Apoptosis is recognized as a mechanism of cell loss in the setting of acute myocardial infarction (AMI). Whether it contributes to myocyte elimination late after AMI has not yet been confirmed. We attempted to identify the features of apoptosis in myocytes that survived AMI. A search for ongoing apoptosis was performed in samples obtained from 38 human hearts: group I (n = 10), noncardiac death (control); and group II (n = 28), left ventricular aneurysm (in 20 patients, the samples were collected during aneurysmectomy and from 8 at autopsy). The morphometric evaluation included the degree of cellular hypertrophy, density of the capillary network, extent of myocytolysis, and features of apoptosis. Immunohistochemistry for caspase-3 and Bcl-2 was used as a prerequisite for transmission electron microscopy. Slides showing the strongest reaction for caspase-3 and negative for Bcl-2 were selected for transmission electron microscopy. CD-34 immunohistochemistry was used to quantify the capillary density. A significant reduction in capillary density was observed compared to the control group (1,085.6 ± 205.0/mm 2 vs 2,968.7 ± 457.3/mm 2 ; p <0.001). Myocytes that survived the acute phase of AMI were significantly hypertrophied (24.5 ± 4.7 μm vs 14.5 ± 1.6 μm; p <0.001) and showed a moderate to severe degree of myocytolysis. The average intensity score of the immunohistochemistry reactions for caspase-3 was 8.2 ± 3.8. Using transmission electron microscopy, apoptotic bodies were found in caspase-3–positive samples. In conclusion, the expression of caspase-3 and the presence of apoptotic bodies confirmed apoptosis as a common pathway of cardiomyocyte death in the setting of a limited blood supply after AMI.
The process of programmed cell death has been extensively investigated in relation to various cardiovascular ailments in recent years. Apoptotic myocyte cell death has been documented in the setting of acute myocardial infarction (AMI) and for up to several weeks after AMI and in idiopathic dilated cardiomyopathy, end-stage heart failure, atrial fibrillation, and cardiac aging. Since it became generally accepted that apoptosis can occur in terminally differentiated cells, such as cardiomyocytes, conflicting data regarding the selection of patients, sample collection, methods of research, and the assumption of the apoptotic rate have been reported. The results of most studies, both experimental and pathologic, used co-localization of in situ DNA nick end-labeling and immunohistochemistry (IHC) to detect the different proteases that mediate the apoptotic execution phase. Of these proteases, activated caspase-3 was the most frequently studied. Few investigators, however, confirmed their findings using transmission electron microscopy (TEM).
The aim of the present morphologic study was to identify the features of ongoing apoptosis in myocardiocytes that survived AMI and to assess its relation to alterations in the capillary network. To ascertain whether apoptosis contributes to the myocyte elimination that occurs months or even years after AMI, the results from both IHC and TEM performed on samples collected from human myocardium were analyzed.
Methods
The investigation conformed to the principles outlined in the Declaration of Helsinki.
Myocardial samples from 38 human hearts were studied in 2 groups. Group I (n = 10) consisted of patients who had died from noncardiac causes (cerebral injuries or malignant tumors) and served as the control group (mean age 52.4 ± 11.2 years). Group II (n = 28) included patients aged 38 to 72 years (mean 57.8) who had been diagnosed with a true left ventricular (LV) aneurysm. A LV aneurysm, defined as a convex protrusion of the full thickness of the ventricular wall and composed of a mature scar, was found during autopsy examination in 8 patients who had previously been diagnosed with Q-wave AMI ( Table 1 ). None of these patients had undergone reperfusion during the acute phase of AMI, and the most common cause of death was progression of heart failure or electrical instability associated with the aggravation of angina pectoris. Autopsies were performed 12 to 36 hours after death and the following parameters were investigated: LV mass, infarct location and size, wall thickness of the infarcted area and the infarct-free LV wall, and the propensity of atherosclerosis. The major coronary arteries, isolated beginning from Valsalva’s sinus up to the technically accessible distal parts, were transected and analyzed at 5-mm intervals. To assess the degree of coronary obstruction, the sections were stained according to Weigert’s method and, using the quantitative planimetric option of a computer program, the cross-sectional area of the lumen, intima, and media were measured. A total of 20 patients were diagnosed with a true LV aneurysm, defined as a segment of the ventricular wall showing dyskinetic systolic expansion during ventriculography and/or echocardiography several months after Q-wave AMI ( Table 1 ). The aneurysm samples were collected during routine combined procedures of coronary artery bypass grafting and aneurysmectomy. The examination of the coronary arteries in patients who underwent anurysmectomy was done using coronary angiography. Islets of myocytes suitable for morphometry were found in 19 of 28 analyzed aneurysms.
Pt. No. | Age (years) | Interval from AMI to Sample ⁎ (mo) | IRA Stenosis (%) | Capillary Density (/mm 2 ) | Myocyte Diameter (μm) | Myocytolysis | Caspase-3 IHC (R-S Scale) |
---|---|---|---|---|---|---|---|
1 | 50 | 72 | 98.4 † | 1,288.6 | 24.5 ± 4.5 | ++ | 8 |
2 | 67 | 6 | 99.6 † | 1,260.1 | 21.2 ± 5.6 | +++ | 12 |
3 | 61 | 130 | 98.8 † | 1,089.1 | 28.2 ± 4.3 | ++ | 6 |
4 | 38 | 71 | 99.8 † | 954.7 | 24.7 + 3.9 | ++ | 12 |
5 | 56 | 12 | 99.4 † | 1,394.1 | 21.9 ± 4.1 | + | 8 |
6 | 68 | 46 | 98.0 † | 1,241.0 | 26.8 ± 4.7 | ++ | 8 |
7 | 69 | 6 | 100 ‡ | 1,004.4 | 25.4 ± 3.4 | ++ | 6 |
8 | 51 | 48 | 100 ‡ | 832.0 | 24.8 ± 5.2 | ++ | 8 |
9 | 55 | 12 | 100 ‡ | 1,015.1 | 24.3 ± 4.7 | ++ | 12 |
10 | 68 | 6 | 100 ‡ | 1,042.1 | 20.6 ± 2.6 | + | 3 |
11 | 59 | 60 | 100 ‡ | 1,084.5 | 27.8 ± 7.8 | +++ | 8 |
12 | 56 | 24 | 100 ‡ | 1,189.3 | 27.4 ± 6.6 | ++ | 12 |
13 | 50 | 23 | 100 ‡ | 1,356.7 | 22.3 ± 4.1 | + | 2 |
14 | 49 | 12 | 100 ‡ | 1,198.2 | 19.9 ± 5.2 | +++ | 12 |
15 | 65 | 120 | 100 ‡ | 1,307.2 | 27.7 ± 5.7 | + | 12 |
16 | 72 | 6 | 100 ‡ | 1,143.7 | 28.4 ± 3.9 | ++ | 12 |
17 | 69 | 36 | 100 ‡ | 808.1 | 21.4 ± 4.7 | +++ | 12 |
18 | 38 | 88 | 100 ‡ | 743.3 | 22.8 ± 6.0 | + | 2 |
19 | 57 | 12 | 100 ‡ | 673.6 | 25.4 ± 6.0 | + | 2 |
Mean ± SD | 57.8 ± 9.9 | 42 ± 38 | 1,085.6 ± 205.0 | 24.5 ± 4.7 | 8.2 ± 3.8 |
⁎ Samples taken at death or aneurysmectomy.
† Reduction of cross-sectional IRA lumen assessed at autopsy.
The myocardial samples collected at autopsy and aneurysmectomy were fixed in 10% buffered formalin (pH 7.4) for 24 hours, processed, and embedded in paraffin blocks. All blocks were sectioned at a thickness of 5 μm, and step sections of 40 to 50 cuts were selected for morphometric evaluation. The slides for the morphologic and morphometric studies were coded and evaluated by 2 independent observers. The following parameters were evaluated: myocyte diameter, myocyte nucleus diameter, myocyte nuclear density, and capillary density.
Myocytolysis, defined as a loss of muscular striation and displacement of muscle content by cytoplasmic vacuoles, was assessed semiquantitatively: 0, none; +, mild (myocytolysis visible in ≤30% of myocytes; ++, moderate (30% to 60% of visible myocytes); and +++, severe (>60% of myocytes showing varying degrees of myocytolysis).
A morphometric evaluation was performed using a Leica Q500 MC computer-based image analyzer (Leica, Cambridge, United Kingdom), and the measurements were performed using a computer program (Leica Q500 MC QWin, Leica, Cambridge, United Kingdom). The results were automatically recalculated for the area of 1 mm 2 .
Details of the autopsy examinations, examination of the coronary arteries, sample collection, and morphometric measurements have been previously described.
Sections of 5 μm were cut and mounted on positively charged glass slides (Superfrost Plus, Thermo Scientific, Thermo Fisher Scientific, Waltham, Massachusetts). The sections were deparaffinized, rehydrated, and washed in Tris-buffered saline. Microwave-based antigen retrieval for the Bcl-2 oncoprotein was performed using Dako Target Retrieval Solution (pH 9, Dako, Glostrup, Denmark) for 30 minutes with the microwave set at 250 W. No antigen retrieval procedure was necessary for caspase-3. After the antigen retrieval procedures, all the slides were incubated in 3% hydrogen peroxide for 10 minutes to block endogenous peroxidase activity, followed by an additional incubation of 10 minutes in Tris-buffered saline. Next, the sections were incubated with the primary antibodies. For IHC, the following protocols were used for the primary antibodies in humidity chambers: caspase-3 (monoclonal mouse, 1:200, NovoCastra Laboratories, Newcastle Upon Tyne, United Kingdom, clone JHM62, code NCL-CPP32) overnight at 4°C, Bcl-2 oncoprotein (monoclonal mouse, 1:50, Dako, clone 124, code M 0887) at room temperature for 1 hour, and CD34, specific for endothelial cells (monoclonal mouse, 1:40, NovoCastra, clone QBEnd/10, code NCL-END) overnight at 4°C. After incubation, all sections were washed in Tris-buffered saline and subsequently incubated in Dako EnVision + System-HRP at room temperature for 30 minutes. The reaction results were visualized using diaminobenzidine chromogen at room temperature for 5 minutes. Finally, the sections were counterstained with hematoxylin, dehydrated, cleared in xylene, and coverslipped.
The intensity of IHC reactions for caspase-3 was evaluated according to the Remelle-Stegner scale. In brief, the percentage of positive cells within the studied area was classified as 0 (none), 1 (0% to 10%), 2 (10% to 50%), 3 (51% to 80%), or 4 (>80%), and the intensities of the IHC reactions were assessed as 0 (none), 1 (mild), 2 (moderate), and 3 (strong). The total score ranged from 0 to 12. Slides showing the strongest reaction for caspase-3 and negative for Bcl-2 (an inhibitor of apoptosis) were selected for additional ultrastructural studies.
After deparaffinization, the slides were rehydrated and treated with 0.1% osmium tetraoxide for 20 minutes and washed in 0.1 m sodium cacodylate buffer. Next, the slides were processed through a series of alcohols (70%, 90%, absolute), propylene oxide, and increasing concentrations of resin. Finally, onto the slides (in the area of interest selected by the corresponding studies of hematoxylin-eosin and IHC step sections), capsules with resin were placed upside down and left for polymerization at 60°C. After polymerization, the capsules with embedded tissue fragments were detached from the glass and, after trimming an appropriate area, ultrathin sections were cut. The sections were stained with aqueous uranyl acetate and citric lead solutions and studied using TEM (LEO 912/ab, Zeiss, Oberkochen, Germany).
The data are presented as the mean ± SD, computed from the average measurements obtained from each tissue sample. To confirm the normal distribution of variables, the Shapiro-Wilk test was used. The statistical significance was determined using the Student t test or the Mann-Whitney U test, as appropriate. p Values <0.05 were considered significant.
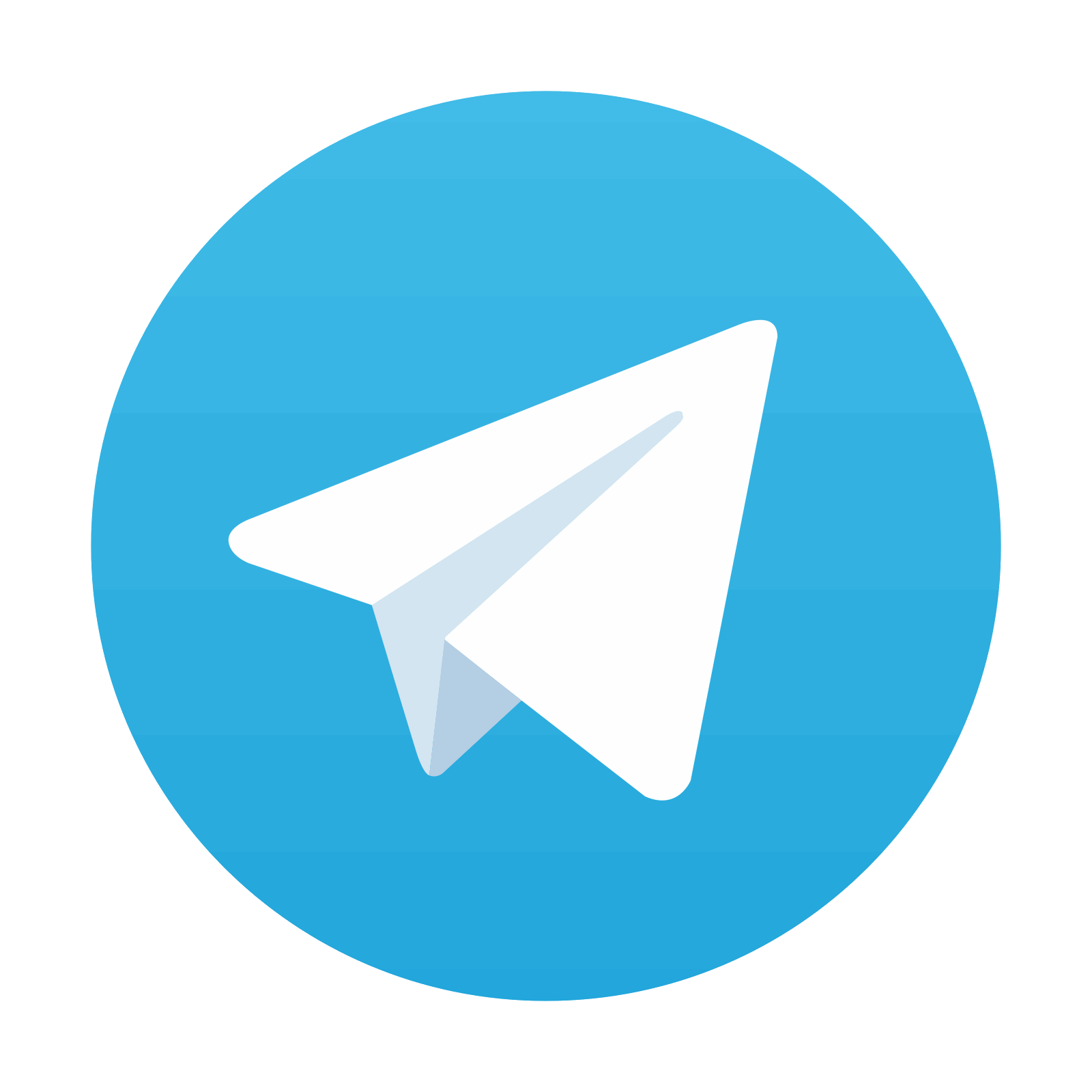
Stay updated, free articles. Join our Telegram channel

Full access? Get Clinical Tree
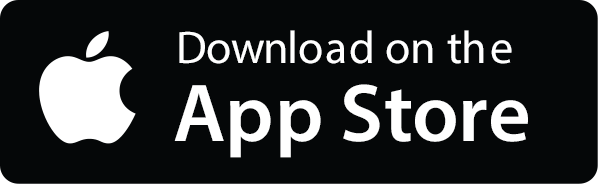
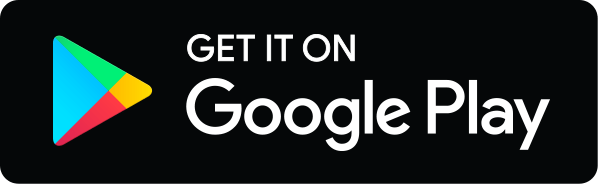