluorescence measurement in the future; and (c) the kinetics of inhaled fluorescein with respect to absorption in the bloodstream, interstitium or cells are unknown. Therefore, the hypothesis that high-grade lesions, exclusively seen in PSP and not in normal lungs, may be the result of the accumulation of inhaled fluorescein aerosol in certain pulmonary areas due to local air trapping, which needs to be further studied. Alternatively, fluorescein may accumulate in areas that receive more rather than less ventilation. However, the study of the wash-in and wash-out phases of fluorescein administration in a patient or an animal model would help clarify the true meaning of the fluorescein accumulation.
The use of FEAT in patients with PSP demonstrated the existence of actual fluorophore leakage through the visceral pleura. This can be explained by the presence of pleural porosity (e.g. the presence of pleural pores with a width of several microns) in the absence of blebs, bullae or other abnormalities observed at white-light thoracoscopic inspection. The exact nature of the pleural porosity is also unknown but may be associated with the loss of surface mesothelial cells, thinning and rupture of the basement membrane and/or downregulation of junctional proteins. It is possible that an interplay between peripheral airway and airspace inflammation (that may be enhanced by smoking), mechanical factors (such as more negative surrounding pressures and increased mechanical stretching of the lung apices in ectomorphic individuals) and differentiation in collagen quality may lead to the structural changes defined as “pleural porosity”.
26.5 Newer Fluorescence Techniques in Endoscopic Diagnosis, Staging and Therapy
Fluorescence techniques are continuously evolving and have already been implemented in several areas of clinical practice. In addition the technique may be combined with different methods for diagnosis and therapy in the future. In this chapter, we attempt to show brief characteristics of some of the most important research activities on the field of fluorescence diagnosis, staging and therapy in respiratory endoscopy.
26.5.1 Near-Infrared Thoracoscopy of Tumoural Protease Activity for Improved Detection of Peripheral Lung Cancer
Researchers from the Centre for Molecular Imaging Research in Massachusetts General Hospital, Boston, USA, have recently attempted to evaluate the ability to use activated probes targeting proteases which are overexpressed in many tumour types, in order to improve the visualisation of parenchymal-surface lung tumours during thoracoscopy (Figueiredo et al. 2006).
Direct visualisation of the pleural space and lung surface at the time of video-assisted thoracoscopic surgery (VATS) offers a higher spatial resolution than non-invasive cross-sectional imaging modalities, such as CT or MRI. Therefore, micrometastatic disease not identified by these modalities may, in some cases, be seen directly. In addition the luminescence of tumours, especially small foci, is often similar to the adjacent lung parenchyma. The basic objective of the study was to determine the possibility of providing adjuncts to VATS, which could result in better visualisation of tumours and would be beneficial in increasing the sensitivity of lesion detection as well as allowing for a shorter procedure duration by decreasing the time needed to adequately inspect the pleural space and identify areas that require sampling and removal.
Activatable fluorescent imaging agents typically increase their fluorescence intensity after target interaction: when initially injected, they are optically silent, but after interaction with enzymes that are overexpressed in tumours, they become brightly fluorescent. Because of their low initial fluorescence, which results in lower background signal, higher target to background ratios (TBR) can be achieved when compared to other fluorescent agents, allowing easier detection of tumour foci. Additionally, compared to fluorophores, which are often of low molecular weight, the activatable agents remain at their target for longer periods of time, allowing detection of the probe during long procedures. Many of the activatable agents fluoresce in the near-infrared region (NIR) of the electromagnetic spectrum, and this results in several advantages: (a) deeper penetration of NIR signal when compared to visible light, allowing the visualisation of pathology below the surface, and (b) a simultaneous acquisition of full-colour white-light images, as seen during conventional thoracoscopy, combining with NIR images, thus provide information on molecular activity. These can be overlaid onto the white-light anatomical images to guide the surgeon during a procedure. Two different near-infrared fluorescent activatable “smart” imaging probes were used in this study, which differed in the attached fluorochrome and in peak absorption and emission wavelengths – one probe with a peak absorption of 680 nm and peak emission of 700 nm [ProSense 680] and another activatable probe with peak absorption of 750 nm and peak emission of 780 nm [ProSense 750].
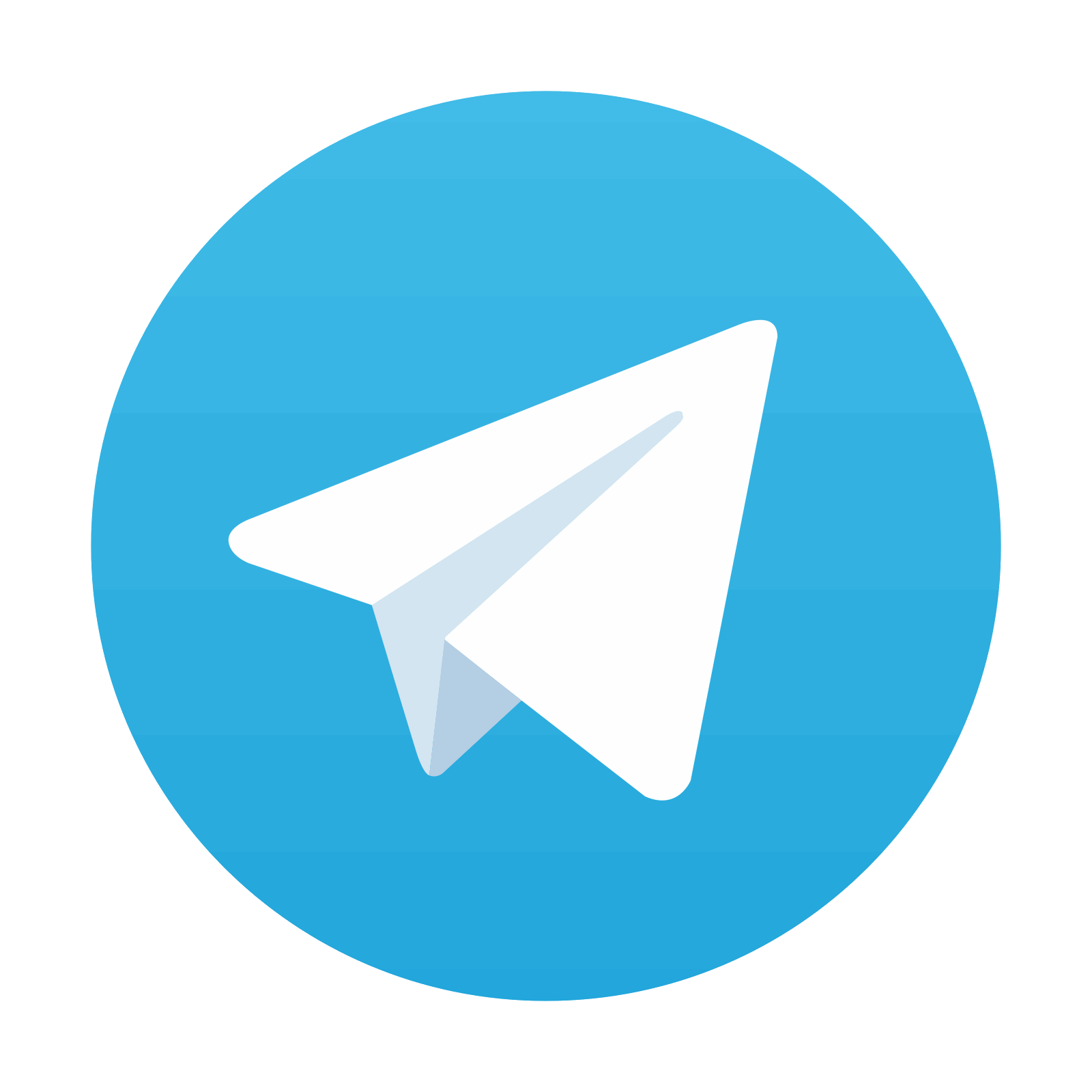
Stay updated, free articles. Join our Telegram channel

Full access? Get Clinical Tree
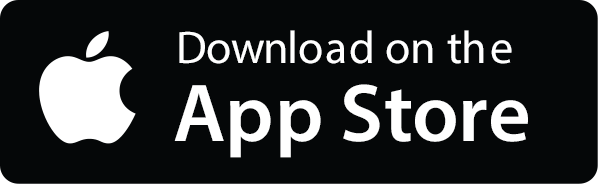
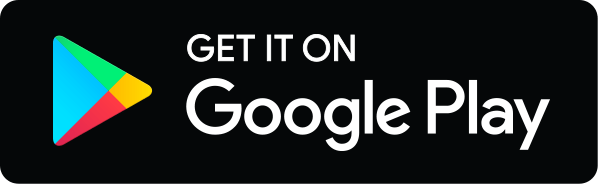