This chapter is intended to provide a clinically oriented discussion of proximal aortic disease emphasizing not only the more common problems seen in clinical practice such as ascending aneurysms and dissection but also to serve as an initial reference for many less common conditions that are generally encountered only occasionally or in specialized circumstances. A discussion of congenital aortic disease is included since an ever-increasing number of patients are now surviving into adulthood and will be encountered by vascular specialists.
The primordial heart and the vascular system appear in the middle of the third week of embryonic development. As the pharyngeal arches form during the fourth and fifth weeks of development, they are supplied by arteries—the aortic arches—that arise from the aortic sac and terminate in the dorsal aortae. Initially, the paired dorsal aortae run through the entire length of the embryo, but they soon fuse to form a single dorsal aorta, just caudal to the pharyngeal arches (Figure 26-1). When the primordial ventricle contracts, blood is pumped through the bulbus cordis and truncus arteriosus into the aortic sac, from which it is distributed to the aortic arches in the pharyngeal arches. The blood then passes into the dorsal aortae for distribution to the embryo.
During the fifth week of development, active proliferation of mesenchymal cells in the walls of the bulbus cordis results in the formation of bulbar ridges, similar ridges form in the truncus arteriosus. The bulbar and truncal ridges undergo a 180-degree spiraling. The spiral orientation of the bulbar and truncal ridges results in the formation of a spiral aorticopulmonary septum when the ridges fuse. This septum divides the bulbus cordis and truncus arteriosus into two arterial channels, the aorta and pulmonary trunk (Figure 26-2). The final transformation of the truncus arteriosus, aortic sac, aortic arches, and dorsal aorta into the adult arterial pattern are shown in Figure 26-3.1
FIGURE 26-2.
Partitioning of the bulbus cordis and truncus arteriosus. (A) Ventral aspect of the heart at 5 weeks. (B) Transverse sections of the truncus arteriosus and bulbus cordis, illustrating the truncal and bulbar ridges. (C) The ventral wall of the heart and truncus arteriosus have been removed to demonstrate these ridges. (D) Ventral aspect of the heart after partitioning of the truncus arteriosus. (E) Sections through the newly formed aorta (A) and pulmonary trunk (PT) show the aorticopulmonary septum. (F) Six weeks. The ventral wall of the heart and pulmonary trunk have been removed to show the aorticopulmonary septum. (G) Diagram illustrating the spiral form of the aorticopulmonary septum. (H) Drawing showing the great arteries twisting around each other as the leave the heart.
Reproduced with permission from Moore K, Persaud TVN. The cardiovascular system. In: Before We Are Born. Essentials of Embryology and Birth Defects, 6th ed. Philadelphia, PA: WB Saunders; 2003:264.

FIGURE 26-3.
Arterial changes that result during transformation of the truncus arteriosus, aortic sac, aortic arches, and dorsal aortae into the adult pattern. The vessels that are not colored are not derived from these structures. (A) Aortic arches at 6 weeks. By this stage, the first two aortic arches have disappeared. (B) Aortic arches at 7 weeks. The parts of the dorsal aortae and aortic arches that normally disappear are indicated in with broken lines. (C) Arterial arrangement at 8 weeks. (D) Arterial vessels of a 6-month-old infant.
Reproduced with permission from Moore K, Persaud TVN. The cardiovascular system. In: Before We Are Born. Essentials of Embryology and Birth Defects, 6th ed. Philadelphia, PA: WB Saunders; 2003:264.

Congenital heart disease (CHD) occurs in 0.4% to 0.8% of live births. Excluding bicuspid aortic valve (BAV) and aortic valve stenosis, conotruncal abnormalities represent only 10% to 15% of congenital heart lesions. The incidence of CHD does not seem to vary by race.2
Some environmental exposures, maternal illnesses, or exposure to drugs can increase the likelihood of developing CHD; infants of diabetic mothers are at increased risk of developing CHD in general and conotruncal defects such as truncus arteriosus in particular. Exposure to retinoic acids is also associated with conotruncal defects. Although CHD is often considered a sporadic event, some cases result from inherited genetic traits. There is an increased recurrence risk of CHD when either a parent or a sibling is affected.2,3
Truncus arteriosus (TA) or persistent TA is a rare anomaly, comprising between 1% and 4% of all cases of congenital heart disease. TA results from failure of truncal ridges and aorticopulmonary septum to develop normally and to divide the truncus arteriosus into the aorta and pulmonary trunk. The exact cause of this condition is unknown, but up to 40% of the patients have microdeletions in the chromosome band 22q11. In this anomaly, a single arterial trunk, the TA, arises from the heart by way of a semilunar valve and supplies the systemic, pulmonary, and coronary circulations. A large perimembranous, infundibular ventricular septal defect (VSD) is present below the TA anomaly. The number of truncal valve cusps varies from two to as many as six.1,4,5,6 Other associated abnormalities include right aortic arch in 30% of patients, interrupted aortic arch (IAA) in 10% of the patients, which almost always occurs between the left common carotid and the left subclavian artery, and evidence of DiGeorge syndrome with hypocalcemia in 33% of the patients.5
This anomaly is divided in four types according to the Collett and Edwards’ classification, which is based on the origin of the pulmonary artery. Type I, a short pulmonary trunk emerges from the left lateral wall of the common arterial trunk and divides into right and left pulmonary branches. In type II, the pulmonary arteries arise either independently or via a common orifice from the posterior wall of the common arterial trunk, this is the most common type of persistent TA, type I and II constitute 85% of cases. In type III, the pulmonary arteries arise from the lateral aspects of the truncus. In type IV, no true pulmonary arteries are present, and the lungs are supplied by arteries arising from either the aortic arch or the descending aorta.5,7 In 1965, Van Praaghs proposed the other commonly cited classification that also includes four types. Type A1 is identical to the type I of Collett and Edwards. Type A2 includes Collett and Edwards type II and most cases of type III, namely, those with separate origin of the branch pulmonary arteries from the left and right lateral aspects of the common trunk. In type A3, only one pulmonary artery (right or left) is present, and one lung is supplied by either a bronchial artery or an aberrant pulmonary artery arising from the aortic arch. Type A4 is defined by the coexistence of an IAA (Figure 26-4).4,5,6
FIGURE 26-4.
Anatomic subtypes of truncus arteriosus (TA), according to the classification systems of both Collett and Edwards (I, II, III) and the Van Praaghs (A1, A2, A3, A4).
Reproduced with permission from Karamlou TB, Shen I, Ungerleider RM. Congenital heart disease. In: Brunicardi FC, ed. Schwartz’s Principles of Surgery. 8th ed. New York, NY: McGraw-Hill; 2005:611.

The main pathophysiologic consequences of truncus arteriosus are the mandatory mixing of systemic and pulmonary venous blood at the level of the VSD and truncal valve, which leads to arterial saturations near 85%, and the presence of a nonrestrictive left-to-right shunt. Additionally, truncal valve stenosis or regurgitation, left ventricular outflow tract obstruction, and stenosis of the pulmonary artery branches can further contribute to both pressure and volume loading of the ventricles. These factors lead to severe heart failure early in life. Increased pulmonary vascular resistance may develop as early as 6 months of age, leading to poor results with surgical repair. Pulmonary blood flow increases in type I, remains normal in types II and III, and decreases in type IV.4,5
The clinical signs of truncus arteriosus vary with age and depend on the level of pulmonary vascular resistance. Patients usually present in the neonatal period with signs and symptoms of congestive heart failure (CHF) secondary to increased pulmonary blood flow and mild to moderate cyanosis, which may be seen immediately after birth. A history of dyspnea with feeding, failure to thrive, and frequent respiratory infections is usually present in infants. The peripheral pulses are bounding with a wide pulse pressure. The second heart sound is loud and single. A harsh pan-systolic murmur may be noted at the left sternal border and suggest VSD, and occasionally a diastolic murmur may be heard in the presence of truncal regurgitation.4,5
Chest radiography shows increased pulmonary flow and cardiomegaly. As mentioned above a right aortic arch may be seen in 30% of cases. The ECG shows normal sinus rhythm with biventricular hypertrophy. Echocardiography is sufficient to confirm the diagnosis and fully characterize the various anatomic features in most patients. Echocardiography shows three diagnostic findings: A large VSD directly under the truncal valve, a large single great artery that arises from the heart and nonvisualization of the pulmonary valve, and only one semilunar (truncal) valve is seen. Cardiac catheterization can be helpful in cases where pulmonary hypertension is suspected or to further delineate coronary artery abnormalities, which can be present in up to 50% of the subjects. Coronary abnormalities include stenotic ostia, a high or low takeoff, and abnormal branching or course.4,5
Without surgery most infants die of CHF within 6 to 12 months. If pulmonary blood flow is restricted by the development of pulmonary vascular disease, the patient may survive into early adulthood. The cause of death in unrepaired patients usually is cardiac arrest or multiple organ failure in the face of systemic perfusion that is inadequate to meet the body’s metabolic demands with progressive metabolic acidosis and myocardial dysfunction.5,8
Truncus arteriosus is an indication for surgery and repair should be done in the neonatal period before the development of pulmonary hypertension and Eisenmenger’s physiology. Surgical repair is done with cardiopulmonary bypass (CPB). Repair is based on modifications of the Rastelli procedure and consists of separation of the pulmonary arteries from the aorta, closure of the aortic defect, and VSD closure. For type I a valved or valveless conduit, preferentially aortic homograft, is placed between the right ventricle and the pulmonary artery in order to reconstruct the right ventricular outflow tract. For types II and III, a circumferential band of the truncus, which contains both pulmonary artery orifices, is removed. This cuff is connected to the right ventricle by a valved or valveless Dacron tube. Aortic continuity is restored with a tubular Dacron graft. Severe truncal valve insufficiency occasionally requires truncal valve replacement, which can be done with a cryopreserved allograft. Severe truncal regurgitation, IAA, and coexistent coronary anomalies are risk factors for perioperative death and poor outcome. Pulmonary artery banding is reserved for patients who have increased pulmonary blood flow and are not deemed suitable candidates for definitive repair.4,5,9,10 Surgical mortality rate is 10% to 30%. For those surviving the initial postoperative period, the survival rate at 10 to 20 years is in excess of 60%, with most deaths resulting from sequelae of delayed repair (pulmonary vascular obstructive disease), reinterventions, or residual/recurrent physiologic abnormalities.9,11,12,13
Follow-up every 4 to 12 months is required to detect late complications. Progressive truncal valve insufficiency may develop, and truncal valve replacement may be needed. A small conduit needs to be changed to a larger size, usually by 2 to 3 years of age. Calcification of the valve in the conduit may occur within 1 to 5 years, which requires reoperation. Ventricular arrhythmias may develop because of right ventriculotomy.5,9,11,13
Aortopulmonary window defect (APW) is a rare congenital lesion that accounts for less than 1% of all congenital heart lesions. APW results from failure of the spiral septum to completely divide the embryonic truncus arteriosus; this is a consequence of failure of the conotruncal ridges to fuse. The spiral septum normally divides the truncus into the aorta and the pulmonary artery. No genetic associations or environmental risk factors are known. The APW consists of a large communication between the ascending aorta and the main pulmonary artery that usually occurs as a single defect that begins above the sinuses of valsalva on the left lateral wall of the aorta (Figure 26-5). The presence of a pulmonary and an aortic valve and an intact ventricular septum distinguishes this anomaly from truncus arteriosus. More than half of the patients have some other associated lesions that include but are not limited to coronary artery anomalies, patent ductus arteriosus (PDA), atrial septal defect (ASD), VSD, IAA, and tetralogy of Fallot.4,8,14,15
The main pathophysiologic event in APW is that of a large left-to-right shunt with increased pulmonary blood flow. Volume overload and increased pulmonary blood flow lead to progressive left ventricular dysfunction and early development of CHF, these patients are particularly susceptible to Eisenmenger syndrome at an early age because of combined systolic and diastolic runoff into the pulmonary circulation. Aortic arch obstruction and IAA are usually associated with APW, and they act as an obstruction to systemic flow and further increase the left-to-right shunt. Perfusion to the lower body is therefore dependent on flow through the ductus arteriosus. Closure of the ductus results in severe hypoperfusion of the lower body, increased pulmonary blood flow, and CHF.
Infants usually present with symptoms of heart failure during early infancy, occasionally minimal cyanosis, frequently respiratory tract infections, tachypnea with feedings, and failure to thrive. Peripheral pulses are bounding. There is usually a systolic murmur with a mid-diastolic component as a result of the increased blood flow across the mitral valve. The second heart sound is loud and does not split.8
The ECG shows left or biventricular hypertrophy, chest radiography demonstrates cardiomegaly, prominence of the pulmonary artery, and increased pulmonary blood flow. Echocardiogram shows enlargement of the left-sided heart chambers and the window defect. Cardiac catheterization reveals a left-to-right shunt at the level of the pulmonary artery, and manipulation of the catheter from the main pulmonary artery directly into the ascending aorta is diagnostic.8 Cardiac catheterization is usually reserved for those patients who present after 6 months of age or to any patient at risk of pulmonary hypertension. If pulmonary resistance is elevated, testing for reversibility with vasodilators should be performed since the presence of irreversible pulmonary hypertension is a contraindication for surgery.
The APW should be surgically corrected during infancy or as soon as it is diagnosed to avoid the development of pulmonary hypertension. Spontaneous closure is not known to occur. Surgery is performed with CPB. The defect is exposed from the anterior aspect of the aorta, the main pulmonary artery, or the APW itself, and the defect in the aorta and pulmonary artery are corrected with a patch; and any other associated anomalies should be repaired at the same time.14,15
The patient will require lifelong echocardiographic follow-up to monitor for the development of aortic or pulmonary artery stenosis. The mortality rate reflects the changes that have occurred in pediatric cardiac surgery over time, with a mortality of 37% in the early series when simple ligation or transection of the defect was performed, to 0% in later years with the approach described above. The risk of mortality depends upon the presence or absence of associated anomalies, being less than 10% and 0% respectively.14,15
The hypoplastic left heart syndrome (HLHS) is defined as a spectrum of cardiac malformations with normally aligned great arteries without a common atrioventricular (AV) junction, characterized by underdevelopment of the left heart with significant hypoplasia of the left ventricle including atresia, stenosis, or hypoplasia of the aortic or mitral valve, or both, and hypoplasia of the ascending aorta and aortic arch.16
HLHS, therefore, is made up of several pathologic cardiac phenotypes. At the severe end of the spectrum are found those patients with aortic and mitral atresia with a virtually nonexistent left ventricle. At the mild end of the spectrum are those patients with hypoplasia of the aortic and mitral valves, but without intrinsic valvar stenosis or atresia, and milder degrees of left ventricular hypoplasia.16
The etiology of the HLHS is unknown. The reported prevalence of HLHS is 0.2 per live births and is more common in males (3:2 to 2:1). Several other associated congenital anomalies have been reported, including anomalous venous return, transposition or malposition of the great arteries, VSD, and AV canal defect.17
Multiple classifications and definitions have been used in the past, creating confusion and a lack of consistency in previous reports. In 2005 an International Pediatric and Congenital Cardiac Code was accepted based on work from the Society of Thoracic Surgeons and the European Association for Cardio-Thoracic Surgery. This is a very extensive and detailed classification that goes beyond the scope of this chapter, and readers are referred to it for further details.16
At birth, the right ventricle provides output to the pulmonary circulation via the ductus arteriosus to the systemic circulation. The pulmonary and aortic pressures are equal in both systole and diastole. Blood returning from the pulmonary veins into the left atrium cannot flow into the left ventricle, and patency of the foramen ovale is imperative. There is mixing of pulmonary and systemic venous return, but the relative contribution of each varies depending on the underlying anatomy. The restrictive nature of the ASD can produce postcapillary pulmonary hypertension, leading not only to pulmonary edema and increased cyanosis, but also a decrease in flow of blood to the lungs, with less volume overload on the right ventricle.18,19
A grayish blue color of the skin is soon apparent after birth and denotes a combination of cyanosis and hypoperfusion. The diagnosis is usually made in the first day of life. Signs of heart failure are usually apparent in the first days of life. The peripheral pulses are weak or absent. Cardiac examination reveals a dominant right ventricular impulse, a single second heart sound, and a nonspecific systolic murmur at the left lateral border. Chest radiography shows cardiomegaly and increase pulmonary blood flow. ECG shows right atrial enlargement and right ventricular hypertrophy. Echocardiography is diagnostic. Cardiac catheterization is not usually necessary.8
If left untreated, the vast majority of children with HLHS will die within 7 to 10 days of birth. Survival for patients with HLHS became a possibility in 1980 when Norwood et al. described a staged palliative surgical approach including aortic arch reconstruction. Shortly thereafter, heart transplantation for infants and small children also became feasible.20 In the preoperative management it is mandatory to maintain ductal patency to avoid systemic and coronary hypoperfusion and to prevent excessive pulmonary blood flow. A relative hypoxemia should be maintained (70%–80%), in order to prevent pulmonary vasodilatation since this would increase pulmonary blood flow and would decrease systemic perfusion.18
Three surgical approaches have evolved over the years: A multistage reconstructive surgery based on a single ventricle physiology popularized by Norwood, cardiac replacement proposed by Bailey, and a biventricular repair as used in patients at the mild end of the spectrum of hypoplastic heart syndrome, as well as for those patients with aortic atresia with a VSD and well-developed left ventricle. The first two approaches can be used in any patient with HLHS, the biventricular approach can be used in very few patients.16
The Norwood operation is defined as an aorticopulmonary connection and neoaortic arch construction resulting in univentricular physiology and pulmonary blood flow controlled with a calibrated systemic-to-pulmonary artery shunt, or a right ventricle to pulmonary artery conduit, or rarely a cavopulmonary connection.16
The principles of the Norwood operation are as follows: First, establishment of a permanent communication between the right ventricle and aorta by transection of the pulmonary trunk and creation of an anastomosis between the pulmonary trunk and the ascending aorta and aortic arch. Second, limitation of blood flow to the lungs to attenuate the development of pulmonary hypertension. This is accomplished by a calibrated systemic-to-pulmonary artery shunt, or a right ventricle to pulmonary artery conduit, or rarely a cavopulmonary connection. Third, creation of a satisfactory interatrial communication by an atrial septotomy in order to ensure unobstructed pulmonary venous return to the tricuspid valve. Fourth, the PDA is ligated. Finally, the adequacy of the coronary arterial perfusions assured. This first stage is performed in the first 2 weeks of life.16,21
In a second surgery performed at 4 to 6 months of age, a form of the hemi-Fontan operation or construction of a bidirectional superior cavopulmonary shunt is done and the systemic-to-pulmonary artery shunt is removed. Finally, in a third operation performed at 12 to 18 months after the second surgery, physiologic correction is achieved by separating the systemic and pulmonary circulations using a Fontan-type procedure. Multiple variations in this technique have been reported, trying to fulfill more reliably the surgical criteria for the first stage of palliation and to prepare the patient to become an optimal candidate for the Fontan operation.16,21
Factors associated with poor outcome include prematurity, low birth weight, chromosomal and other extracardiac abnormalities, additional intracardiac lesions, older age, small aorta, poor preoperative right ventricular function, and obstructed pulmonary venous return.20,22,23
Cardiac transplantation offers the advantage of replacement of an abnormal circulation with a normal 4-chamber heart in a single operation. Disadvantages of this approach are the limited availability of donors and the need for lifelong immunosuppression and risk of rejection.
As mentioned before, the biventricular repair is applicable only in patients at the milder end of the spectrum of the HLHS. These include three groups of patients: Those with hypoplasia of the structures of the left ventricle and aorta without intrinsic valvar stenosis or atresia (the so-called hypoplastic left heart complex), those with critical aortic stenosis with left ventricular hypoplasia, and finally those with aortic atresia with VSD and a well-developed left ventricle. The surgery consists of an extensive enlargement of the aortic arch and ascending aorta with a pulmonary homograft and closure of the atrial and VSDs.16
Chang et al.17 analyzed data of 1986 infants (younger than 30 days) with HLHS in the United States. The overall inhospital mortality was 40.9% (812/1986). Only 407 patients (20.5%) underwent surgery, 346 (17.4%) had the Norwood procedure, and 61 (3.1%) had a heart transplantation. A trend was seen in the increasing percentage of patients treated with the Norwood procedure, from <8% in 1988 to 34% in 1997. The percentage of patients who were treated with heart transplantation remained stable. The overall inhospital mortality for patients who underwent the Norwood procedure was 46%. The inhospital mortality rate for patients with the Norwood procedure decreased from 64% in 1988 to close to 30% in 1997. The overall inhospital mortality rate was significantly lower in the heart transplantation group than in the Norwood procedure group (26.2% vs. 46%). From this data one can appreciate the fact that comfort care is still the most common strategy. Comfort care is the predominant option offered to families of infants with HLHS in other countries such as Japan and the United Kingdom.
Bove et al.22 reported a hospital survival of 93% for 85 patients with standard risks and 53% for 15 patients considered to be at high risk in patients who underwent the Norwood procedure. Similar results have been reported by others.23,24,25,26 The mortality for the second and third stage of the correction is lower, with survival for both procedures greater than 95%.25,27,28 The 5-year survival is approximately 70%.24,25
Transposition of the great arteries (TGA) is the most common cause of cyanotic heart disease in newborn infants; it accounts for approximately 5% of all congenital heart defects and is more common in males than in females as well as in infants of diabetic mothers. There is no race predilection. The hallmark of the TGA is ventriculoarterial disconcordance. In typical cases, the aorta is located anteriorly and to the right of the pulmonary artery and arises from right ventricle carrying desaturated blood to the body, while the pulmonary trunk arises posteriorly from the left ventricle carrying oxygenated blood to the lungs. This configuration is known as d-transposition of the great arteries (D-TGA). Survival in these neonates is provided by an ASD, the ductus arteriosus, and/or a VSD, which permits some mixture of oxygenated and deoxygenated blood. The exact etiology of this anomaly is unknown and is presumed to be multifactorial. This defect is thought to result from the failure of the conus arteriosus to develop normally during incorporation of the bulbus cordis into the ventricles. Defective neural crest cell migration may also be involved.1,5
Other associated anomalies include VSD, coarctation of the aorta (COA), IAA, pulmonary atresia, subaortic or valvular aortic stenosis, coronary anomalies, and overriding of the AV valves.29,30
D-TGA results in parallel pulmonary and systemic circulations, with patient survival depending on intracardiac mixing. There is increased pulmonary blood flow, which causes left atrial enlargement and a left-to-right shunt through the foramen ovale.
Infants with D-TGA and intact ventricular septum are cyanotic at birth. The clinical course and manifestations depend on the extent of intercirculatory mixing and the presence of associated anatomic lesions. Those with a VSD may take longer time to become cyanotic. Signs of congestive heart failure develop during the newborn period. Physical findings depend on the presence of associated lesions and include a single and loud second heart sound. No murmur is heard if the ventricular septum is intact and, if there is a VSD, a systolic murmur is present. ECG shows right ventricular hypertrophy. Biventricular hypertrophy may be present in infants with a large VSD, PDA, or pulmonary vascular obstructive disease. Chest radiography reveals the classic egg-shaped configuration with a narrow superior mediastinum. Echocardiography is diagnostic and usually provides the anatomic and functional information necessary for management of these infants. Cardiac catheterization is rarely required, except in those infants requiring surgery in the neonatal period to assess the suitability of the left ventricle to support the systemic circulation.4,5
The natural progression consists of progressive hypoxia and acidosis resulting in death unless mixing of blood improves. CHF develops in the first week of life. Without surgical repair, death occurs in 30% by the first week, 50% by the end of the first month, and 90% by the end of the first year. Those patients with an intact ventricular septum are the sickest group and develop pulmonary vascular obstructive disease early in life. Patients with a VSD or a large PDA are the least cyanotic group but the most likely ones to develop CHF and pulmonary vascular disease. The presence of VSD and pulmonary stenosis protects from pulmonary vascular disease and allows a longer survival without surgery.5
Before surgical repair certain measures are required. The ductus arteriosus should be kept open or reopened with the infusion of prostaglandin in order to improve arterial oxygenation. Balloon atrial septotomy should be performed in order to improve intracardiac mixing. If an adequate interatrial communication exists, this procedure is not required. In older infants or those for whom the balloon septotomy was only temporarily successful, blade atrial septotomy may be performed.5
Several procedures have been described for the repair of TGA. The procedures can be grouped into those that redistribute right- and left-sided flow at the level of the atrium, the ventricle, and the great arteries.
The Mustard and the Senning operations are performed at the atrial level. In the Mustard operation, the pulmonary and systemic blood return is redirected at the atrial level through a pericardial or prosthetic baffle. In the Senning operation, the blood return from the pulmonary veins is redirected through the tricuspid valve to the systemic right ventricle by means of an atrial flap fashioned from the free wall of the right atrium plus redirection of systemic venous flow from both the superior and inferior vena cava, through the mitral valve to the pulmonary left ventricle by using the intra-atrial septum. These atrial switch procedures result in a physiologic correction, but not an anatomic one, as the systemic circulation is still based on the right ventricle. Long-term complications include atrial conduction disturbances, sick sinus syndrome, supraventricular arrhythmias, right ventricular failure, tricuspid valve insufficiency, sudden death, superior or inferior vena cava syndrome, and pulmonary vascular obstructive disease.4,5,31,32
The Rastelli operation is used in patients with VSD and severe pulmonary stenosis. The redirection of flow is accomplished at the ventricular level. The left ventricle output is directed to the aorta by creating an interventricular tunnel between the VSD and the aortic valve. The pulmonary artery is disconnected from the left ventricle and a conduit is placed between the right ventricle and the pulmonary artery.5
In the arterial switch or Jatene procedure, the great arteries are divided proximally, the coronary arteries are reimplantated in the pulmonary trunk, and the distal great arteries are connected to the proximal end of the other great artery (distal aorta to pulmonary trunk and distal pulmonary artery to proximal aorta). The Damus-Kaye-Stansel operation is used in patients with VSD and subaortic stenosis. In this procedure the pulmonary artery is divided proximal to the bifurcation and anastomosed end to side to the ascending aorta by using a Dacron or Gore-Tex tube, the aortic valve is closed, the VSD is closed, and a conduit is placed between the right ventricle and the distal pulmonary artery. The most common complications is pulmonary artery stenosis at the site of the anastomosis. Other complications include complete heart block and aortic regurgitation or stenosis. Arrhythmias are rare.5,7,33
The surgical approach depends on the age of the patient at presentation and the presence of associated congenital cardiac lesions. The arterial switch is considered the procedure of choice in the majority of the cases, since it is associated with fewer complications and restores the normal physiologic relationships of systemic and pulmonary arterial flow. The Senning operation is reserved for those patients with unfavorable coronary anatomy, for late referral, or for patients with TGA and pulmonary vascular obstructive disease. The timing of surgery depends on the presence and size of a PFO, ASD, VSD, PDA, pulmonary stenosis, and aortic stenosis. The arterial switch is performed in the first 2 weeks of life before the left ventricular mass decreases to the point at which it would not be able to generate adequate pressure to perfuse the systemic circulation.
The inhospital mortality for the arterial switch is less than 8%, with a 5-year and 15-year survival greater than 80%.29,30,32,33,34,35 Factors associated with increased mortality include unfavorable coronary anatomy, the presence of a VSD, arch pathology, and delayed sternal closure.29,30,35 The inhospital mortality for the Senning procedure is usually less than 15%.31
L-TGA or congenitally corrected TGA occurs in less than 1% of all patients with congenital heart disease. In this case, the right atrium is to the right of the left atrium. The right atrium empties into the anatomic left ventricle through the mitral valve, and the left atrium empties in the anatomic right ventricle through the tricuspid valve. There is an inversion of the ventricular chambers with their corresponding AV valves. The aorta is located to the left of the pulmonary artery. Oxygenated blood returns into the left atrium, passes to the anatomic right ventricle, and is then ejected into the aorta.5
Theoretically, no functional abnormality exists, but in most cases there are associated intracardiac defects, AV conduction disturbances, and arrhythmias. VSD is present in 80% of the cases. Pulmonary stenosis is present in 50% of patients and is usually associated with VSD. Dextrocardia is present in nearly 50% of subjects and there is usually inversion of the coronary arteries. Patients are usually asymptomatic when there are no associated defects. Most patients with associated defects become symptomatic in the first months of life. Medical and surgical management is dictated by the associated anomalies.5
IAA is an uncommon anomaly, accounting for less than 1% of all congenital heart lesions. There is no gender or race predilection. IAA is defined as an absence of luminal continuity between the ascending and descending aorta, representing an extreme form of COA in which the aortic arch is atretic or a segment of the aortic arch is absent.
In the majority of cases there is a concomitant VSD or PDA. Other associated anomalies include ASD, truncus arteriosus, APW, TGA, HLHS, double-outlet right ventricle, AV canal defect, BAV, mitral valve anomalies, and subaortic stenosis. Up to 50% of patients have associated DiGeorge syndrome.36,37,38,39
Approximately half of patients with IAA have a deletion in the chromosome band 22q11. The T-box gene TBX1 appears to be responsible for most aspects of the DiGeorge phenotype. IAA type A seems to have a different etiology from IAA type B. Three-fourths of patients with IAA type B have the deletion, while few patients with IAA type A have the deletion.40,41
IAA is classified into three types based on the location of the interruption (Figure 26-6). In type A, the interruption occurs distal to the left subclavian artery (20%–30% of the cases). In type B, the interruption occurs between the left subclavian artery and the left carotid artery (70%–80% of the cases); an aberrant right subclavian artery is commonly associated with this type. In type C, the interruption is between the innominate artery and the left carotid artery (1%–5%).36,38,39
FIGURE 26-6.
Anatomic types of interrupted aortic arch. (A) Interruption distal to the left subclavian artery. (B) Interruption between the left subclavian and left carotid arteries. (C) Interruption between the left carotid and innominate arteries. AO = aorta; IA = innominate artery; LC = left carotid artery; LPA = left pulmonary artery; LS = left subclavian artery; MPA = main pulmonary artery; PDA = patent ductus arteriosus; RPA = right pulmonary artery.

Signs usually appear in the first days of life and include dyspnea, cyanosis, decreased peripheral pulses, and signs of CHF; findings become more accentuated when the ductus begins to close. Chest radiography shows cardiomegaly with increased pulmonary blood flow. In patients with DiGeorge syndrome, the upper mediastinum is narrow because of the absence of a thymus. ECG may show right ventricular hypertrophy. Echocardiography is usually diagnostic.5
If the IAA is left untreated, most patients die in 4 to 10 days, usually following closure of the ductus arteriosus. These subjects are ductus dependent; therefore, the ductus should be kept open with the use of prostaglandin E1 in order to avoid systemic hypoperfusion and to prevent an excessive pulmonary flow. Relative hypoxia should be maintained in order to prevent pulmonary vasodilatation since this would increase the pulmonary blood flow and decrease systemic perfusion.
The preferred surgical approach is aortic repair followed by closure of the VSD. The correction can be performed in one or two stages. In the single stage procedure, the repair consists of direct end-to-end anastomosis between the ascending and descending aorta. In some cases a graft is required for the repair. In the same operation, other associated anomalies are corrected. In the staged procedure, reconstruction of the aortic arch is performed by anastomosing the divided end of the left carotid artery to the descending aorta, ligation of the PDA, and pulmonary artery banding. The second stage of the procedure consists of closure of the VSD, debanding of the pulmonary artery, and pulmonary arterial reconstruction. Other procedures might be needed depending on the presence of associated anomalies. Initial inhospital mortality is usually less than 15% in most series, with long-term survival of 70% to 80% at 5 to 15 years. The most important factor associated with worse outcome is the presence of complex anomalies; other risk factors are increased age at the time of surgery, low weight, type B IAA, and the presence of the DiGeorge syndrome.36,37,38,39
Vascular rings comprise less than 1% of congenital heart lesions. Vascular rings can produce tracheal and esophageal compression. Respiratory symptoms are more common in younger patients and dysphagia is more common in older children. Although these anomalies are mainly identified in childhood, there are reports of these occurring in adults as well.42 Vascular rings have been generally divided into four classes: double aortic arch, right aortic arch, innominate compression, and pulmonary sling. Double aortic arch and right aortic arch are the two most common forms.43
Double aortic arch is a rare anomaly that is characterized by a complete vascular ring encircling the trachea and esophagus. A double aortic arch is responsible for 50% of all vascular ring anomalies. There is no gender or race predilection. The vascular ring results from persistence of the fourth arches and failure of the distal part of the right dorsal aorta to disappear. In more than 70% of the patients the right aortic arch is dominant and passes posterior to the trachea and esophagus. Associated anomalies include VSD, ASD, PDA, COA, and tetralogy of Fallot. Less frequently associated anomalies include truncus arteriosus, TGA, pulmonary atresia, and HLHS.1,44,45 Chromosome 22q11 deletions are present in 20% of patients with double aortic arch.46
Symptoms result from compression of the esophagus and trachea by the vascular ring. The severity of symptoms depends on the degree of compression by the vascular ring. The onset of symptoms is usually at birth but can be as late as 3 years of age. Respiratory symptoms are more common than gastrointestinal and cardiac symptoms. The most common respiratory symptoms are stridor and wheezing. Other respiratory symptoms include coughing, choking, upper and lower respiratory infection, dyspnea, increased oral secretions and, aspiration. The most common gastrointestinal symptom is choking with feeds. Other symptoms include dysphagia, failure to thrive, emesis, and cyanosis with feedings. Lastly, cardiac features include murmurs, cyanosis, chest pain, and CHF. Chest radiography sometimes shows narrowing of the trachea. An esophagogram will demonstrate an indentation in the esophageal wall. Echocardiography, MRI, or CT are diagnostic. Arch predominance is best determined by echocardiography or MRI. Aortic angiography is rarely needed.44,45,47
The treatment of a double aortic arch is surgical. Alsenaidi et al.44 in their series of 81 patients, approached the repair through a left lateral thoracotomy in 72 patients and median sternotomy in 5 patients. Surgery consists of resection of the atretic arch, ligation of the PDA or ligamentum arteriosus, and complete and thorough mobilization of the trachea and esophagus. Right side dominance was present in 56 patients (71%), left dominance in 16 (20%), and equal dominance in 7 patients (9%). Inhospital mortality was 3% and 5-year survival was 96%. Long-term outcome is excellent. Similar results were reported by Bonnard et al. and Backer et al.45,47 in a more heterogeneous group of patients that included other vascular ring abnormalities. Video-assisted thoracoscopic surgery has been reported in this population with good results.48
When the entire right dorsal aorta persists and the distal part of the left dorsal aorta involutes, a right arch results. When there is a right aortic arch, the arrangement of the three branches is reversed.1 Several types of vascular rings exist in which the aortic arch is right sided and they share the defining feature of all vascular rings, namely, encirclement of the trachea and esophagus by connected segments of the aortic arch and its branches. A right aortic arch may occur without forming a vascular ring. The presence or absence of a vascular ring in the setting of a right aortic arch depends on the branching of the brachiocephalic vessels and the location of the ductus arteriosus.49
Two primary forms of vascular ring with a right aortic arch exist. In the most frequent form, an aberrant origin of the left subclavian artery from a retroesophageal diverticulum (diverticulum of Kommerell) is present, which originates as the last branch of the aortic arch. The ring is completed by a left-sided ductus arteriosus (or its remnant ligamentum arteriosum) passing from the aberrant left subclavian artery to the proximal left pulmonary artery. In this type, the brachiocephalic vessels originate from the arch in mirror-image fashion with a left innominate artery as the first branch followed by the right common carotid and subclavian arteries. A left-sided ductus arteriosus or ligamentum arteriosum passes between the descending aorta and the proximal left pulmonary artery.49
As with double aortic arch, anomalies associated with right aortic arch include VSD, ASD, PDA, COA, and tetralogy of Fallot. Less frequently associated anomalies are truncus arteriosus, TGA, pulmonary atresia, and HLHS.1,44,45 Chromosome 22q11 deletions are frequently present in patients with right aortic arch.41,46
Clinical manifestations are similar to those for double aortic arch, although patients with a right aortic arch tend to present later than those with a double aortic arch. The most common symptoms are stridor, wheezing, and choking with feeds. Chest radiography sometimes shows narrowing of the trachea. An esophagogram shows an indentation in the esophageal wall. Echocardiography, MRI, or CT are diagnostic. Aortic angiogram is rarely needed.45,47
Surgical division of the vascular ring is indicated in any patient with symptoms of airway or esophageal compression and in patients undergoing surgery for repair of associated cardiovascular or thoracic anomalies. The procedure is performed through a left thoracotomy, the ductus arteriosus, or the ligamentum arteriosum is divided, and complete and thorough mobilization of the trachea and esophagus are performed. Results are similar to those for double aortic arch.45,47,48
Tracheal compression is caused by a more distal and posterior origin of the innominate artery. Patients present with stridor. Life-threatening symptoms may present as the innominate artery compresses the trachea anteriorly by more than 75% of the original tracheal lumen as it courses from the left of the mediastinum to the right arm.43 Bronchoscopy is essential at the time of surgery to confirm the diagnosis and results of repair. Suspension of the aorta and innominate artery to the sternum is the treatment of choice, with excellent results and minimal morbidity and mortality.50
Pulmonary artery slings are not true rings. The left pulmonary artery originates from the right pulmonary artery and encircles the distal trachea and right mainstem.43 Approximately 30% to 50% are associated with complete tracheal rings ranging from short segments to the entire length. Patients are usually younger than 2 years and present with stridor, wheezing, or respiratory distress. The procedure of choice to establish the diagnosis is echocardiography while a bronchoscopy is key to the assessment of tracheal stenosis. An esophagogram may show a distinctive anterior pulsatile indentation of the esophagus that is pathognomonic. Correction is accomplished by dividing and reanastomosing the left pulmonary artery anterior to the trachea to the main pulmonary artery, simultaneous resection, or tracheoplasty is also performed. Median sternotomy is the preferred approach.51
Sinus of valsalva aneurysm (SVA) develops as gradual bulging of a sinus of valsalva into the right atrium or the right ventricle. It is more common in Orientals and the male-to-female ratio is approximately 2 to 4:1. SVA is an uncommon defect responsible for 0.1% to 3.5% of all congenital heart lesions. In the United States and Western countries it is present in less than 0.3% of the congenital lesions. The right coronary sinus is more frequently involved (70%–90%), followed by the noncoronary sinus (10%–30%) and the left coronary sinus (<1%–6%). The aneurysm may rupture into the right ventricle (60%–90%), right atrium (10%–40%), or left atrium (<5%).52,53,54,55
The most commonly associated cardiac defects is VSD, which is present in more than 40% of patients; other associated anomalies include ASD, pulmonary valvular stenosis, subaortic stenosis, BAV, and COA.52,53,54,55
The essential lesion in the vast majority of cases is progressive separation of the aortic media of the sinus from the aortic valve cusp. The congenital weakness in this region gradually gives away under aortic pressure to form an aneurysm. A deficiency of normal elastic tissue and abnormal development of the bulbus cordis have been associated with the development of SVA. Acquired SVA are even less common than congenital aneurysms. Acquired aneurysm are caused by conditions that cause degeneration of the media including infections (syphilis, bacterial or fungal endocarditis or tuberculosis), atherosclerosis, cystic medial necrosis, connective tissue disorders, inflammatory diseases (Takayasu’s arteritis, Behcet’s disease), direct trauma, or surgical complications during the repair of a VSD or removal of a calcified aortic or mitral valve.52,55,56
Unruptured aneurysms are asymptomatic and produce no signs, but occasionally can cause compression and distortion of surrounding structures. Aneurysm rupture usually occurs in the third or fourth decade of life and is accompanied by chest pain, dyspnea, palpitations, syncope, myocardial ischemia, arrhythmias, a machinery-like murmur, and bounding peripheral pulses with the development of CHF. In more than 50% of cases there is a sudden onset of symptoms with life-threatening hemodynamic deterioration as a consequence of acute aortic regurgitation, coronary steal, or massive left-to-right shunt. In a small subset of patients with small perforations that remain limited or increase in size gradually, patients may remain asymptomatic for a prolonged period until symptoms of CHF develop. Sudden cardiac death may result from tamponade, myocardial ischemia, conduction disturbances, or arrhythmias.53,54,55,57 Chest radiography shows cardiomegaly with increased pulmonary blood flow. Sometimes it is possible to visualize the bulging caused by the aneurysm. Echocardiography is diagnostic in most cases. CT has not been widely used to diagnose SVA. MRI has proven very useful in the diagnosis of SVA. MRI and cine MRI may define the anatomy precisely, demonstrate wall thickness, the presence of thrombus, and small perforations better than aortography. Diagnostic cardiac catheterization is rarely necessary although can be useful in evaluating the hemodynamic significance of the rupture, associated cardiac defects, and coronary anatomy.53,55
Small- to moderate-sized asymptomatic unruptured aneurysms are usually followed. Surgical repair is indicated for symptomatic unruptured aneurysms and aneurysms that cause hemodynamic alterations, including significant aortic regurgitation and ventricular outflow tract obstruction. Other indications include infection, malignant arrhythmias, acute ostial coronary artery obstruction, associated congenital defects, and rupture. In the latter case, surgery should be done as soon as possible to avoid the development of CHF.52,53,55
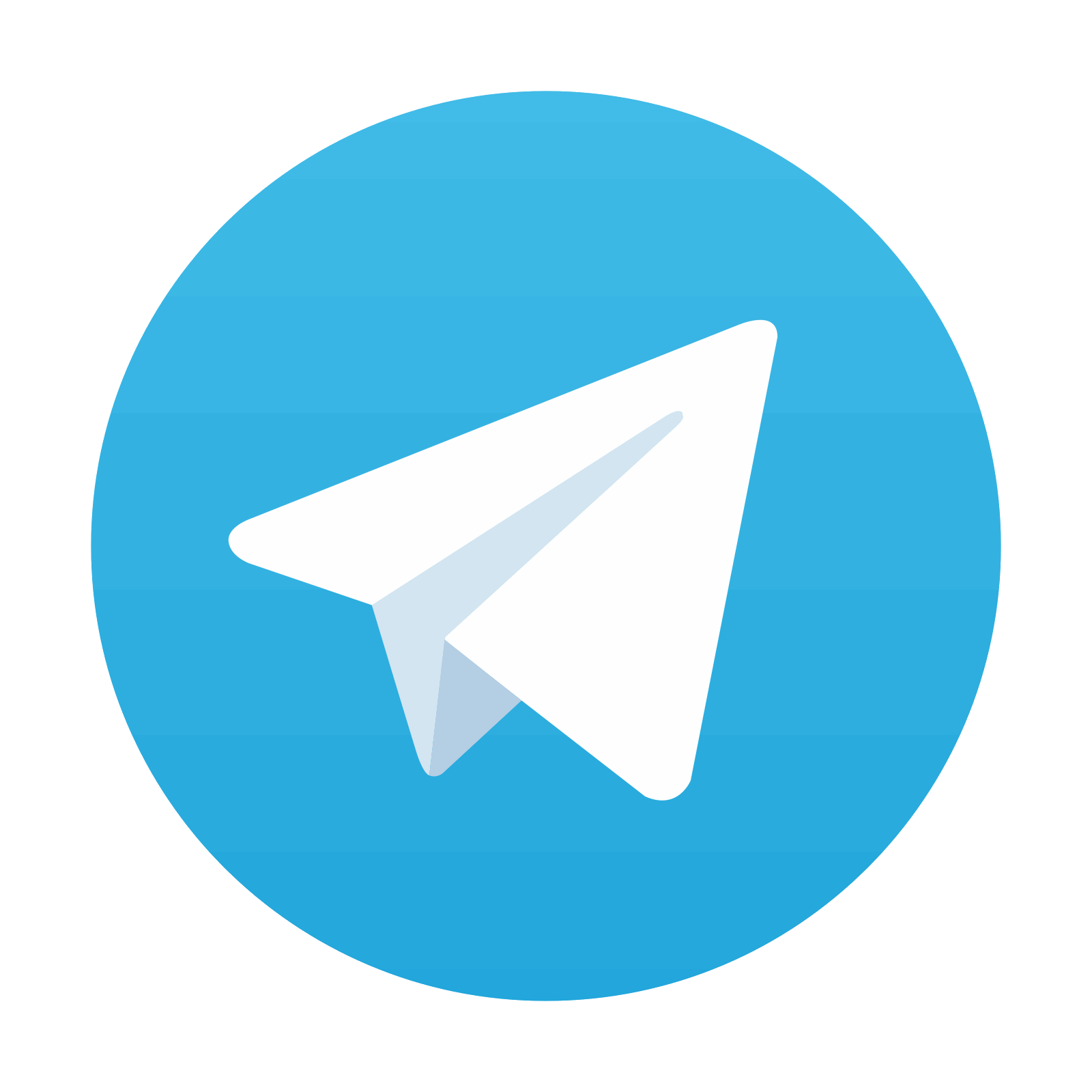
Stay updated, free articles. Join our Telegram channel

Full access? Get Clinical Tree
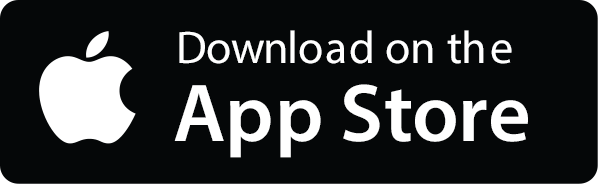
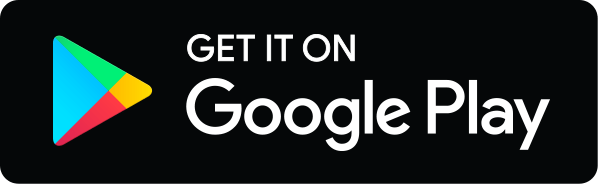