Endurance athletes have an increased risk of developing atrial fibrillation (AF) at 40 to 50 years of age. Signal-averaged P-wave analysis has been used for identifying patients at risk for AF. We evaluated the impact of lifetime training hours on signal-averaged P-wave duration and modifying factors. Nonelite men athletes scheduled to participate in the 2010 Grand Prix of Bern, a 10-mile race, were invited. Four hundred ninety-two marathon and nonmarathon runners applied for participation, 70 were randomly selected, and 60 entered the final analysis. Subjects were stratified according to their lifetime training hours (average endurance and strength training hours per week × 52 × training years) in low (<1,500 hours), medium (1,500 to 4,500 hours), and high (>4,500 hours) training groups. Mean age was 42 ± 7 years. From low to high training groups signal-averaged P-wave duration increased from 131 ± 6 to 142 ± 13 ms (p = 0.026), and left atrial volume increased from 24.8 ± 4.6 to 33.1 ± 6.2 ml/m 2 (p = 0.001). Parasympathetic tone expressed as root of the mean squared differences of successive normal-to-normal intervals increased from 34 ± 13 to 47 ± 16 ms (p = 0.002), and premature atrial contractions increased from 6.1 ± 7.4 to 10.8 ± 7.7 per 24 hours (p = 0.026). Left ventricular mass increased from 100.7 ± 9.0 to 117.1 ± 18.2 g/m 2 (p = 0.002). Left ventricular systolic and diastolic function and blood pressure at rest were normal in all athletes and showed no differences among training groups. Four athletes (6.7%) had a history of paroxysmal AF, as did 1 athlete in the medium training group and 3 athletes in the high training group (p = 0.252). In conclusion, in nonelite men athletes lifetime training hours are associated with prolongation of signal-averaged P-wave duration and an increase in left atrial volume. The altered left atrial substrate may facilitate occurrence of AF. Increased vagal tone and atrial ectopy may serve as modifying and triggering factors.
There is growing evidence that endurance athletes have an increased risk of developing atrial fibrillation (AF) at 40 to 50 years of age. In a retrospective cohort study, the annual incidence rates of lone AF in marathon runners and sedentary men were 0.43/100 and 0.11/100, respectively. Endurance sport practice was associated with an 8.8-fold increased risk of incident AF. Arrhythmia occurred particularly in athletes with >1,500 lifetime hours of sport. Most athletes showed paroxysmal or persistent AF but some progressed to permanent AF during follow-up. Endurance athletes were susceptible to vagally mediated AF, and an increased left atrial volume conferred a higher risk of AF. We recently showed that training years and increased vagal tone were associated with left atrial remodeling in professional soccer players. In an animal model, exercise training induced fibrosis and structural remodeling of the atria and increased AF susceptibility. This was the first study suggesting that exercise-induced atrial remodeling may not necessarily be a benign adaptation to exercise conditioning as previously assumed. Signal-averaged P-wave analysis can detect intra-atrial conduction delay and has been used in patients with and without structural heart disease to predict risk of AF. We hypothesized that in nonelite athletes the accumulated lifetime training hours have an impact on signal-averaged P-wave duration, left atrial volume, and modifying factors such as increased vagal tone that facilitate occurrence of AF.
Methods
The Grand Prix of Bern is 1 of the most popular 10-mile races in Switzerland with >25,000 participants. Nonelite athletes were recruited by an open invitation letter published on the 2010 events homepage. All athletes applied by e-mail and provided age, endurance training years, and average endurance and strength training hours per week. Calculation of average training hours was based on athletes’ estimation and/or exercise diary. Measurement of training years started in adulthood (≥18 years). Lifetime training hours were calculated using the formula average endurance and strength training hours per week × 52 × training years. We included men runners ≥30 years of age with and without previous marathon participation. We excluded subjects with a history of hypertension (blood pressure >140/90 mm Hg) and other known cardiovascular diseases except episodes of paroxysmal AF. Paroxysmal AF was defined as any documented episode of AF of ≥30-second duration with spontaneous conversion to sinus rhythm. Study participants were randomly selected and stratified into 3 groups according to their lifetime training hours. Group thresholds were determined after subject selection to ensure equal group sizes: low training group (<1,500 hours), medium training group (1,500 to 4,500 hours), and high training group (>4,500 hours). Baseline examination consisted of a comprehensive questionnaire to ascertain personal and sports histories, electrocardiography, signal-averaging of the P wave, echocardiography, cardiopulmonary exercise testing on a treadmill, 24-hour ambulatory Holter monitoring, and analysis of heart rate variability. Determination of heart rate at rest and measurement of blood pressure were performed in a quiet room after 5 minutes in a supine position. Runners were examined 12 to 2 weeks (mean 6 ± 3) before the race. Two experienced cardiologists blinded to athletes’ performance performed all analyses. All athletes provided written informed consent and the protocol was approved by the local ethics committee.
Twelve-lead electrocardiograms (ECGs) were recorded with the subject in a supine position and recorded at a paper speed of 25 mm/s (MAC5500, GE Healthcare, Glattbrugg, Switzerland). The methods for recording and analyzing a signal-averaged P wave has been described previously. In brief, a signal-averaged P wave was recorded in a room free from electrical interference. It incorporated 3 bipolar orthogonal leads referred to as the x, y, and z leads, which correspond to those used for acquisition of standard signal-averaged ECG. A P-wave template was generated and confirmed by the user. Then 250 P waves that meet the criteria of matching (95%) with the template P wave were averaged to form a final template. Averaged P-wave signals were digitized and filtered using a spectral filter with a bandwidth of 40 to 250 Hz and then combined into a vector magnitude ([x 2 + y 2 + z 2 ] 1/2 ). Measurements computed by the system included filtered P-wave duration in milliseconds and root mean square voltage in the terminal 20 ms of the P wave expressed in microvolts. In addition, the integral of the P wave (area under the vector magnitude curve from P-wave onset to offset) was computed. Onset and offset of the P wave were manually adjusted.
Standard transthoracic echocardiography was performed (S5-1 2.5-MHz transducer, iE33, Phillips Healthcare, Zurich, Switzerland) according to recommendations of the European Association of Echocardiography. Images were stored digitally and analyzed offline. Left atrial and left ventricular (LV) end-diastolic and end-systolic volumes were calculated according to current recommendations and indexed for body surface area. LV ejection fraction was derived from end-diastolic and end-systolic volumes. Pulse-wave Doppler was performed in the apical 4-chamber view to obtain peak early filling (E-wave) and late diastolic filling (A-wave) velocities, peak early filling/late diastolic filling velocity ratio, deceleration time of early filling wave, and isovolumic relaxation time. Pulse-wave tissue Doppler imaging was performed in the apical 4-chamber view to acquire peak septal and mitral annular velocities.
Spiroergometric testing was performed on a treadmill according to recommendations of the American Heart Association. We used a ramp protocol starting at 7.2 km/hour with speed increasing 0.2 km/hour every 20 seconds until exhaustion. Athletes were encouraged to reach a respiratory exchange ratio of ≥1.05. Respiratory parameters were measured continuously in an open spirometric system (CS 200, Schiller-Reomed AG, Dietikon, Switzerland) and registered as averaged values over 30 seconds. The first (aerobic) and second (anaerobic) lactate thresholds were determined according to current recommendations. Blood pressure was measured at rest and peak exercise.
An ambulatory ECG was recorded over a period of 24 hours according to recommendations of the American Heart Association. Three-channel ECGs were recorded with a Lifecard CF digital recorder (Spacelabs Healthcare, Nuremberg, Germany) and manually analyzed and interpreted using Pathfinder software (Spacelabs Healthcare). Premature atrial contraction (PAC) and premature ventricular contraction were classified according to onset and QRS structure and function. For analysis of heart rate variability the program eliminated 1 RR interval before and 2 intervals after each nonsinus beat. Four standard 24-hour time domain measurements were used: SD of normal-to-normal intervals, heart rate variability triangular index, SD of average normal-to-normal intervals, and square root of mean squared differences of successive normal-to-normal intervals (RMSSD). RMSSD is an accepted measurement of vagal activity in athletes.
Data were analyzed with SPSS 17.0 for Windows (SPSS, Inc., Chicago, Illinois). Normality of quantitative variables was analyzed with Kolmogorov–Smirnov test. The 3 training groups were compared by analysis of variance or Kruskal–Wallis test as appropriate. Categorical data were analyzed using chi-square test. For correlations of signal-averaged P-wave duration, left atrial volume, and LV mass, Pearson correlation coefficient was calculated. A 2-sided p value <0.05 was considered to indicate statistical significance.
Results
Four hundred ninety-two men applied for participation and 70 were randomly selected. Ten runners had to be excluded (8 could not participate in the race because of muscular problems, 1 had mitral valve prolapse, and 1 had an undiagnosed arterial hypertension with diastolic dysfunction). Sixty runners entered the final analysis. Mean age was 42 ± 7 years. Thirty-eight runners (63%) participated in marathon events (1 to 30, mean 8 ± 7). Main type of exercise training was aerobic endurance exercise (88 ± 16%). Four athletes (6.7%) had a history of an ECG-documented episode of paroxysmal AF. One athlete was in the medium training group and 3 athletes were in the high training group (p = 0.252).
With more lifetime training hours athletes were nonsignificantly older, participated more often in marathon competitions, and had significant better 10-mile race times ( Table 1 ). Athletes in the high training group had a significantly longer duration of signal-averaged P-wave, showed more PACs, and had greater parasympathetic tone expressed by RMSSD ( Figure 1 , Table 2 ). PACs were significantly associated with vagal tone (r = 0.446, p = 0.001). Left atrial volume increased significantly from the low to the high training group ( Figure 1 ). An enlarged left atrium (atrial volume >29.0 ml/m 2 ) was present in 24% of runners in the low training group, 40% of runners in the medium training group, and 83% of runners in the high training group (p = 0.001). LV mass increased significantly with training hours, whereas LV volume and LV systolic and diastolic functions showed no significant differences among groups ( Table 3 ). LV hypertrophy (LV mass >115 g/m 2 ) was present in 23% of runners in the medium training group and 59% of runners in the high training group (p <0.001). Diastolic function and blood pressure at rest showed no differences between athletes with and without LV hypertrophy. Signal-averaged P-wave duration showed a weak correlation with LV mass but no correlation with left atrial volume ( Figure 2 ). Blood pressure at rest was normal in all athletes and blood pressure at rest and peak exercise showed no significant differences among groups. Oxygen uptake at aerobic and anaerobic lactate thresholds and at peak exercise increased significantly from the low to the high training group ( Table 1 ).
Variable | Lifetime Training Hours | p Value | ||
---|---|---|---|---|
<1,500 | 1,500–4,500 | >4,500 | ||
(n = 17) | (n = 21) | (n = 22) | ||
Age (years) | 39 ± 4 | 42 ± 7 | 44 ± 9 | 0.132 |
Body mass index (kg/m 2 ) | 23.8 ± 2.4 | 23.8 ± 1.5 | 22.7 ± 1.5 | 0.079 |
Body surface area (m 2 ) | 1.92 ± 0.13 | 1.96 ± 0.14 | 1.92 ± 0.11 | 0.429 |
Marathon participation (n) | 0.8 ± 2.4 | 2.9 ± 2.9 | 10.6 ± 9.5 | <0.001 |
10-mile participation (n) | 1.6 ± 2.0 | 6.6 ± 6.1 | 10.5 ± 10.8 | 0.002 |
Endurance training (years) | 5.3 ± 3.5 | 14.1 ± 6.7 | 22.1 ± 7.4 | <0.001 |
Endurance training (hours/week) | 2.7 ± 1.8 | 4.1 ± 2.2 | 7.9 ± 4.1 | <0.001 |
Strength training (hours/week) | 0.5 ± 0.5 | 0.5 ± 0.6 | 1.0 ± 1.0 | 0.075 |
Lifetime training (hours) | 723 ± 469 | 2,864 ± 648 | 9,209 ± 5,209 | <0.001 |
10-mile race time (mm:ss) | 85:24 ± 11:47 | 81:47 ± 10:12 | 73:12 ± 9:01 | 0.002 |
Cardiopulmonary exercise testing | ||||
Systolic blood pressure at rest (mm Hg) | 126 ± 7 | 122 ± 8 | 121 ± 11 | 0.184 |
Peak systolic blood pressure (mm Hg) | 184 ± 19 | 180 ± 14 | 176 ± 12 | 0.323 |
Peak heart rate (beats/min) | 181 ± 6 | 180 ± 10 | 179 ± 11 | 0.787 |
Oxygen consumption at aerobic threshold (ml/min/kg) | 25.8 ± 3.2 | 26.4 ± 5.7 | 29.8 ± 4.8 | 0.020 |
Oxygen consumption at anaerobic threshold (ml/min/kg) | 42.0 ± 7.1 | 44.0 ± 5.7 | 49.5 ± 7.1 | 0.002 |
Peak oxygen consumption (ml/min/kg) | 51.1 ± 6.6 | 51.4 ± 6.3 | 56.6 ± 6.1 | 0.011 |
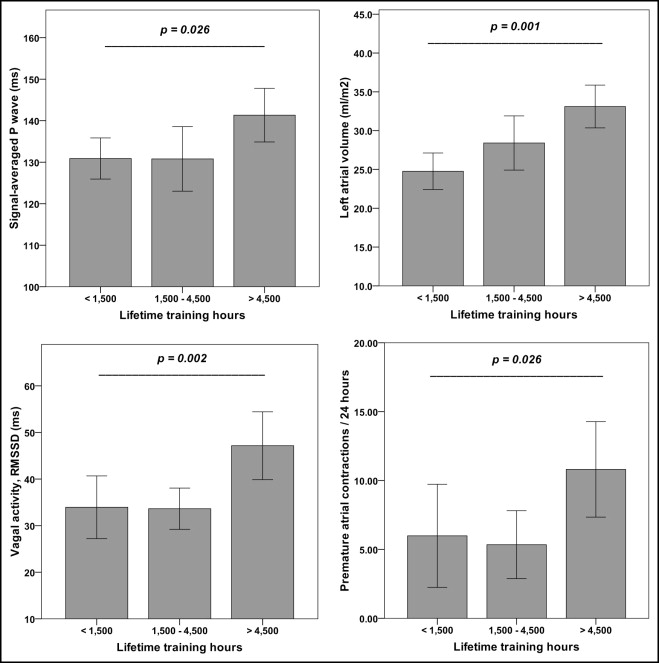
Variable | Lifetime Training Hours | p Value | ||
---|---|---|---|---|
<1,500 | 1,500–4,500 | >4,500 | ||
(n = 17) | (n = 21) | (n = 22) | ||
Electrocardiogram and signal-averaged electrocardiogram | ||||
Heart rate at rest (beats/min) | 61 ± 9 | 55 ± 7 | 50 ± 8 | 0.001 |
Signal-averaged P wave (ms) | 131 ± 6 | 131 ± 13 | 142 ± 13 | 0.026 |
Root mean squares voltage in terminal 20 ms of P wave (μV) | 5.0 ± 1.7 | 4.5 ± 1.8 | 3.8 ± 2.6 | 0.396 |
PQ interval (ms) | 159 ± 19 | 164 ± 15 | 167 ± 16 | 0.288 |
QRS duration (ms) | 100 ± 9 | 100 ± 9 | 99 ± 10 | 0.890 |
Sokolov index (mV) | 2.9 ± 0.8 | 2.9 ± 1.0 | 3.2 ± 1.1 | 0.623 |
T-wave amplitude (μV) ⁎ | 764 ± 209 | 867 ± 340 | 966 ± 263 | 0.090 |
Corrected QT interval | 411 ± 20 | 410 ± 24 | 396 ± 24 | 0.074 |
Analysis of heart rate variability | ||||
SD of normal-to-normal intervals (SDNN, ms) | 199 ± 50 | 202 ± 35 | 229 ± 42 | 0.060 |
Heart rate variability triangular index | 54 ± 15 | 57 ± 10 | 62 ± 15 | 0.177 |
SD of average normal-to-normal intervals (SDANN, ms) | 177 ± 44 | 177 ± 33 | 199 ± 41 | 0.151 |
Square root of mean squared differences of successive normal-to-normal intervals (RMSSD, ms) | 34 ± 13 | 34 ± 9 | 47 ± 16 | 0.002 |
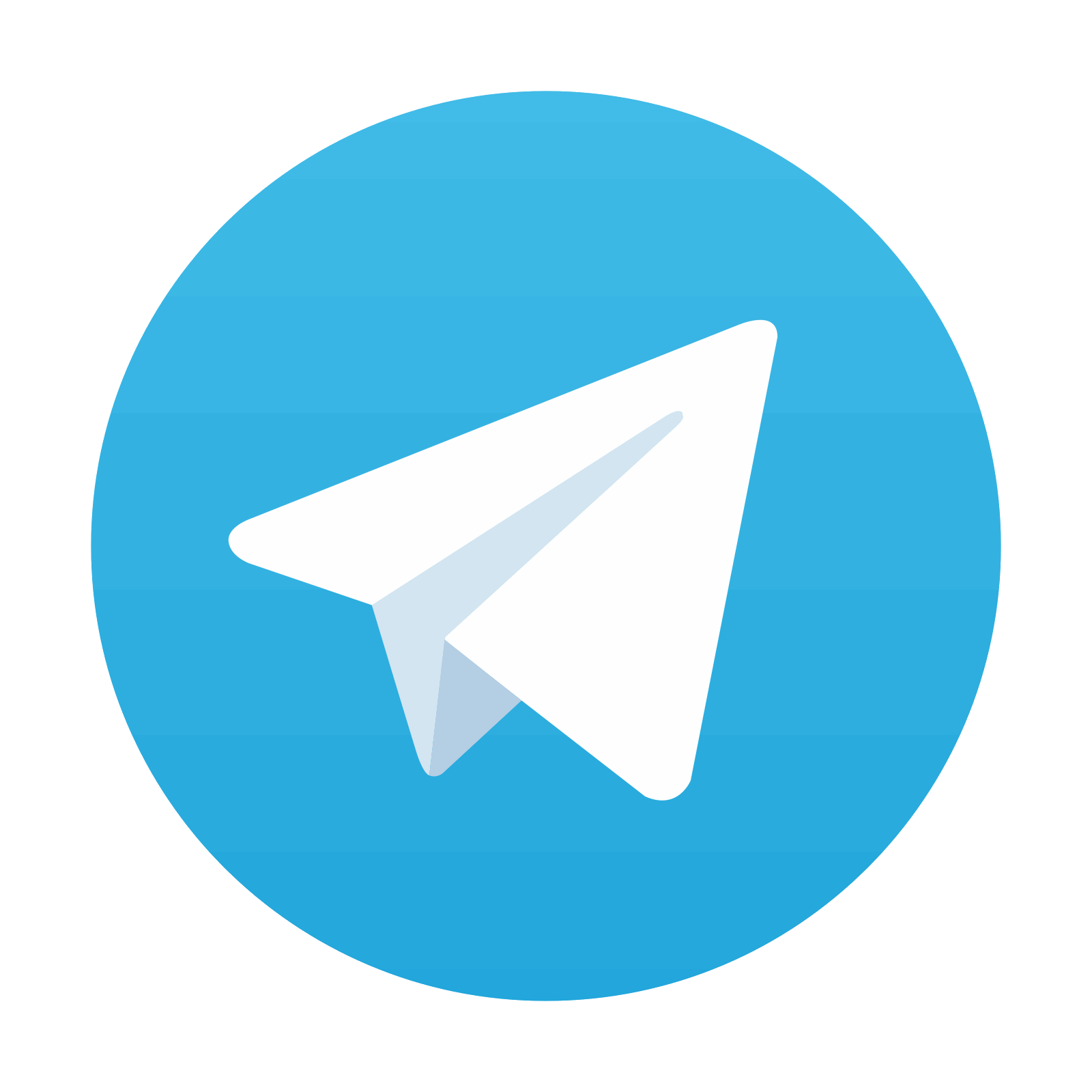
Stay updated, free articles. Join our Telegram channel

Full access? Get Clinical Tree
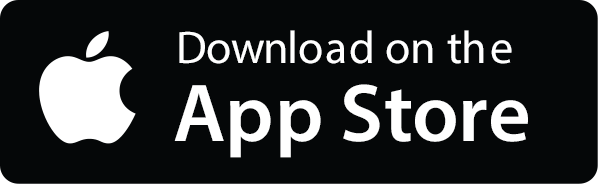
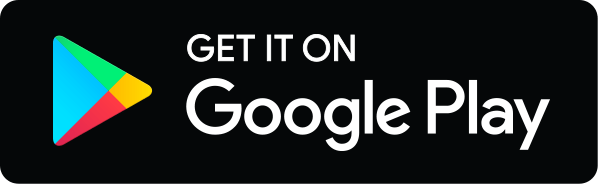
