9 Anticoagulation in Percutaneous Coronary Intervention
The Biology of Coagulation: Therapeutic Targets
The Central Role of Thrombin
The disruption of endothelial integrity and the expression of prothrombotic molecules such as tissue factor lead to the activation of the soluble coagulative proteins (Fig. 9-1). This amplifying cascade converges on the generation of activated factor X (FXa) and the prothrombinase complex, which leads to the conversion of thrombin from its parent molecule prothrombin. Thrombin generation leads to multiple effects influencing the formation of thrombosis.1 Specifically, thrombin catalyzes the conversion of fibrin from fibrinogen enabling clot formation while also activating factors V, VIII, and X, thus promoting its own generation. In addition, via direct effects on the protease-activated receptor 1 (PAR1), thrombin promotes platelet activation leading to the expression of CD40 ligand, P-selectin, and the glycoprotein (GP) IIb/IIIa receptor, as well as the secretion of vasoactive agents, including adenosine diphosphate, serotonin, and thromboxane A2 (TXA2). The direct effects of thrombin on endothelial cells and smooth muscle cells result in the expression of adhesion molecules enabling platelet and leukocyte attachment, while its effect on endothelial membrane permeability contributes to the transmigration of the cellular and cytokine-mediated inflammatory response within the vascular wall. While thrombin promotes vasodilation in the intact endothelium, it contributes to vasoconstriction where the endothelium is damaged or denuded. Thrombin also appears to promote fibroblast cytokine production and is mitogenic (Fig. 9-2). However, thrombin has a short circulating half-life, and in the context of a normal endothelial barrier, the effects of thrombin are tightly controlled by a negative feedback mechanism. Anti-thrombin is a single-chain plasma glycoprotein produced by the liver. As an inhibitor of coagulation, this molecule has the ability to bind to thrombin, FXa, and FIXa in equimolar concentrations. Anti-thrombin’s action is increased over 1000-fold by the binding of pentasaccharide chain–containing heparins. The pentasaccharide sequence enables the binding of heparins to anti-thrombin and augments the binding affinity of thrombin and the other clotting factors. Anti-thrombin is also activated by the glycosaminoglycan heparin sulfate, which is found on the surface of endothelial cells. Other pathways for the inhibition of thrombin exist. These include the binding of thrombin to thrombomodulin and protein C, together with protein S. This inactivates the upstream coagulation proteins, FVa and FVIIIa, and promotes the release of tissue plasminogen activator (TPA). Hence, thrombin plays a central effector role in the vascular response to balloon-induced and stent-induced vascular injuries and remains an important therapeutic target for the prevention of ischemic complications during PCI. A schematic of the structure of the thrombin molecule is presented in Fig. 9-3. Separate substrate recognition sites are involved in the binding of heparin, fibrinogen, and thrombomodulin, and the catalytic site is responsible for the serine protease activity and is blocked by the direct thrombin inhibitors.
Adverse Events Following Percutaneous Coronary Intervention
Improvements in interventional techniques and refinements in anti-thrombotic therapies have led to a decline in the incidence of ischemic complications following PCI. Hence, further iterations in anti-thrombotic strategies can be considered a “two-edged sword,” with improved prevention of ischemic complications potentially leading to an increase in bleeding complications (Table 9-1). While the relationship between peri-procedural myocardial infarction (MI) has been widely debated, several studies using data from large-scale clinical trials demonstrate an increased risk of mortality with CK-MB (creatine kinase–muscle brain) elevations of greater than three times the upper limit of normal. In an analysis of patients enrolled in the REPLACE-2 study, CKMB elevation greater than or equal to three times the upper limit of normal was associated with a 3.5-fold increased risk of mortality at 12 months and accounted for 13.2% of all mortality seen by 12 months (Fig. 9-4).2 Therefore, this underpins the threshold definition for peri-procedural (within 48 hours) MI within many PCI trials of adjunctive pharmacotherapy. Similarly, the clinical significance of postprocedural bleeding events has undergone greater scrutiny over recent years. While these analyses have been hampered by nonstandardized approaches to the recognition and reporting of bleeding events in clinical trials and the lack of routine assessment of blood loss following PCI, a substantial increase in early and late mortality associated with TIMI (thrombolysis in MI) major and minor bleeding following PCI is evident. In an analysis by Kinnard et al., who examined 10,974 patients over a 10-year period, major bleeding events were associated with an approximately 10-fold excess in mortality and approximately three-fold increase in non–Q wave MI.3 Urgent revascularization and Q-wave MI were also increased. These observations are supported by analyses of patients enrolled in the ACS trials that report comparable rates of 12-month mortality rates of 12.2% and 11.3% associated with bleeding and ischemic events within 30 days, respectively. In an analysis of the REPLACE-2 study, TIMI minor or major bleeding was associated with a 2.3-fold relative risk of 12 month mortality and accounted for 3.9% of all the mortality observed in this population, while a bleeding event that met the TIMI major criteria was associated with a 6.1-fold increased mortality risk (see Fig. 9-4). In a further analysis of patients with ACS undergoing invasive management in the Acute Catheterization and Urgent Intervention Triage Strategy (ACUITY) study, using a similar methodology, reported that the late mortality hazard was associated with MI, again similar to major bleeding, 2.7-fold and 2.9-fold (both P < 0.001), respectively.4 The clinical characteristics independently associated with bleeding and ischemic events among these ACS patients are displayed in Table 9-2. Within this analysis, more late mortality was attributable to bleeding not associated with coronary artery bypass grafting (CABG) than to MI (11.7% vs. 9.1%), highlighting the greater significance of bleeding events among patients with ACS receiving invasive management. From a clinical perspective, a greater consideration of the relative ischemic and bleeding risks among patients undergoing PCI, especially in the context of ACS, is required when choosing anti-thrombotic agents within modern interventional practice.
TABLE 9-1 Clinical Endpoint Definitions Bleeding and Ischemia Commonly Used in Clinical Trials of Anti-Thrombotic Agents in Percutaneous Coronary Intervention
Endpoint | Definition |
---|---|
Myocardial infarction (MI) (post-PCI) | Creatine kinase (muscle brain) CK-MB elevation >3 times the upper limit of normal or the development of new Q waves; if CK-MB is unavailable, total CK may be used |
Myocardial infarction (post-CABG) | CK-MB elevation >5 times the upper limit of normal and the development of new Q waves, or CK-MB elevation >10 times the upper limit of normal without new Q waves; if CK-MB is unavailable, total CK may be used |
Myocardial infarction (non–peri-procedural) | CK-MB elevation >2 times the upper limit of normal or the development of new Q waves; if CK-MB is unavailable, total CK may be used |
Thrombolysis in MI (TIMI) major bleeding | Intracerebral hemorrhage, or any bleeding associated with a >5 g/dL fall in hemoglobin or a 15% absolute decrease in hematocrit* |
TIMI minor bleeding | Any bleeding event associated with a >3 g/dL fall in hemoglobin or a 10% absolute decline in hematocrit, or a >4 g/dL fall in hemoglobin or a 12% absolute decline in hematocrit in the absence of overt bleeding* |
Major bleeding (REPLACE-2 definition) | Intracerebral hemorrhage or any bleeding event associated with a >3 g/dL fall in hemoglobin, or a >4 g/dL fall in hemoglobin in the absence of overt bleeding, or any red cell transfusion of 2 or more units* |
GUSTO (Global Use of Strategies to Open Occluded Coronary Arteries) severe or life-threatening bleeding | Intracerebral hemorrhage or bleeding that causes hemodynamic compromise or requires intervention |
GUSTO minor bleeding | Bleeding that requires transfusion but does not cause hemodynamic compromise |
* All calculations of falls in hemoglobin are adjusted for any transfusion by the Landefeld index.
Monitoring of Anticoagulation
Various assays, including the activated clotting time (ACT), ecarin clotting time (ECT), and FXa levels have been used to monitor the therapeutic effect of anticoagulants during PCI. However, the correlation between the levels achieved with these assays with various agents and clinical events have only been studied retrospectively. Furthermore, the relationship between the assay level achieved and clinical events is also influenced by the concomitant anti-platelet therapy. Hence, in the context of unfractionated heparin therapy, increasing levels of ACT are associated with a modest reduction in peri-procedural ischemic events but a moderate increase in bleeding events.5 In contrast, when heparin is given with abciximab, ischemic events are fewer; there is little further reduction in events at higher ACT levels but a substantial increase in bleeding events occurs (Fig. 9-5). The ACT assay is not as useful for monitoring the efficacy of enoxaparin and the other low-molecular-weight heparins (LMWHs), with lesser degrees of prolongation observed in this assay.6 Traditional laboratory-based FXa assays also remain impractical for cath-lab use. The ENOX assay (Rapidpoint) is a whole-blood, point-of-care assay that correlates with laboratory enoxaparin-induced anti-FXa levels.7 The Evaluating Enoxaparin Clotting times (ELECT) study explored the relationship between the ENOX assay results and clinical outcomes among 445 patients receiving subcutaneous enoxaparin, intravenous enoxaparin, or both prior to PCI.8 There was a nonsignificant and nonlinear association between the ENOX times and ischemic complications, whereas bleeding events increased with greater ENOX times. ENOX times of between 250 and 450 seconds (correlating with anti-FXa levels of between 0.8 and 1.8 international units per milliliters [IU/mL]) for intra-procedural anticoagulation and levels of less than 200 seconds to 250 seconds for sheath removal have been recommended when enoxaparin is used. Similarly, the Hemonox, another point-of-care test of anti-FXa levels, appears to be able to detect suboptimal levels of anti-FXa (<0.5 IU/ml) with modest sensitivity.9,10 The clinical usefulness of these tests remains to be determined, and they are not in routine clinical use at this time. In contrast to both heparin and LMWH, bivalirudin is generally associated with greater prolongation of ACT. This effect appears to occur in a dose-dependent manner, though no gradient of benefit with respect to ischemic or bleeding events has been observed across the range of ACT values recorded at the doses studied in clinical trials.11 Furthermore, despite the higher ACT levels, lower rates of bleeding have been consistently observed with bivalirudin, highlighting the limited value of ACT in predicting clinical events with this agent. Hence, ACT provides qualitative but not quantitative information about bivalirudin and is only of value in determining if this agent was effectively administered. As a possible clinical alternative, the monitoring of these agents with the ECT may be more appropriate. Measurements based on this test appear to better correlate with plasma bivalirudin and hirudin levels.12 Whether the levels based on this assay relate to clinical events and evolve to recommended targets for therapy remains to be established.
Unfractionated Heparin
Clinical Data with Unfractionated Heparin
Worldwide, unfractionated heparin remains the mainstay anticoagulant for patients undergoing PCI. Despite this fact, there are no prospective randomized data to demonstrate the efficacy of this agent compared with placebo, and current dosing recommendations are empiric. Nevertheless, clinical experience and anecdotal evidence clearly demonstrate the need for some degree of anticoagulation in the setting of balloon-induced and stent-induced vascular injuries. In the absence of prospective randomized data, several studies point toward the benefits and risks associated with greater degrees of anticoagulation with heparin in PCI. Early case control studies in the era of PTCA suggest that patients experiencing acute vessel closure and death or urgent revascularization had lower ACT levels than those not experiencing these complications. Similarly, among 403 patients randomized to either intravenous (IV) heparin 5000 units or 20,000 units IV before balloon angioplasty, those receiving the higher dose experienced a nonsignificant reduction in the rates of death, MI, acute vessel closure, and repeat interventions (8.0% vs. 12.5%, P = ns [not significant]) but an increased rate of bleeding complications (20% vs. 6%, P < 0.001).13 Weight-adjusted dosing has been studied as a strategy to reduce the variability in dose response. In a 400-patient randomized trial assessing weight-adjusted dosing compared with higher fixed dosing, the weight-adjusted dosing strategy was not associated with superior efficacy or safety, though earlier sheath removal was made possible. Nevertheless, a pooled analysis of data from patients treated with heparin only in several randomized clinical trials suggested that there is a gradient of benefit associated with increasing degrees of anticoagulation with commensurate risk of bleeding events. This analysis suggested that ACT levels in excess of 350 seconds are associated with fewer ischemic events, though bleeding rates also increase at these levels.5 Such levels of anticoagulation are not required when concomitant GP IIb/IIIa inhibition is used, and the relevance of these data in the context of pretreatment with thienopyridines is not known.14 These observations have also been difficult to demonstrate in smaller studies where the initial heparin doses and, therefore, the ACT levels achieved were lower. In contrast, available data do not support the use of prolonged heparin infusions following PCI for the prevention of subacute ischemic events, where no significant reduction in ischemic events is observed but there is a clear excess in bleeding events and increased length of hospital stay. This is especially true for patients receiving GP IIb/IIIa inhibition.
Low-Molecular-Weight Heparin
Pharmacology
The LMWHs are produced by chemical or enzymatic depolymerization of unfractionated heparin resulting in heparin fragments with a mean molecular weight that is approximately 30% of most unfractionated heparin preparations. However, the molecular sizes of heparin molecules still vary, and therefore anticoagulant characteristics remain heterogeneous, though more predictable, when compared with heparin. The principal effect of the LMWHs is the inhibition of anti-FXa via anti-thrombin. In comparison with unfractionated heparin, the LMWHs demonstrate a more consistent dose response as well as less platelet activation; they also demonstrate platelet factor 4 interactions that lead to less HIT. LMWHs have a longer half-life compared with unfractionated heparin. Clearance is by renal excretion, however, and the biologic half-life is increased in patients with renal failure (Table 9-2). Several small studies have explored the various dosing strategies for the use of enoxaparin in PCI. Adequate levels of anti-FXa were observed among patients 2 to 8 hours following subcutaneous dosing of enoxaparin 1 mg/kg twice per day and among those receiving an additional 0.3 mg/kg intravenous dose 8 to 12 hours following subcutaneous dosing at 1.0 mg/kg.15 Other investigators have suggested that doses as low as 0.5 mg/kg of IV enoxaparin may be safe and efficacious and enable easier sheath management, though a quarter of the patients in this study also received a GP IIb/IIIa inhibitor. Some evidence suggests that enoxaparin may be reversed by the intravenous administration of protamine, but these data are limited.
Clinical Data with Enoxaparin
Among the available LMWHs, the majority of the data supports the use of enoxaparin in PCI. The initial reported experience with enoxaparin, specifically in PCI, includes a series of studies performed by the National Investigators Collaborating on Enoxaparin (NICE) study group. These studies explored enoxaparin without abciximab (NICE-1) and with abciximab (NICE-4) among patients undergoing PCI and compared these historically with the arms of the EPILOG and EPSITENT trials, respectively (Fig. 9-6
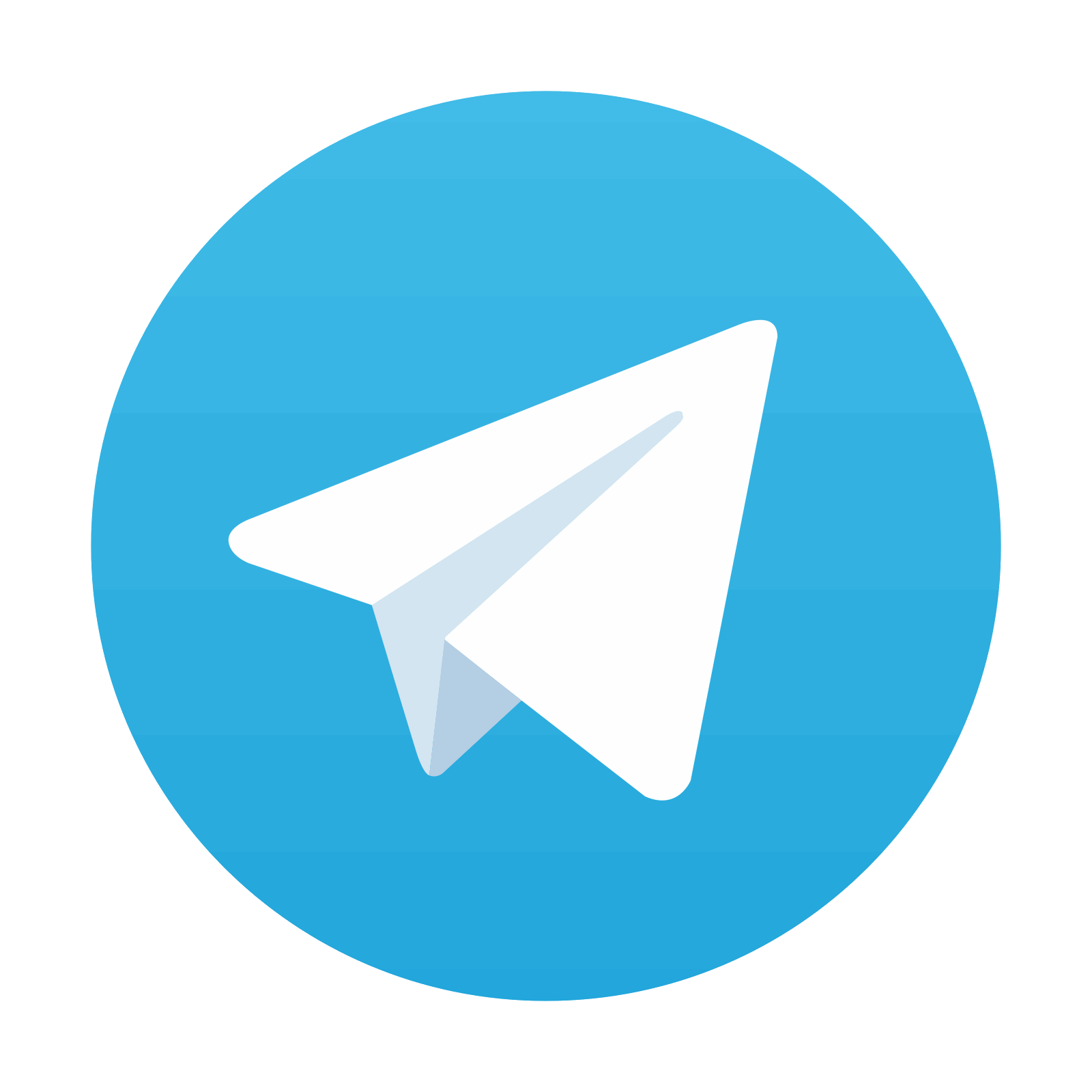
Stay updated, free articles. Join our Telegram channel

Full access? Get Clinical Tree
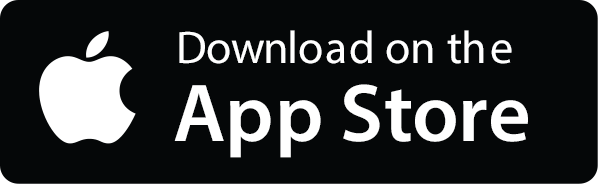
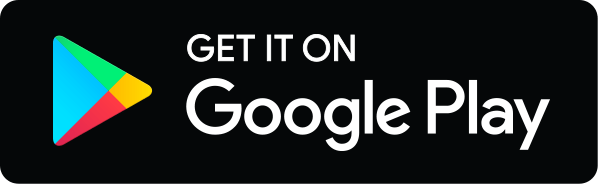