Transcutaneous Measurement of Oxygen Saturation
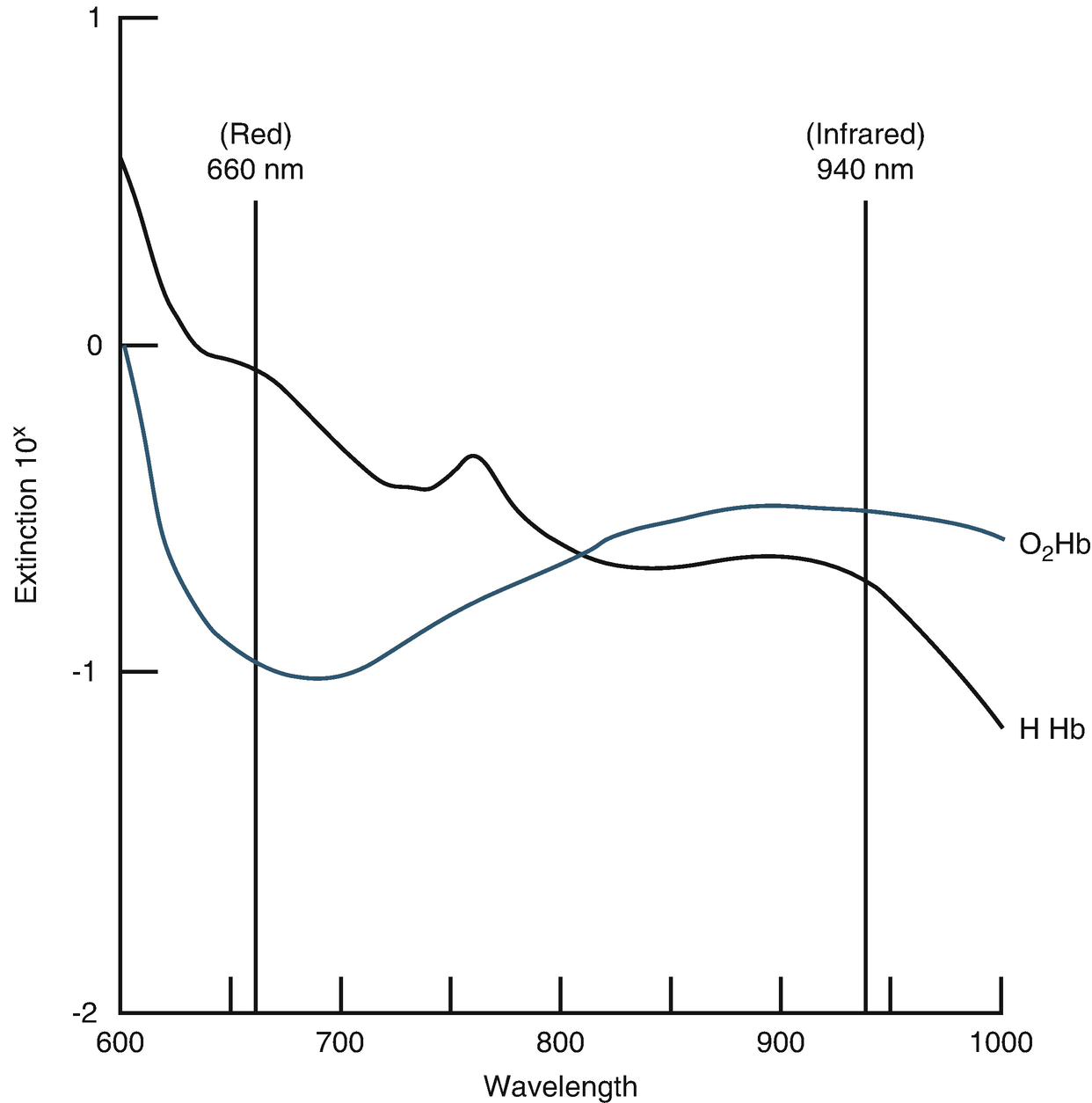
Absorption mechanism used in pulse oximetry
Factors That Affect Oxygen Saturation Measurement
Pulse oximeters are relatively easy to use. They do not require calibration or preheating of the patient’s skin, and they can be used immediately with all types of patients of any age. However, there are factors that can affect peripheral oxygen saturation (SpO2) measurement and interpretation, which are discussed below.
Type of Sensor and Its Placement
The location of the sensor is the most important factor in obtaining a real SpO2 value. Incorrect placement results in an optical shunt, which happens when the light received by the photodiode does not pass correctly through the tissue to be analyzed. Incorrect placement can also result in exposure to ambient light, which can affect the measurement. In most cases where this occurs, ambient light accounts for 85% of SpO2 readings, which is approximately the extrapolated value that corresponds to a ratio of 1:0 between red and infrared light. Overestimations occur as a result of exposure to an excess of ambient light, which can lead to a belief that a patient is properly saturated, while in reality the values are low. Actual hypoxemia may pass undetected in these patients.
An optical shunt can also occur in the absence of ambient light. This is because in the majority of oximeter devices, the red light and infrared emission diodes are 2–3 mm apart. If the sensor is detached and one of the diodes is left out, it can produce falsely low (60–70%) or high (95–100%) SpO2 values, depending whether it is the infrared diode or the red-light diode that is uncoupled, respectively. To avoid this situation, which can result in clinical complications for the patient, proper placement of the sensor should be ensured. The light emitter should be facing the receiver, with both correctly covered to avoid contact with ambient light. The sensors should be fastened to a fabric that does not let light pass through. The sensors should not be fastened to the skin with excessive force (over 50 mm Hg) that could affect the signal-to-noise ratio and severely disturb the operation of the SpO2 sensor.
Another less common problem with pulse oximeters is skin lesions by burning. This usually occurs when a different type of sensor is used from that determined by the oximetry equipment or when the surface of the sensor has been damaged. The sensor should be checked to ensure it is the correct one, and its position should be changed every 8 hours.
It is worth noting that, to date, little attention has been given to the performance of different types of sensors in newborns and infants. In fact, we know of no study that has systematically assessed the differences among sensors used in this age group. In the experience of the authors, more flexible sensors provide more reliable SpO2 measurements in young patients, given that these sensors can be applied more easily, without the need for a lot of pressure to fasten them. The problem with flexible sensors is that they tend to be more expensive than more fixed or rigid sensors.
Peripheral Perfusion
Pulse oximeters depend on peripheral perfusion, and most of the equipment currently available requires at least 20 mm Hg of pulse pressure or systolic pressure of over 30 mm Hg to operate adequately and reliably. The efficiency of the pulse oximeter falls significantly if the pressure falls below these thresholds. The most reliable test of correct functioning is the signal-to-noise ratio, which can be appreciated through the pulse curve, built into most new oximeters. There are significant variations among the different pulse meter brands with respect to the SpO2 reading in situations of hypotension—for example, shock.
Response Time
In theory, the response time of the pulse oximeter depends only on the time it takes the oxygenated blood to reach the point where the sensor is located, which is 4 seconds to the toe of a newborn, 4.5 seconds to that of an infant, and 5.5 seconds to that of a child of around 5 years of age. However, the pulse oximeters available nowadays average the values registered in a range from 2 to 15 seconds (average time) or 4 to 32 beats per minute, to avoid errors and provide a more reliable reading of SpO2.
This procedure attempts to reduce the number of false alarms. The longer the average time of these values is, the more slowly the equipment reacts to changes. For example, fewer alarms sound with postural changes; however, this extends the time that the physician has to react to real changes in SpO2 or it can lead to false low SpO2 values if the patient is constantly moving, and this might falsely suggest the presence of hypoxemia. Erroneous readings can be indistinguishable from real hypoxemia, particularly without pulse plethysmography curves. In addition, the use of the average time can lead to erroneous conclusions in situations in which a very precise measurement of SpO2 is required. An example is the decision to use supplementary oxygen in newborns and infants with bronchopulmonary dysplasia. The association between oxygen desaturations and cardiorespiratory events such as apnea necessarily require a short average time. Finally, the different average SpO2 times make it impossible to define normal values in a population and extrapolate them to oximeters with different average times. Therefore, all of the reference values obtained with a specific pulse oximeter can be extrapolated only to subjects in which the same equipment is used.
These problems can be overcome by using the beat-to-beat mode, which provides an SpO2 value for every heartbeat. However, every clinical unit must decide to what extent it needs SpO2 measurements and whether oximetry is intended for adequate diagnosis (for example, polysomnography) or for more general monitoring and detection of threatening situations on the basis of determined limits.
Motion Artifacts
The pulsatile (arterial) component contributes only 1–5% of the total absorbance as measured by the pulse oximeter. Consequently, the instrument is very sensitive to signal changes—for example, body movement. It is particularly important to identify the presence of movements in order to discount them. This can be done by analyzing the plethysmograph curve from the SpO2 reading. Ideally, the plethysmograph curve should be complete. The SpO2 values are not reliable and must be discarded if the curve is disrupted or incomplete. A less reliable alternative is to compare the pulse rate and the heart rate obtained with the electrocardiograph, because the two rates should be identical. Without a system for validating and excluding artifacts, SpO2 values should not be interpreted.
Influence of Changes in Hemoglobin and Skin Pigmentation
Pulse oximeters only use two light wavelengths. Consequently, they cannot identify abnormal hemoglobin or pigments that can interfere with the reading. Some of these pigments or distinct forms of hemoglobin—among them fetal hemoglobin—absorb light, which results in false readings. Methemoglobinemia will give values of around 85% of SpO2, and carbon monoxide intoxication will result in falsely high SpO2 values. Bilirubin generally does not alter pulse oximeter readings.
Reference studies of skin pigmentation and SpO2 have yielded variable results. A study involving nine premature African American newborns concluded that a Nellcor N100® overestimated SpO2 by 5% in two cases, but the others were very precise. Another study showed falsely high SpO2 levels in black adults but only if the SpO2 was <90%. At higher levels, there are no discrepancies between SpO2 and SaO2. This matter is remains unclear, and more studies are required, especially in relation to children.
Influence of Specific Algorithms
Pulse oximeters extrapolate their measures to a table of predetermined values, which is based on empirical data obtained in adults. Consequently, these instruments should be revalidated to be used in children. Studies that have compared different types of pulse oximeters have found statistically significant differences in their results. Moreover, the same equipment can yield significant differences when applied to different age groups.
These differences among devices can be partly explained by the ways in which the equipment shows or calculates SpO2. Examples of this are the Nellcor® oximeter, which measures functional SpO ([HbO2/(HbO2 + RHb)] ∗ 100)—where RHb is reduced hemoglobin—as compared with the Ohmeda® oximeter, which estimates the fractional SpO [HbO2/(HbO2 + RHb + MetHb + COHb) ∗ 100]—where MetHb is methemoglobin and COHb is carboxyhemoglobin. The former equipment estimates SaO2 by simply subtracting 2%, which represents MetHb and COHb concentrations, and displaying an SpO2 that is 2% less than the fractional SpO2. However, in the clinical context, functional SpO2 is preferred, and COHb and MetHb are determined only in patients in whom clinical suspicion requires them.
The differences in the values obtained in studies using the same equipment could be caused by the way in which motion artifacts are discounted. For reasons that are explained below, another important factor is the SpO2 values that are measured: the lower the SpO2 range is, the less precise the measurement is. The differences can likewise be explained by different types of software. This can explain why in a 1990 study the same Ohmeda Biox III/3700® underestimated real SaO2 values by 2.9%, while in 1991 this same equipment overestimated SaO2 by only 0.4%. Reassessments should be done when every new version of the software is installed.
Precision in Detecting Hyperoxemia and Hypoxemia
Pulse oximeters derive their measurements from algorithms based on empirical in vitro data on oxygen saturation values obtained from healthy adult volunteers, in which SpO2 generally varies within a normal range around 100%. Most suppliers maintain that their equipment shows SpO2 values at close to ±4–6% of the real SaO2 values. Normal SpO2 levels in infants and preschool children are in the same range as those in adults. However, with SpO2 below 75–80%, the values are simply extrapolated from the algorithm obtained with higher SpO2 values. This procedure can result in underestimation of the real oxygen saturation values. Given the above, we do not know the real sensitivity of oximeters to screen for low SaO2 values in seriously ill children—for example, those in shock or with associated infectious problems.
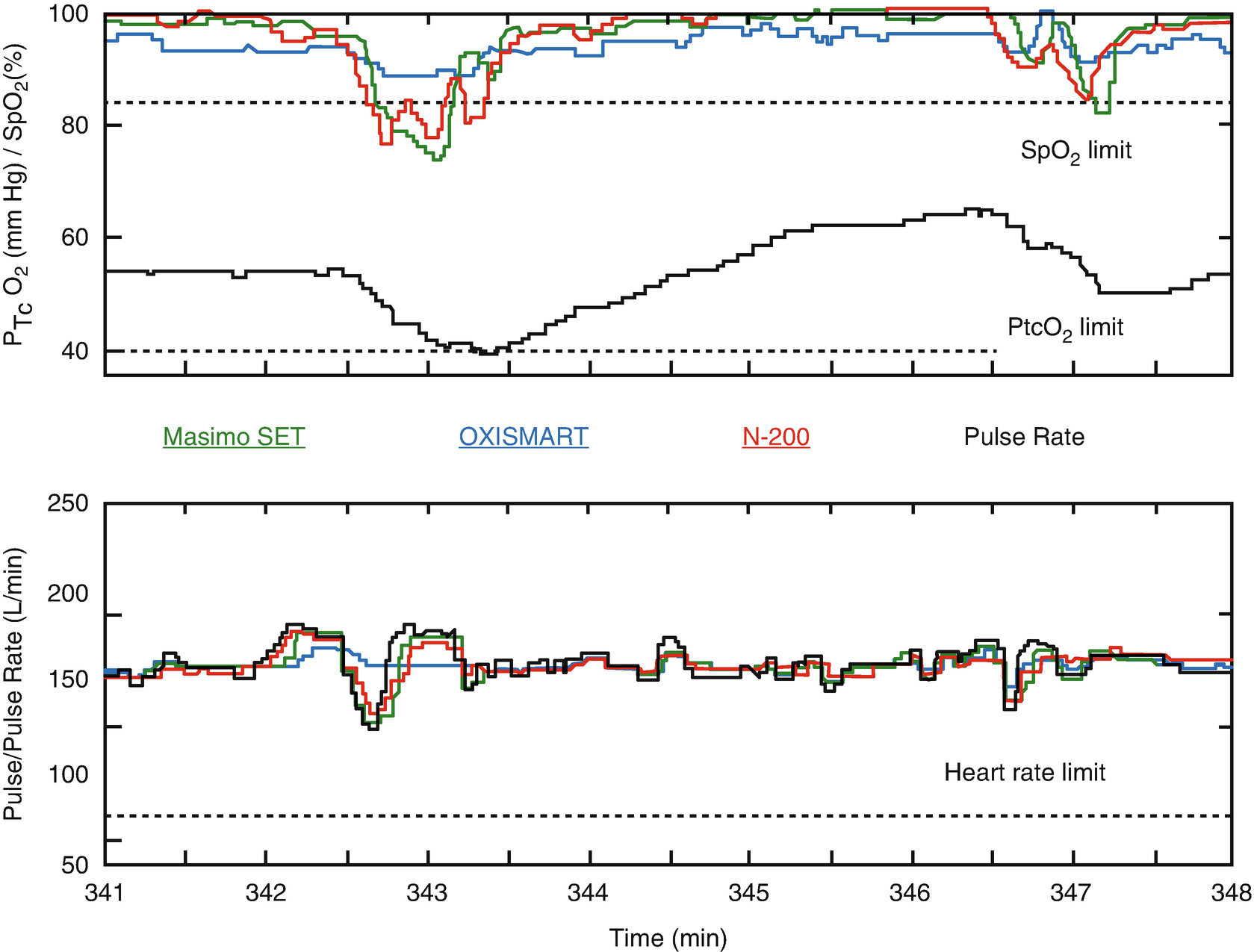
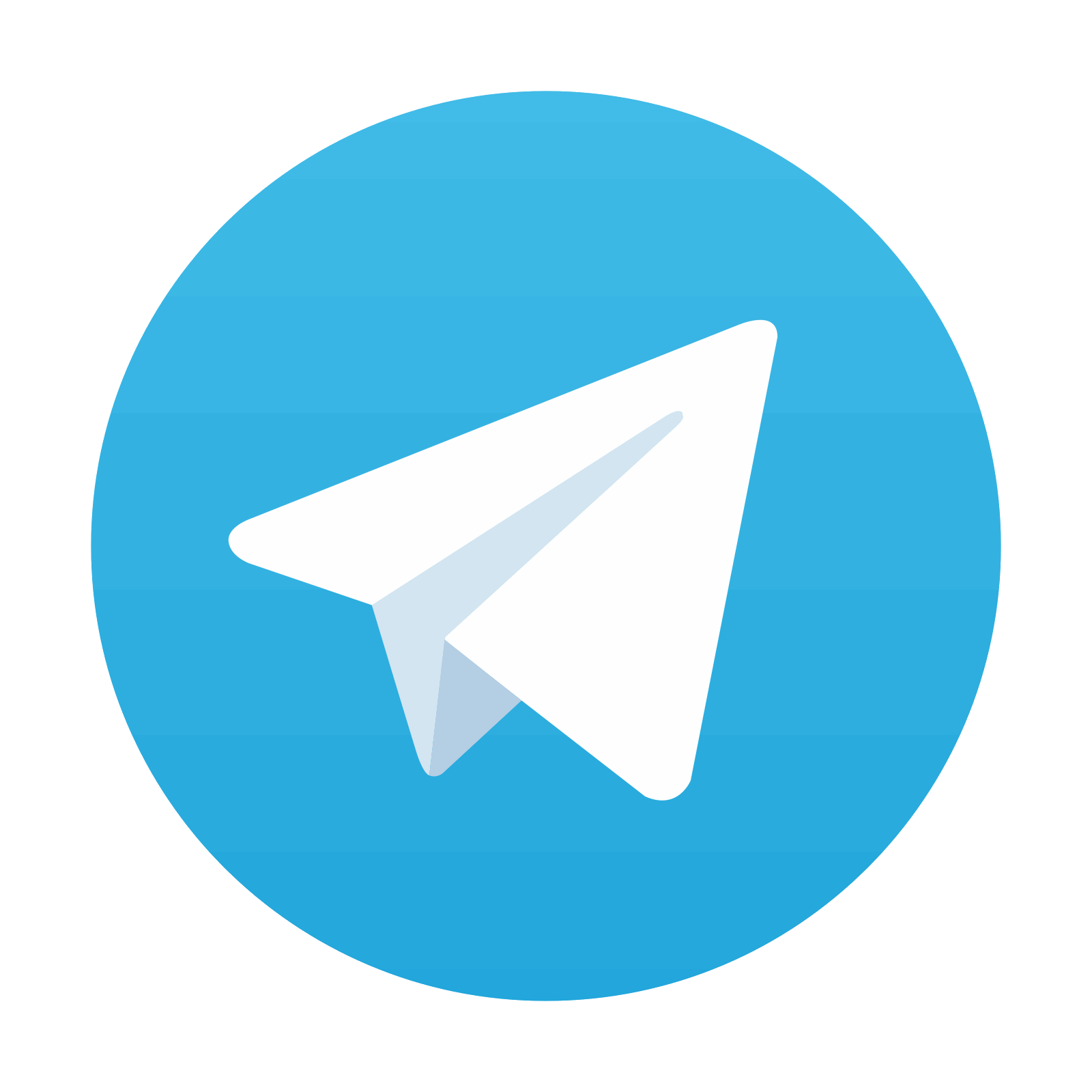
Stay updated, free articles. Join our Telegram channel

Full access? Get Clinical Tree
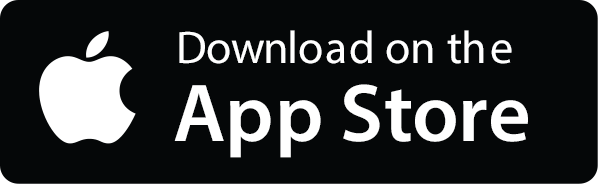
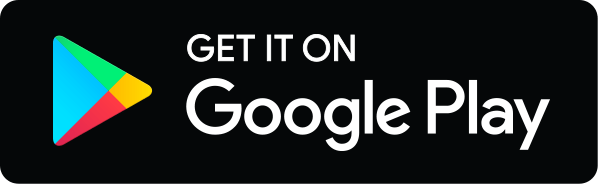