Atrioventricular Conduction
The usefulness of intracardiac recording and stimulation techniques in humans was first realized during its application to patients with disorders of atrioventricular (A-V) conduction. Electrophysiologic study allowed confirmation and further elucidation of the underlying mechanisms and site of block suspected indirectly by surface ECG. The A-V block has been traditionally classified by criteria combining implications about anatomic site, mechanism, and prognosis. Analysis of A-V conduction from the surface ECG is confined to (a) the duration and shape of the P wave, (b) the duration and pattern of alternation of the P-R interval, (c) the QRS morphology and the duration of either conducted beats or escape rhythms, and (d) the rate of escape rhythms (if present). Because intracardiac studies can provide much of this information directly, a classification of A-V block that is applicable to the A-V conduction system as a whole or to any of its components is preferred (Table 4-1). Thus, in our laboratory, we prefer to consider first-degree, second-degree, and third-degree A-V block as prolonged conduction, intermittent conduction, and no conduction, respectively, because it more accurately defines the underlying physiology. For example, in the presence of first-degree block, impulse transmission (output/input) is 1:1 (hence the inappropriateness of the term block), although conduction through the whole A-V conduction system, or any of its parts, is prolonged; in the presence of second-degree block, impulse transmission is less than 1:1; and in the presence of third-degree block, no impulse transmission occurs.
Second-degree block is further subdivided into Types I and II. Type I second-degree block, to which the eponym Wenckebach block is often applied, is characterized by progressive prolongation in A-V conduction time preceding the nonconducted time in the conducted beat following the blocked impulse. In contrast, Type II second-degree block, to which the eponym Mobitz Type II is often applied, is characterized by sudden failure of conduction without alteration of conduction time of conducted beats either preceding or following the nonconducted one.
El-Sherif et al.1 have described a canine model in which ligation of the anterior septal artery produced an apparent Type II second-degree block in the His bundle. However, careful measurement of intra-His conduction times with stable plunge wire electrodes revealed minimal (1- to 2-msec) increments before the blocked beat, a range that usually falls within the error of measurement of the recording techniques commonly used in humans. Furthermore, a temporal continuum between apparent Type II block and obvious Type I block in the His–Purkinje system has been demonstrated in these canine animals by Simson et al.2 in our laboratory as well as in a few patients with acute myocardial infarction. Thus, the subdivision of second-degree A-V block into two distinct types may not be sound from a mechanistic viewpoint. Nevertheless, from a practical viewpoint it is worth noting that intermittent conduction (second-degree A-V block) associated with no (or undetectable) preceding increment is a behavior characteristic of the His–Purkinje system. Therefore, we shall continue to use the designations Type I block and Type II block as clinically descriptive terms. In the presence of 2:1 or higher-grade intermittent conduction, classification into Type I or II is not possible and is really a category unto itself. Therefore, block greater than second-degree A-V block includes 2:1 A-V block, high-degree A-V block (≥2 consecutive blocked P waves of intermittent conduction), and complete A-V block with evidence.
Theoretically, abnormalities of A-V conduction may result from conduction disturbances in any region of the heart, although certain patterns of block can be localized to specific sites (Table 4-2). Nevertheless, considerable overlap is seen, and the identification of the site of block on the basis of the surface ECG alone may not be possible. In the case of a prolonged P-R interval, intracardiac measurements may show normal intracardiac conduction (e.g., P-Atrioventricular junction [P-AVJ] atrium = 50, A-H = 125, H-V = 55). The chance of one’s developing complete heart block, and its clinical significance, depends on the site of block. Hence, precise localization of a conduction disturbance may aid the clinician in planning the therapeutic approach.
Two questions must be addressed when deciding which patients should be referred for evaluation of A-V conduction disturbances: (a) When is the surface ECG likely to be misleading? (b) When does the localization of a conduction disturbance per se provide therapeutically important information independent of the patient’s symptoms, surface ECG, and so on? Because of the lack of complete data, these questions are often answered on subjective rather than objective grounds; nevertheless, the following paragraphs present
information that may provide some guidelines for the use of intracardiac studies in defining A-V block.
information that may provide some guidelines for the use of intracardiac studies in defining A-V block.
TABLE 4-1 Atrioventricular Block | ||||||||||||
---|---|---|---|---|---|---|---|---|---|---|---|---|
|
Atrium
An impulse originating in the sinus node must traverse the right atrium to reach the A-V node. Although there are no anatomic data supporting the presence of specific internodal tracts (anterior, middle, or posterior) that facilitate internodal conduction,3 preferential pathways of atrial conduction exist, but they appear to be related to the spatial and geometric arrangement of atrial fibers rather than to specialized “tracts.”
Because the sinus impulse can propagate directly through all types of atrial muscle as well as through these proposed preferred pathways, high-grade or complete heart block that is due to atrial disease in humans is virtually unknown. Of interest is the fact that a majority of dogs develop complete A-V block and a junctional rhythm if all three internodal tracts (found in dogs) are sectioned. On the other hand, prolonged A-V conduction (first-degree block) that is due to atrial conduction delay is not uncommon in humans. The ECG pattern of left atrial enlargement (a P wave ≥120 msec in duration with posteriorly and leftward directed terminal forces) is produced by delayed or prolonged left atrial activation rather than left atrial enlargement or hypertrophy in the absence of conduction delay.4 A correlation between left atrial size and ECG left atrial enlargement is best seen in rheumatic and primary myocardial disease and rather poorly seen in coronary artery disease.4 In all instances, however, delayed activation to the lateral left atrium is present. Therefore, so-called electrocardiographic “left atrial enlargement” is better thought of as an interatrial conduction delay (IACD).
TABLE 4-2 Sites of Atrioventricular Block | ||||||||||||||||||||||||||||||
---|---|---|---|---|---|---|---|---|---|---|---|---|---|---|---|---|---|---|---|---|---|---|---|---|---|---|---|---|---|---|
|
The assessment of intra-atrial conduction demands the reproducible and accurate timing of local electrocardiograms from specific anatomic sites in the atria.5 While high-density mapping may be useful to ascertain local atrial defects, fewer points are needed to demonstrate the cause of P-wave abnormalities (in a general way). For the reasons given in Chapter 2, simple measurement of the P-A interval is inadequate for the assessment of intra-atrial conduction. At a minimum, atrial electrograms from the high-right atrium (HRA) in the region of the sinus node, the A-V junction at the His bundle recording site, and the posterior lateral and even anterior left atrium recorded from various positions in the coronary sinus are required. Recordings from other sites along the lateral right atrial wall are easily obtained, whereas recordings from other sites in the left atrium are more difficult to obtain unless an atrial septal defect (ASD) or a patent foramen ovale is present, or a transseptal catheterization or retrograde approach is performed. These latter two procedures are not really justified unless a left atrial tachycardia is suspected. For accuracy and consistency, the principles of measurement described in Chapter 2 must be followed strictly. Accuracy is further enhanced by the use of rapid recording speeds (200 mm/sec or greater). A single catheter may be used to map more than one location as long as the rhythm (usually sinus) remains constant, and a reproducible reference electrogram is chosen (e.g., an intracardiac atrial electrogram and/or P wave in the surface ECG). Normal atrial activation using standard sites is shown in Figure 4-1. The mean activation time in a group of 24 patients with normal P waves and the mean values in a group of 15 patients with electrocardiographic left atrial enlargement are shown in Figure 4-2A, B, respectively. Obviously detailed atrial activation mapping using the Carto electroanatomic system is most accurate and is necessary for ablation of left atrial tachyarrhythmias. Normal activation is shown in Figure 4-3 in which activation of the left atrium occurs over Bachmann bundle, with additional conduits through the interatrial septum and coronary sinus (and potentially via interatrial bridges). In contrast, Figure 4-4 shows an atrial activation using standard catheter positions in a patient with a P wave that meets the standard ECG criteria for left atrial enlargement. In this patient, delayed activation of the left atrium (P to coronary sinus) and normal intra-right atrium conduction are shown by the atrial map. Biatrial conduction delay in standard catheter positions is shown in Figure 4-5.
Intra-atrial delay involving only the right atrium is far less common, but it is seen in the presence of congenital heart disease, particularly the endocardial cushion defects6 and Ebstein anomaly of the tricuspid valve.7 In approximately 20% of
patients with prolonged A-V conduction and congenital heart disease, the mechanism is prolonged intra-right atrial conduction.8 In these situations, prolonged P-A and HRA to low-right atrium intervals are frequently encountered. “First-degree A-V” block has been reported in as many as 50% of patients with endocardial cushion defects; it may be due entirely or in part to a delay in intra-atrial conduction, with a defect involving the atrionodal junction. Of five patients with Ebstein anomaly studied by Kastor et al.,7 three had prolonged P-A and intra-right atrial conduction intervals and all had broad (≥110 msec) P waves. The prolonged intra-right atrial conduction time was probably due to longer time necessary to traverse the abnormally large right atrium. We have also seen a patient with an ASD with a P-A interval measured at the AVJ of 110 msec. The demonstration that intra-atrial conduction delay is responsible for prolonged A-V conduction (first-degree A-V block) may alleviate concern that A-V nodal delay, intra-His delay, or infra-His delay is responsible.
patients with prolonged A-V conduction and congenital heart disease, the mechanism is prolonged intra-right atrial conduction.8 In these situations, prolonged P-A and HRA to low-right atrium intervals are frequently encountered. “First-degree A-V” block has been reported in as many as 50% of patients with endocardial cushion defects; it may be due entirely or in part to a delay in intra-atrial conduction, with a defect involving the atrionodal junction. Of five patients with Ebstein anomaly studied by Kastor et al.,7 three had prolonged P-A and intra-right atrial conduction intervals and all had broad (≥110 msec) P waves. The prolonged intra-right atrial conduction time was probably due to longer time necessary to traverse the abnormally large right atrium. We have also seen a patient with an ASD with a P-A interval measured at the AVJ of 110 msec. The demonstration that intra-atrial conduction delay is responsible for prolonged A-V conduction (first-degree A-V block) may alleviate concern that A-V nodal delay, intra-His delay, or infra-His delay is responsible.
![]() FIGURE 4-3 Right and left atrial activation defined by high-density electroanatomic mapping. See section “Atrium.” |
![]() FIGURE 4-5 Biatrial conduction delay. A single P wave is shown. Note the delayed activation of all atrial electrograms. T, time line. |
Second-degree intra-atrial block is rare. It occasionally occurs spontaneously as Type I second-degree exit block from an automatic atrial focus in the setting of digitalis toxicity (Fig. 4-6). A similar phenomenon can be precipitated in the laboratory by rapid atrial pacing, which results in progressive increments in the stimulus-to-A interval until the stimulus is not propagated, at which time the process repeats itself.9 Additionally, Castellanos et al.10 have reported on two patients in whom a progressive increment in the interval from the HRA to the low-right atrium (recorded at the A-V junction) occurred until the beat was dropped. Such types of intra-atrial delays appear to be substrates for intra-atrial reentry (Chapters 8, 9).
Type II second-degree block and complete heart block do not occur as manifestations of atrial conduction disease in humans during sinus rhythm. Interatrial and intra-atrial dissociation have been observed during atrial tachyarrhythmias, particularly atrial flutter and fibrillation. In such instances, all or some part of the atrium manifests one rhythm while the remainder is activated differently.
Atrioventricular Node
The A-V node accounts for the major component of time in normal A-V transmission. The range of normal A-H time during sinus rhythm is broad (60 to 125 msec), and it can be profoundly influenced by changes in autonomic tone. The same is true of all the other measurements of A-V nodal function (e.g., refractory periods), thereby rendering the laboratory evaluation of intrinsic (as opposed to physiologic) A-V nodal function difficult. Thus, measurements of A-V nodal function over periods of time may not be reproducible.11 One may chemically denervate the A-V nodal node (i.e., 0.04 mg/kg atropine and 0.02 mg/kg propranolol to better assess intrinsic A-V nodal function). The clinical utility of such measurements is unknown.
Delay in the A-V node is by far the most common source of prolonged A-V conduction (first-degree A-V block) (Fig. 4-7).12 The magnitude of delay can vary greatly, but it can be enormous. In our laboratory, we have observed A-H intervals as long as 950 msec. From another viewpoint, most patients (≥95%) with a total P-R interval greater than 300 msec have some degree of A-V nodal delay. It is worth reemphasizing that A-V conduction can vary greatly with changes in the
patient’s clinical state (i.e., influence of autonomic nervous system) and in response to pacing-induced changes in heart rate in a characteristic fashion (Chapter 3), with a prolongation in conduction time and refractoriness at increasing paced heart rates. On the other hand, clinical states with heightened sympathetic tone are accompanied by decreased A-V nodal and conduction time and refractoriness at any paced rate.
patient’s clinical state (i.e., influence of autonomic nervous system) and in response to pacing-induced changes in heart rate in a characteristic fashion (Chapter 3), with a prolongation in conduction time and refractoriness at increasing paced heart rates. On the other hand, clinical states with heightened sympathetic tone are accompanied by decreased A-V nodal and conduction time and refractoriness at any paced rate.
Second-degree block that is due to intermittent failure of conduction through the A-V node is very common in a wide variety of circumstances. The A-V node is the site of most Type I second-degree blocks. Type I second-degree block may be a manifestation of a pathologic process, or it may be a physiologic response within normal limits. This type of block may occur in the setting of any acquired or congenital disease of the A-V node, especially inferior wall myocardial infarction. It can also be precipitated by drugs such as digitalis, beta blockers, calcium channel blockers, or amiodarone. In addition, it may be seen in the young, healthy heart at rest in the presence of high levels of resting vagal tone, a state that is particularly common in well-trained endurance athletes. Furthermore, Type I second-degree block can be precipitated in almost all subjects by incremental atrial pacing (Chapter 2) and in an analogous fashion may be seen during atrial tachycardia.
![]() FIGURE 4-6 Automatic atrial tachycardia with exit Wenckebach block. Atrial tachycardia with regularly irregular (i.e., group beating) atrial activity associated with complete heart block is present in a patient with digitalis intoxication (serum digoxin level = 3.8 ng/mL). Note the progressively decreasing A-A intervals followed by a pause and then resumption of the pattern. Hr are retrograde His bundle potentials associated with fascicular depolarizations (Chapter 7), another manifestation of digitalis intoxication. |
The easily recognized typical Wenckebach periodicity (Fig. 4-8), with a gradual prolongation of each succeeding conduction interval with decreasing increments, may be relatively uncommon in clinical practice. More often, Wenckebach periodicity is atypical.13 It is not uncommon to observe (a) the A-H interval stabilize for several beats, especially during long Wenckebach cycles (11 to 10, etc.); (b) the greatest increment occurs with the second beat after the pause; and (c) very little incremental conduction delay after a large initial “jump” (dual A-V nodal pathways present, see below and in Chapter 8, A-V nodal reentry), or only a relatively small overall increment in A-H associated with a modest decrease in A-H following the pause (Fig. 4-9). This latter phenomenon is often seen associated with an increased vagal tone. As shown in Figure 4-9, this usually is accompanied by slowing of the sinus rate. Typical Wenckebach periodicity is more frequently observed during pacing-induced second-degree A-V block (Fig. 4-10). In patients with dual A-V nodal pathways, Wenckebach cycles are almost always atypical. The greatest jump in A-H occurs when block in the fast pathway occurs, whichever complex this may be. In patients with A-V nodal reentry, Wenckebach cycles may be terminated by A-V nodal echoes or the development of supraventricular tachycardia (Chapter 8). These should really not be called Wenckebach cycles because there is no blocked paced impulse.
![]() FIGURE 4-7 First-degree block (prolonged conduction) confined to the A-V node. The A-H interval is prolonged to 250 msec. The H-V interval is 45 msec (normal). |
Type II second-degree A-V block occurring in the A-V node has been reported,14 but in each case, either the block could have been localized to a more likely site (usually the His bundle rather than the A-V node) or the block probably was atypical Type I, as suggested by a decrease in the A-H interval of the beat following the blocked impulse. One of the more common situations in which “apparent” Type II A-V nodal block can be seen is during heightened vagal tone during sleep. A clue to this phenomenon is associated sinus slowing (Fig. 4-9). Another rare cause of apparent Type II block with normal QRS complexes is concealed His bundle extrasystoles.15 In my opinion, true Type II second-degree block occurring in the A-V node has never been adequately documented.
Second-degree A-V block in the A-V node can often be partially or completely reversed by altering autonomic tone. Hence, exercise (or other measures to increase sympathetic tone) or the administration of atropine (to decrease vagal tone) may produce reversion to 1:1 conduction. However, occasionally in the presence of structural damage (e.g., congenital heart block or inferior myocardial infarction), these measures may be ineffectual. In such cases spontaneous progression to third-degree block (complete A-V block) may occur. More than likely, in most instances, failure of conduction to improve following atropine or isoproterenol suggests block is probably high in the His bundle. In general, Type I second-degree A-V nodal block is usually well tolerated from a hemodynamic standpoint, and it seldom if ever merits pacemaker therapy on symptomatic grounds. Prophylactic therapy is discussed later in this chapter.
Two-to-one block in the A-V node (Fig. 4-11) is less common than Mobitz I block. It is often associated with organic disease of the A-V node (e.g., Lyme disease), myocardial infarction, s/p mitral valve or other cardiac (particularly congenital heart) surgery, or drug toxicity. Since the impaired function of the A-V node is present, the A-H, and hence, P-R, of the conducted beats is almost always prolonged. Two-to-one block with P-R ≤160 msec should suggest an intra- or infra-His site of block. Improvement of conduction by atropine, beta agonists, or exercise suggests an A-V nodal site of block. However, as stated above, if structure disease of the A-V node is present, improvement of A-V node conduction under these conditions may be small or inapparent.
Third-degree (complete) heart block occurring in the A-V node is relatively common. Most cases of congenital complete heart block are localized to the A-V node (Fig. 4-12),16 as are most cases of transient complete heart block seen in acute inferior wall myocardial infarction.17 This is also the site of block in digitalis intoxication or when block is produced by beta blockers and/or calcium blockers. Intracardiac
recordings reveal atrial deflections that are dissociated from the ventricular rhythm caused by a subsidiary pacemaker. By definition, the atrial deflection is not followed by a His bundle deflection, but the escape ventricular deflection may or may not be preceded by one. Most often, the escape rhythm originates in the His bundle, which is characterized by a narrow (“normal”) QRS complex preceded by a His bundle deflection with a normal H-V interval (Fig. 4-12). It should be emphasized that 20% to 50% of adults with chronic complete block in the A-V node have wide complexes.18,19 Because in the presence of wide QRS complexes, many physicians assume block below His and implant pacemakers in asymptomatic people, such a finding, particularly in asymptomatic or minimally symptomatic patients, may be an indication for electrophysiologic study since A-V nodal block may be reversible.
recordings reveal atrial deflections that are dissociated from the ventricular rhythm caused by a subsidiary pacemaker. By definition, the atrial deflection is not followed by a His bundle deflection, but the escape ventricular deflection may or may not be preceded by one. Most often, the escape rhythm originates in the His bundle, which is characterized by a narrow (“normal”) QRS complex preceded by a His bundle deflection with a normal H-V interval (Fig. 4-12). It should be emphasized that 20% to 50% of adults with chronic complete block in the A-V node have wide complexes.18,19 Because in the presence of wide QRS complexes, many physicians assume block below His and implant pacemakers in asymptomatic people, such a finding, particularly in asymptomatic or minimally symptomatic patients, may be an indication for electrophysiologic study since A-V nodal block may be reversible.
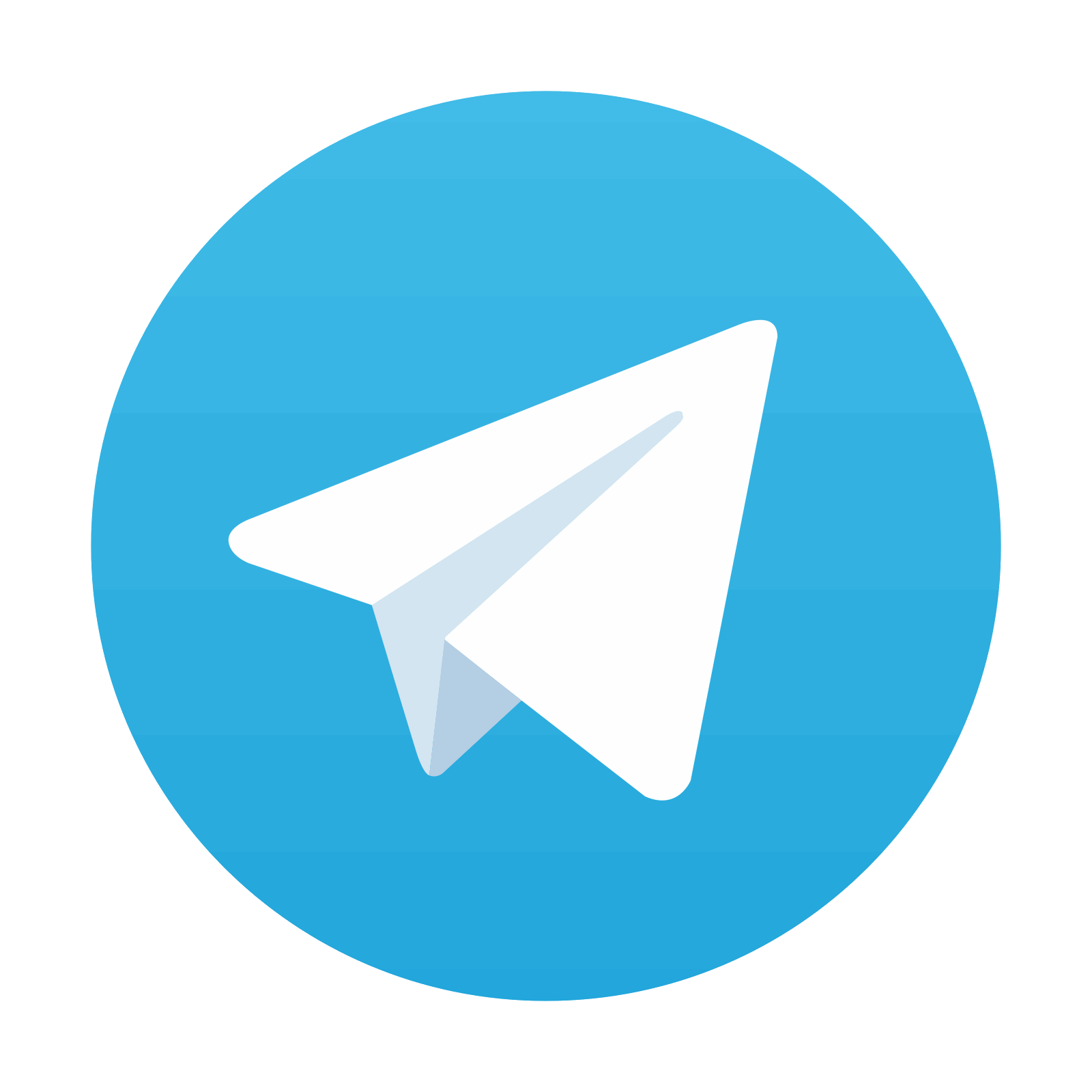
Stay updated, free articles. Join our Telegram channel

Full access? Get Clinical Tree
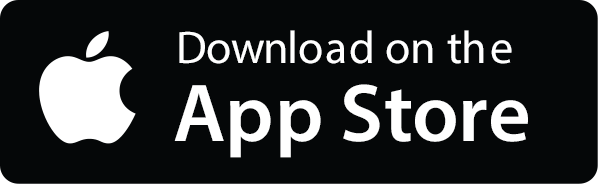
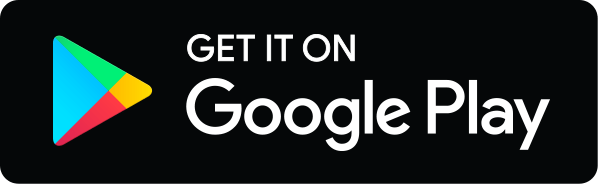