Chronotropic incompetence (CI) is common in heart failure with preserved ejection fraction (HFpEF) and may be a key reason underlying exercise intolerance in these patients. However, the determinants of CI in HFpEF are unknown. We prospectively studied 157 patients with consecutive HFpEF who underwent cardiopulmonary exercise testing and defined CI according to specific thresholds of the percent heart rate reserve (%HRR). CI was diagnosed as present if %HRR <80 if not taking a β blocker and <62 if taking β blockers. Participants who achieved inadequate exercise effort (respiratory exchange ratio ≤1.05) on cardiopulmonary exercise testing were excluded. Multivariable-adjusted logistic regression was used to determine the factors associated with CI. Of the 157 participants, 108 (69%) achieved a respiratory exchange ratio >1.05 and were included in the final analysis. Of these 108 participants, 70% were women, 62% were taking β blockers, and 38% had chronic kidney disease. Most patients with HFpEF met criteria for CI (81 of 108; 75%). Lower estimated glomerular filtration rate (GFR), higher B-type natriuretic peptide, and higher pulmonary artery systolic pressure were each associated with CI. A 1-SD decrease in GFR was independently associated with CI after multivariable adjustment (adjusted odds ratio 2.2, 95% confidence interval 1.1 to 4.4, p = 0.02). The association between reduced GFR and CI persisted when considering a variety of measures of chronotropic response. In conclusion, reduced GFR is the major clinical correlate of CI in patients with HFpEF, and further study of the relation between chronic kidney disease and CI may provide insight into the pathophysiology of CI in HFpEF.
Despite a growing appreciation of the presence of chronotropic incompetence (CI) as a pathophysiological abnormality in heart failure with preserved ejection fraction (HFpEF), the overall prevalence and clinical factors associated with CI in HFpEF remain unclear. An understanding of factors associated with CI in HFpEF may enhance our understanding of the pathophysiology of CI in HFpEF and may allow clinicians to identify at-risk patients who may benefit from exercise testing to evaluate for CI and who may potentially benefit from rate adaptive pacing to alleviate CI. We therefore evaluated the prevalence and clinical correlates of CI in a well-characterized HFpEF cohort.
Methods
Consecutive patients willing to undergo cardiopulmonary exercise testing (CPET) were prospectively recruited from the outpatient clinic of the Northwestern University HFpEF Program from March 2008 to January 2011 as part of a systematic observational study of HFpEF ( ClinicalTrials.gov identifier #NCT01030991). Patients were initially identified by an automated daily query of the inpatient electronic medical record at Northwestern Memorial Hospital using the following search criteria: (1) diagnosis of HF or the words “heart failure” in the hospital notes, (2) BNP >100 pg/ml, or (3) administration of ≥2 doses of intravenous diuretics. The list of patients generated was screened daily, and only those patients who had left ventricular ejection fraction (LVEF) >50% and who met Framingham criteria for HF were offered post-discharge follow-up in a specialized HFpEF outpatient program. Once evaluated as an outpatient in the HFpEF clinic, a cardiologist who specializes in HF confirmed the diagnosis of HF. The diagnosis of HFpEF was based on previously published criteria that requires an LVEF >50%. In line with the previous studies in HFpEF, patients with hemodynamically significant valvular disease (defined as greater than moderate in severity), previous cardiac transplantation, history of overt LV systolic dysfunction (i.e., previous LVEF <40%), or a diagnosis of constrictive pericarditis were not recruited into the study.
In the Northwestern HFpEF Program, all study procedures (including laboratory testing, electrocardiography, echocardiography, invasive hemodynamic testing, and CPET) are performed in the outpatient setting. All study participants gave written informed consent, and the institutional review board at Northwestern University approved the study.
We collected the following data on all study participants: demographics, New York Heart Association (NYHA) functional class, co-morbidities, medications, vital signs, and body mass index and information from laboratory blood work, echocardiography, CPET, and invasive hemodynamics (when available). Estimated glomerular filtration rate (GFR) was calculated using the Modified Diet in Renal Disease equation. Chronic kidney disease (CKD) was defined as GFR <60 ml/min/1.73 m 2 . All patients underwent comprehensive echocardiography, as described previously.
Symptom-limited CPET using a 10-W bicycle protocol was performed in all patients. CPET was performed in the outpatient setting after the patient was confirmed to be stabilized with no significant cardiac medication changes within the previous 4 weeks. Respiratory gases were analyzed using a calibrated metabolic cart (Vmax, Sensormedics, San Diego, California). Breath-by-breath respiratory gas analysis for measurement of inspired oxygen and expired carbon dioxide was obtained online and averaged every 20 seconds at rest, throughout exercise and during the recovery period. The peak VO 2 value was defined as the highest VO 2 value achieved at end exercise after reaching anaerobic threshold. The VO 2 at anaerobic threshold was determined as the point at which carbon dioxide increases in a nonlinear fashion relative to the rate of oxygen consumption by the V-slope method and confirmed from the nadir of the ventilatory equivalent for VO 2 . The VO 2 at anaerobic threshold for each patient was visually noted and agreed on by 2 independent cardiopulmonary specialists. Standard 12-lead electrocardiograms were obtained at rest, each minute during exercise and for at least 5 minutes during the recovery phase; blood pressure was measured at rest and at each stage of exercise. CPET variables were measured and calculated based on current guidelines.
CPET results were used to establish the presence of CI, our main outcome variable. We defined CI based on thresholds of percent heart rate reserve (%HRR), which was in turn calculated as %HRR = (peak observed HR – HR at rest)/(age-predicted maximal HR – HR at rest). Age-predicted maximal HR was estimated using the Astrand formula, 220 − age. Several studies use the Astrand formula in developing CI definitions that account for β-blocker use, which may lead to pharmacologically induced CI irrespective of underlying physiology. We adopted the %HRR cutoffs of those studies in defining CI: %HRR <80 for those not taking β blockers and %HRR <62 for those taking β blockers.
There is some controversy surrounding the appropriate definition of age-predicted maximum HR. For instance, Tanaka et al recommend the following formula to estimate: 208 – 0.7 × age. We therefore recalculated %HRR and re-established a diagnosis of CI using the Tanaka formula as part of our sensitivity analyses.
For all patients with adequate exercise effort, we obtained the average HR over 20 seconds intervals for up to 140 seconds after peak exercise. HR recovery at 1 and 2 minutes was defined as the difference in HR at peak exercise and HR at 1 and 2 minutes after peak exercise, respectively. We used the HR difference at 1 minute to calculate a binary indicator of abnormal HR recovery as defined by Watanabe et al, based on their finding that HR recovery ≤18 beats is associated with increased mortality.
Participants were stratified by CI status, and clinical, laboratory, echocardiographic, and CPET parameters were compared between groups using the Fisher exact and student’s t tests for dichotomous and continuous variables, respectively. False discovery rate methods were used to account for multiple comparisons. Given the number of statistical tests performed in our study, a p value <0.003 was considered statistically significant (corresponded to a q value <0.05).
A series of multivariable models were generated to examine the association between GFR and CI. Variables associated with CI status on univariate analysis were included as covariates in our multivariable models of chronotropic response. We additionally included smoking status and β-blocker use (because they have been previously shown to impair chronotropic response) and LV mass index and loop diuretic use (as markers of cardiac and renal disease severity) as covariates in our multivariable models. We conducted multivariable-adjusted logistic regression to examine the association of GFR with CI, and we repeated these analyses using linear regression to examine the association between GFR and %HRR. For the statistical models, we calculated goodness-of-fit measures (Akaike information criterion and the adjusted Pearson coefficient [R 2 ]). Models were similarly used to evaluate correlates of HR recovery. The mean ΔHR relative to peak VO 2 was estimated from a linear regression model of ΔHR on peak VO 2 for those with and without CI. All analyses were performed using Stata, v. 12 (StataCorp, College Station, Texas).
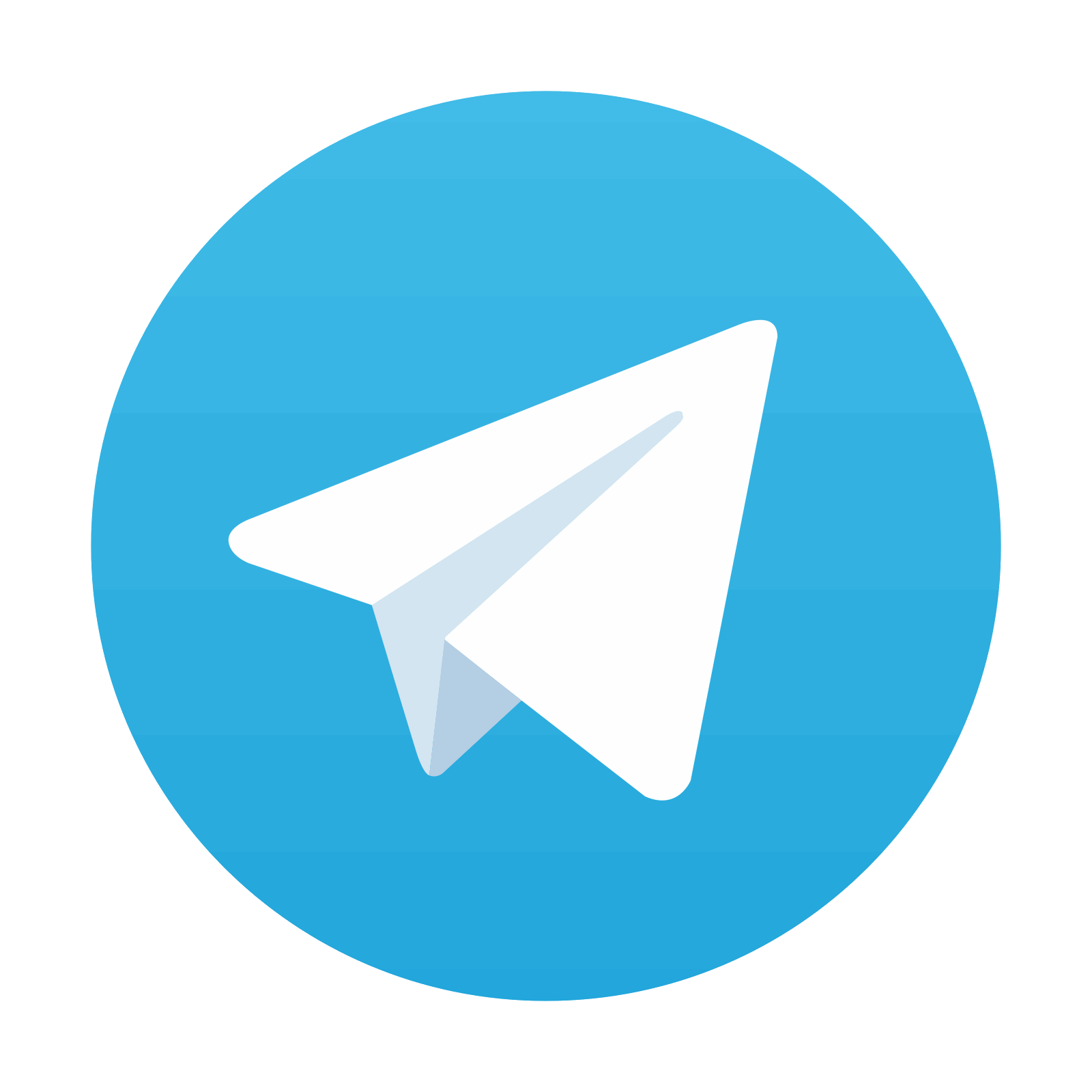
Stay updated, free articles. Join our Telegram channel

Full access? Get Clinical Tree
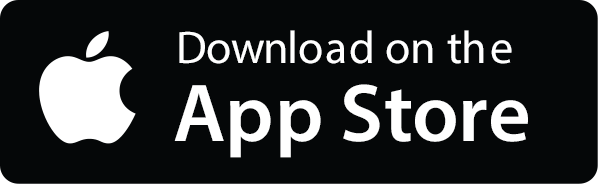
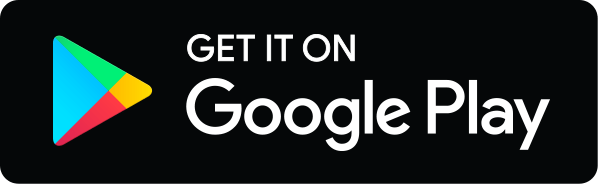