Airways
Clinical Indications
Computed tomography (CT) remains the most accurate noninvasive means for evaluating the airways and is of established value for imaging virtually the entire gamut of diseases that affect both large and small airways (1). In fact, the most common indications generally fall into one of three broad categories: (a) patients in whom occult disease is suspected—included in this category are symptomatic patients, presenting with chronic cough, localized wheezing, dyspnea, or hemoptysis, especially with normal or nonlocalizing radiographs (2, 3, 4, 5); (b) patients with radiographic abnormalities for whom better anatomic delineation is required—included in this category are patients with radiographic evidence of chronic infiltrates and/or atelectasis (6) or diffuse parenchymal disease in which identification of airway abnormalities is pertinent (e.g., sarcoidosis) (7); and (c) candidates for interventional bronchoscopic procedures—included in this category are patients for whom an ever-increasing number of methods for diagnosing and especially treating endobronchial abnormalities are now available for which precise preprocedural anatomic correlation is of value (8, 9, 10, 11, 12). In addition to these broad indications, CT also frequently plays a critical role in the follow-up of patients undergoing treatment for the entire gamut of diseases affecting the airways.
Technique
The introduction of multidetector computed tomography (MDCT) has profoundly altered our approach to scanning the airways (13,14). The ability to acquire contiguous and/or overlapping high-resolution 0.5-mm to 1- to 2-mm-thick sections throughout the thorax in a single breath-hold enables even the smallest airways to be optimally visualized (Fig. 5-1). With this approach it is now possible with newer scanners to routinely reconstruct at a minimum three prospective datasets, for example, 5-mm sections every 5 mm using standard lung and mediastinal reconstruction algorithms, as well as an additional high-resolution dataset with 1-mm sections reconstructed every 10 mm using an appropriate high-resolution reconstruction algorithm. Following this “generic” approach, all studies in effect become a combination of routine and high-resolution examinations resulting in improved detection of airway lesions, especially when compared with conventional CT techniques (15). First, although never evaluated in a prospective randomized fashion, in our experience, the availability of high-resolution
images frequently discloses otherwise unsuspected airway disease, especially involving the peripheral airways. Second, in cases in which additional anatomic resolution is needed, the ability to retrospectively reconstruct select images through regions of interest without the need to rescan patients frequently improves diagnostic certainty (Fig. 5-1). In this regard, it is also now possible to obtain high-quality multiplanar and/or volumetric reconstructions through the whole lung, providing a more global assessment of the extent and severity of disease—techniques of potential value in patients with diffuse airway inflammation, in particular (Fig. 5-2).
images frequently discloses otherwise unsuspected airway disease, especially involving the peripheral airways. Second, in cases in which additional anatomic resolution is needed, the ability to retrospectively reconstruct select images through regions of interest without the need to rescan patients frequently improves diagnostic certainty (Fig. 5-1). In this regard, it is also now possible to obtain high-quality multiplanar and/or volumetric reconstructions through the whole lung, providing a more global assessment of the extent and severity of disease—techniques of potential value in patients with diffuse airway inflammation, in particular (Fig. 5-2).
These advantages must be weighed against a number of potential disadvantages. These include added reconstruction and postprocessing times, as well as storage requirements for potentially several hundred additional images. Of greater concern is the potential of added risk resulting from increased radiation exposure. This problem can be minimized by routinely using reduced milliamperage whenever feasible, especially in younger patients,
women, and patients for whom multiple follow-up studies are planned. Diagnostic images can almost always be obtained using between 80 and 100 mAs and frequently as low as 40 mAs in thin patients (16,17). As shown by Yi et al. (18), no significant loss of diagnostic accuracy can be identified when evaluating patients with bronchiectasis with tube current setting as low as 70 mA.
women, and patients for whom multiple follow-up studies are planned. Diagnostic images can almost always be obtained using between 80 and 100 mAs and frequently as low as 40 mAs in thin patients (16,17). As shown by Yi et al. (18), no significant loss of diagnostic accuracy can be identified when evaluating patients with bronchiectasis with tube current setting as low as 70 mA.
Although volumetric high-resolution CT (HRCT) has been reported to result in a considerably greater radiation dose (19), use of low-dose scanning coupled with dose modulation techniques available on newer CT scanners can be employed to minimize this problem. One solution is to initially perform volumetric data acquisition, with subsequent follow-up examinations performed with dedicated high-resolution images only using low-dose technique, whenever feasible, to minimize subsequent radiation exposure.
Another approach of potential value, specifically in patients with suspected bronchiectasis, is to utilize 3-mm rather than 1-mm sections. As shown by Remy-Jardin et al. (20), no difference in accuracy or estimation of disease extent or severity could be identified when 1-mm and 3-mm sections were compared, leading these authors to conclude that the use of scans generated by 4 × 2.5 mm collimation in place of 4 × 1 mm collimation could allow as great as 20% decrease in radiation dose without sacrificing diagnostic accuracy. It should be emphasized, however, that sections thicker than 3 mm are suboptimal for evaluating bronchiectasis and should never be relied on to make this diagnosis (Fig. 5-3) (21).
Scans are routinely performed in deep inspiration with the patient supine. Expiratory images may prove useful to either detect or confirm a suspicion of air
trapping (Fig. 5-4) (22, 23, 24, 25). Expiratory HRCT scans may be obtained either during suspended respiration after forced exhalation (static expiratory CT) or during forced exhalation (dynamic expiratory CT) (26,27). Expiratory scans may be obtained at selected levels, typically 3 to 5, or through the entire lung using volumetric technique.
trapping (Fig. 5-4) (22, 23, 24, 25). Expiratory HRCT scans may be obtained either during suspended respiration after forced exhalation (static expiratory CT) or during forced exhalation (dynamic expiratory CT) (26,27). Expiratory scans may be obtained at selected levels, typically 3 to 5, or through the entire lung using volumetric technique.
Although assessment of the central airways may be accomplished using a wide range of window settings, visualization of smaller peripheral airways, as well as identification of subtle focal air trapping, is dependent on the appropriate choice of window levels and widths (Fig. 5-5). In one study using inflation-fixed lungs imaged with 1.5 mm high-resolution technique, optimal evaluation of peripheral bronchial walls requires the use of window centers between 2250 and 2700 HU, with corresponding window widths between 1,000 and 1,400 HU, respectively (28).
Although visualization of the airways per se rarely requires administration of intravenous contrast material, in cases in which atelectasis is identified on corresponding radiographs, the use of a bolus of contrast media is
recommended when there is a suspicion of mediastinal disease or to facilitate differentiation between central tumor and peripheral consolidated or atelectatic lung (Fig. 5-6).
recommended when there is a suspicion of mediastinal disease or to facilitate differentiation between central tumor and peripheral consolidated or atelectatic lung (Fig. 5-6).
Advanced Reconstruction Techniques
Although routine axial images remain the standard for identifying airway disease, this method has important limitations, including (a) inability in most cases to identify mucosal disease; (b) limited accuracy in identifying submucosal disease, especially central extension of neoplasm; (c) limited accuracy in the estimation of the length of tracheal and bronchial stenoses; and (d) limited assessment of obliquely coursing airways. Endobronchial lung cancers, even those located in the central airways, can be missed on conventional CT, particularly when thick 7- to 10-mm sections only are acquired.
With the introduction of MDCT, various high-quality reconstruction techniques have become routinely available
(29, 30, 31). These include multiplanar reconstructions (MPRs), maximum intensity projections (MIPs) and minimum intensity projections (MinIPs), external rendering with either three-dimensional (3D) shaded surface displays (SSDs) or volumetric rendering, and internal rendering, or so-called virtual bronchoscopy (VB) (Fig. 5-7). Of these various techniques, the most useful, to date, have been MPRs (32, 33, 34), especially useful for assessing airway stenoses, particularly those after prolonged tracheal intubation or lung transplantation (35). Although less commonly employed, MIPs have proved of value for assessing bronchiolar disease, especially for patients with so-called tree-in-bud opacities (Fig. 5-2). Least often used in our experience, although of considerable promise, is VB.
(29, 30, 31). These include multiplanar reconstructions (MPRs), maximum intensity projections (MIPs) and minimum intensity projections (MinIPs), external rendering with either three-dimensional (3D) shaded surface displays (SSDs) or volumetric rendering, and internal rendering, or so-called virtual bronchoscopy (VB) (Fig. 5-7). Of these various techniques, the most useful, to date, have been MPRs (32, 33, 34), especially useful for assessing airway stenoses, particularly those after prolonged tracheal intubation or lung transplantation (35). Although less commonly employed, MIPs have proved of value for assessing bronchiolar disease, especially for patients with so-called tree-in-bud opacities (Fig. 5-2). Least often used in our experience, although of considerable promise, is VB.
To date, numerous reports have shown that VB is a reliable means for displaying the central airways, comparable to routine fiberoptic bronchoscopy (FB) in detecting obstructing endobronchial lesions or focal airway stenoses (29,30,36, 37, 38, 39, 40, 41, 42). However, despite these studies, VB has rarely proved of real diagnostic value. One exception is the use of VB in guiding ultrathin bronchoscopy (Fig. 5-8) (43,44). An additional new application for which VB is essential is electromagnetic catheter navigation (12). This technique requires correlating anatomic reference points identified on virtual bronchoscopic images with those obtained at the time of endoscopy. Although not widely available at this time, it may be anticipated that as this technique gains validation it will emerge as a standard method for diagnosing especially peripheral lung lesions otherwise too difficult to biopsy using standard bronchoscopic technique.
![]() Figure 5-8 Virtual bronchoscopic CT-guided ultrathin bronchoscopy. See Color Figure 5-8I–P. A–H: Select magnified axial (A–D) and corresponding sagittal (E–H) images beginning at the secondary carina and proceeding caudally in a patient with a left lower lobe nodule. I–L: Virtual bronchoscopic views corresponding to the axial and sagittal views shown in A to H. M–P: Bronchoscopic views obtained using ultrathin bronchoscopy. These correspond precisely with the virtual bronchoscopic views at the same levels shown in I to L. Q: CT scanogram obtained at the time of bronchoscopy showing the extremely peripheral position of the ultrathin bronchoscope. R: Magnified view of the left lower lobe showing the tip of the ultrathin bronchoscope within the left lower lobe nodule (arrow). These images confirm that virtual bronchoscopy may be indispensable by providing a road map to guide ultrathin bronchoscopes into regions of the lung previously difficult to access by bronchoscopists. (From Naidich DP, Webb WR, Grenier PA, et al. Imaging of the airways: functional and radiologic correlations. Philadelphia: Lippincott Williams & Wilkins; 2005 , with permission.) |
Normal Airway Anatomy
Trachea and Mainstem Bronchi
Anatomy
The trachea is a cartilaginous and fibromuscular tube extending from the inferior aspect of the cricoid cartilage (at the level of the sixth cervical vertebra) to the carina (at the level of the fifth thoracic vertebra). It measures from 10 to 12 cm in length (45,46). The trachea is divided into extrathoracic and intrathoracic portions; the trachea can be considered intrathoracic at the point at which it passes posterior to the manubrium. In a CT study of 50 anatomically normal persons, the extrathoracic trachea measured 2 to 4 cm in length, whereas the intrathoracic trachea measured 6 to 9 cm in length (mean, 7.5±0.8 cm).
![]() Figure 5-9 Normal tracheal anatomy. See Color Figure 5-9B. A: Cross-sectional drawing shows typical appearance of a horseshoe-shaped tracheal cartilage within the anterior and lateral tracheal wall, which is markedly thicker than the posterior tracheal membrane. Although labeled, tracheal mucosa and submucosa are rarely identifiable with CT. B: Histopathologic section shows characteristic horseshoe-shaped cartilage as dark. The posterior tracheal membrane is bowed anteriorly. (From Webb EM, Elicker BM, Webb WR. Using CT to diagnose nonneoplastic tracheal abnormalities: appearance of the tracheal wall. AJR Am J Roentgenol. 2000;174:1315–1321 , with permission.) |
The trachea contains 16 to 22 cartilaginous “rings,” which are in fact horseshoe shaped and incomplete posteriorly (Fig. 5-9). These help to support the tracheal wall and to maintain an adequate tracheal lumen during forced expiration. The posterior portion of the tracheal wall, lying
between the open ends of the tracheal cartilages, is a thin fibromuscular membrane termed the posterior tracheal membrane (47) (Fig. 5-9). The blood supply to the trachea is bilateral and tenuous (48). Superiorly, blood is supplied from branches of the inferior thyroid arteries and the extreme right intercostal artery, whereas inferiorly, branches of the bronchial arteries and the third intercostal artery supply the trachea.
between the open ends of the tracheal cartilages, is a thin fibromuscular membrane termed the posterior tracheal membrane (47) (Fig. 5-9). The blood supply to the trachea is bilateral and tenuous (48). Superiorly, blood is supplied from branches of the inferior thyroid arteries and the extreme right intercostal artery, whereas inferiorly, branches of the bronchial arteries and the third intercostal artery supply the trachea.
There is marked variability in the cross-sectional appearance of the trachea, which may appear rounded, oval, or horseshoe shaped (Fig. 5-10) (49). The posterior tracheal membrane may appear convex posteriorly, flat, or convex anteriorly (35,38). In one study of anatomically normal individuals, the trachea most often appeared round or oval, with a horseshoe-shaped trachea with a flat posterior membrane noted in nearly 25% (45).
Tracheal diameter can vary widely in anatomically normal persons. In men, tracheal diameter averages 19.5 mm and ranges from 13 to 25 mm (mean,±3 standard deviations) in the coronal plane and 13 to 27 mm in the sagittal plane (49). In women, tracheal diameter is slightly less, averaging 17.5 mm and ranging from 10 to 21 mm in the coronal plane and 10 to 23 mm in the sagittal plane (49). In children, tracheal diameter increases as the child grows, and tracheal cross-sectional area correlates most closely with height (50,51). In children, variation in tracheal dimensions at different levels is
as much as 20% (50,51).
as much as 20% (50,51).
The shape of the intrathoracic trachea (as well as the mainstem and lobar bronchi) can change dramatically with expiration or dynamic respiratory maneuvers (Figs. 5-4 and 5-11). Most commonly, invagination of the posterior membrane occurs with forced expiration, resulting in a crescent-moon or horseshoe-shaped lumen (27). Marked anterior bowing of the posterior tracheal membrane can be used on expiratory CT as a marker of forced expiration. It may also be of value when assessing the effectiveness of a patient’s expiratory effort when attempting to diagnose tracheobronchomalacia or peripheral air trapping. Slight side-to-side narrowing of the trachea also occurs during expiration, a finding accentuated in the presence of tracheomalacia. In a study of dynamic CT during forced expiration, the sagittal tracheal diameter decreased an average of 32%, from 19.6±2.3 to 13.3±3.5 mm. In the coronal plane, mean tracheal diameter decreased 13%, from 19.4±2.7 to 16.9±2.6 mm during forced expiration (27). The extrathoracic trachea can increase in diameter slightly during forced expiration or during a Valsalva maneuver.
Lobar and Segmental Bronchi
Similar to the trachea, the bronchi are composed of both cartilaginous and fibromuscular elements. However, the distinction between those parts of the bronchial lumen that are supported by cartilage and those that are not is less clear cut than in the trachea. For a short distance, the main bronchi contain horseshoe-shaped cartilage plates, as does the trachea, but the cartilage plates become less regularly shaped at more peripheral levels. On dynamic expiratory scans, some posterior invagination of the walls of the central bronchi is often visible on CT, as in the trachea, reflecting this anatomy.
Airways divide by dichotomous branching, with approximately 23 generations of branches from the trachea to the alveoli. The wall thickness of conducting bronchi and bronchioles is approximately proportional to their diameter, at least for bronchi distal to the segmental level. In general, the thickness of the wall of a bronchus or bronchiole less than 5 mm in diameter should measure 1/6 to 1/10 of its diameter (Table 5-1) (52). Because a bronchus is usually recognized only when its walls are visible, one cannot see bronchi with a wall thickness of less than 300 μm on CT or HRCT. As a consequence, normal bronchi less than 2 mm in diameter or closer than 2 cm from pleural surfaces equivalent to seventh to ninth order airways are generally below the resolution even of high-resolution CT unless they happen to course in precisely the same plane as the CT section (Table 5-1) (53,54).
Accurate evaluation of the airways necessitates a detailed knowledge of both normal anatomy and variants (Fig. 5-12 and Table 5-2). Identification of individual airways is a function of their size and orientation, as well as recognition of the spurs that demarcate their origins. Spurs appear either as triangular wedges or as a linear septum dividing airways when seen longitudinally. Alternatively, spurs may appear as only faint curvilinear densities when sectioned horizontally. Recognizing the normal appearance of spurs not only allows confident identification of individual airways, but also, as significantly, serves as an important indication of pathology when abnormally thickened (55).
Bronchial Nomenclature
Nomenclature for describing segmental lung anatomy has undergone several changes, but that described by Jackson and Huber in 1943 (56) has been generally adopted. Bronchi are also designated using a numeric system
popularized by Boyden (57), in which segments are designated by B followed by a number (e.g., B1) and subsegments are indicated by the segmental number followed by a small letter (e.g., B1a) (Fig. 5-12). The numbering of segments is roughly in their order of origin from the airway as one proceeds distally from the carina. Standard nomenclature and 1961 revisions in letter-number codes are used in this book (Table 5-2) (58,59).
popularized by Boyden (57), in which segments are designated by B followed by a number (e.g., B1) and subsegments are indicated by the segmental number followed by a small letter (e.g., B1a) (Fig. 5-12). The numbering of segments is roughly in their order of origin from the airway as one proceeds distally from the carina. Standard nomenclature and 1961 revisions in letter-number codes are used in this book (Table 5-2) (58,59).
Table 5-1 Airway Dimensions | ||||||||||||||||||||||||||||||||||||||||
---|---|---|---|---|---|---|---|---|---|---|---|---|---|---|---|---|---|---|---|---|---|---|---|---|---|---|---|---|---|---|---|---|---|---|---|---|---|---|---|---|
|
Right-Sided Bronchial Anatomy
The right and left bronchial trees are considered independently. Emphasis is placed on recognition of characteristic axial sections (Fig. 5-13). Some variability in the cross-sectional appearance of the airways is to be anticipated; this often is the result of variation in the relationship of bronchi to the plane of the scan rather than true anatomic variation. It is also easy to distort the appearance of a normal airway if the section thickness exceeds the width of the airway, hence the rationale for being able to retrospectively reconstruct high-resolution sections through select airways as needed. Finally, there is considerable variation observed in the branching patterns of subsegmental bronchi, an additional cause of potential confusion unless care is taken to trace these airways centrally toward the carina (Table 5-2).
![]() Figure 5-12 Anatomic drawing illustrates terminology of the central airways. (From Prakash UBS. Bronchoscopy. New York: Raven Press; 1994 , with permission.) |
On the right side there are six characteristic sections that serve as convenient, easily recognizable landmarks (Fig. 5-13). These include (a) the distal trachea/carina and apical segment bronchus; (b) the right upper lobe bronchus including the anterior and posterior segmental bronchi; (c) the bronchus intermedius; (d) the origins of the middle and right lower lobe bronchi, often including the origin and proximal portion of the superior segmental bronchus; (e) the trunk of the proximal basilar bronchi, the “truncus basalis”; and (f) the peripheral basilar lower lobe bronchi. The right upper lobe bronchus is termed eparterial because it arises above the right main pulmonary artery. It has a horizontal course usually originating just below the carina. Air characteristically marginates the posterior wall of the right upper lobe bronchus as well as the posterior wall of the bronchus intermedius. Less commonly, an aberrant segmental vein can be identified posterior to the bronchus intermedius draining into the right superior pulmonary vein, most often originating from the posterior segment of the right upper lobe and less frequently from the superior segment of the right lower lobe (60). Unlike the right upper lobe anterior and posterior segmental bronchi that lie in the same plane as the CT section, the apical segmental bronchus courses superiorly and can be identified as a lucency superimposed on the distal end of the right upper lobe bronchus.
Unlike the right upper lobe bronchus, the middle lobe bronchus has an oblique course as it originates at the distal end of the bronchus intermedius (Fig. 5-13). It is easily distinguished from the origin of the right lower lobe bronchus
by identifying the lobe spur. As it courses obliquely, the distal aspect of the middle lobe bronchus appears to taper, giving it a narrowed, triangular appearance. This should not be misinterpreted as abnormal. The medial and lateral segmental bronchi arise from the distal middle lobe bronchus and are generally easily recognized on sequential thin sections. Typically, the superior segmental bronchus of the right lower lobe also can be identified at this level originating from the posterior aspect of the right lower lobe bronchus. Similar to the right upper lobe bronchus, the superior segmental bronchus also courses horizontally and is generally well seen along its entire length.
by identifying the lobe spur. As it courses obliquely, the distal aspect of the middle lobe bronchus appears to taper, giving it a narrowed, triangular appearance. This should not be misinterpreted as abnormal. The medial and lateral segmental bronchi arise from the distal middle lobe bronchus and are generally easily recognized on sequential thin sections. Typically, the superior segmental bronchus of the right lower lobe also can be identified at this level originating from the posterior aspect of the right lower lobe bronchus. Similar to the right upper lobe bronchus, the superior segmental bronchus also courses horizontally and is generally well seen along its entire length.
Table 5-2 Segmental and Subsegmental Bronchi: Nomenclature and Variants | ||||||||||||||||||||||||||||||||||||||||||||||||||||||||||||||||||||||||||||||||||||||||||||||||||||||||||||||||||||||||||||||||||||||||||||||||||||||||||||||||||
---|---|---|---|---|---|---|---|---|---|---|---|---|---|---|---|---|---|---|---|---|---|---|---|---|---|---|---|---|---|---|---|---|---|---|---|---|---|---|---|---|---|---|---|---|---|---|---|---|---|---|---|---|---|---|---|---|---|---|---|---|---|---|---|---|---|---|---|---|---|---|---|---|---|---|---|---|---|---|---|---|---|---|---|---|---|---|---|---|---|---|---|---|---|---|---|---|---|---|---|---|---|---|---|---|---|---|---|---|---|---|---|---|---|---|---|---|---|---|---|---|---|---|---|---|---|---|---|---|---|---|---|---|---|---|---|---|---|---|---|---|---|---|---|---|---|---|---|---|---|---|---|---|---|---|---|---|---|---|---|---|---|---|
|
![]() Figure 5-13 Characteristic cross-sectional anatomy of the airways. A, B: Diagrammatic illustrations of the right and left bronchial trees, respectively, each showing six characteristic axial sections illustrated in C to L. C–L: Axial sections corresponding to the diagrammatic representations in A and B (see Table 5-2 for correlation). Tr, trachea; Ap Seg (B1), right upper lobe apical segmental bronchus; Ap P (B1 + 2), left upper lobe apical-posterior segmental bronchus; Ant Seg (B3), right upper lobe anterior segmental bronchus; RULB, right upper lobe bronchus; Post Seg (B2), right upper lobe posterior segmental bronchus; BI, bronchus intermedius; LMB, left mainstem bronchus; RMB, right mainstem bronchus; Ant Seg Br (B3), left upper lobe anterior segmental bronchus; Ap-Post (B1 + 2), left upper lobe apical posterior segmental bronchus; LULB, left upper lobe bronchus; LB, lingual bronchus; LLLB, left lower lobe bronchus; MLB, middle lobe bronchus; RLLB, right lower lobe bronchus; Inf LB (B5), inferior lingular bronchus; LLLB, left lower lobe bronchus; Sup LB (B4), superior lingular bronchus; Sup Seg (B6), left lower lobe superior segmental bronchus; Sup Seg Br (B6), right lower lobe superior segmental bronchus; Lat Seg Br (B4), middle lobe lateral segmental bronchus; Med Seg Br (B5), middle lobe medial segmental bronchus; TB-RLL, truncus basalis, right lower lobe; TB-LLL, truncus basialis, left lower lobe; AB-RLL (B8), right lower lobe anterobasilar segmental bronchus; MB-RLL (B7), right lower lobe medial basilar segmental bronchus; LB-RLL (B9), right lower lobe lateral basilar segmental bronchus; PB-RLL (B10), right lower lobe posterobasilar segmental bronchus; AB-LLL (B8), left lower lobe anterobasilar segmental bronchus; MB-LLL (B7), left lower lobe medial basilar segmental bronchus; LB-LLL (B9), left lower lobe lateral basilar segmental bronchus; PB-LLL (B10), left lower lobe posterobasilar segmental bronchus. (From Naidich DP, Webb WR, Grenier PA, et al. Imaging of the airways: functional and radiologic correlations. Philadelphia: Lippincott Williams & Wilkins; 2005 , with permission.) |
Left-Sided Bronchial Anatomy
Similar to right-sided bronchial anatomy, there are also six characteristic axial sections that can routinely be identified through the left-sided airways (Fig. 5-13). These include (a) the distal trachea/carina and apical-posterior segmental bronchus of the left upper lobe, (b) the left mainstem bronchus and proximal apical-posterior segmental bronchus, (c) the distal left mainstem bronchus/proximal left upper lobe bronchus, (d) the distal left upper lobe bronchus and proximal lingular bronchus, (e) the trunk of the proximal left lower lobe bronchus—the “truncus basalis,” and (f) the proximal basilar segmental bronchi.
Among these characteristic sections, the most complex anatomically are those through the left upper lobe bronchus. Because of its complexity, it is possible to obtain multiple sections through this same airway. As a consequence, the appearance of the left upper lobe bronchus and of its branches is best described at two specific levels (corresponding to the third and fourth characteristic sections described previously) (Fig. 5-13): through the upper portion of the left upper lobe bronchus, which includes the origin of the apical-posterior segmental bronchus, and through the lower portion of the left upper lobe bronchus, which includes the lingula bronchus, respectively.
The key to identifying a section through the upper aspect of the left upper lobe bronchus is to note that the posterior wall of the bronchus at this level is smoothly concave and is further marked by a band of hazy increased density coursing across the left upper lobe bronchus caused by a partial volume effect from the left main pulmonary artery as it passes over the left upper lobe bronchus. At this point, the origin of the apical-posterior segmental bronchus can be identified as a circular lucency superimposed on the distal aspect of the upper portion of the left upper lobe bronchus.
In distinction, a section obtained through the lower portion of the left upper lobe bronchus will also include the secondary carina marking the origin of the left lower lobe bronchus, identifiable as a triangular wedge of soft tissue located posterolaterally. At this level the left interlobar pulmonary artery lies lateral to both the left upper and lower lobe bronchi and typically has a smooth, rounded contour laterally. A circular lucency at the end of the left upper lobe bronchus at this level indicates the origin of the lingular bronchus. The superior segmental bronchus is also often seen coursing posteriorly at or near this level. Unlike the superior segmental bronchus, however, the anterior segmental bronchus to the left upper lobe is more unpredictable: most often arising as a trifurcation from the distal end of the left upper lobe bronchus, this same airway may also arise from the apical-posterior segmental bronchus or even the proximal lingular bronchus. Despite this, the anterior segmental bronchus is usually easily identified as it characteristically courses horizontally in the same plane as the CT scan. Regarding the basilar airways, the only difference between the right and left sides is that on the left, the medial and anterobasilar segmental bronchi most often arise from a common trunk. Otherwise, the right and left lower lobe bronchi tend to be mirror images of each other.
Anatomic Variants
In adults the most commonly encountered lung bud anomalies are either anomalous or supernumerary bronchi (61). Additional anomalies, including agenesis, congenital bronchial atresia (Fig. 5-14), congenital lobar emphysema, congenital cystic adenomatoid malformation, and bronchogenic cysts, may also be seen, although
less commonly. By definition, a displaced or anomalous bronchus is one that arises at a lower level than normal in the bronchial tree. If this same bronchus also supplies the same lung segment, it is more aptly labeled supernumerary. Although several hypotheses have been advanced to account for these anomalies (62), as proposed by Wu et al. (63), the following slightly modified classification has proved especially clinically useful.
less commonly. By definition, a displaced or anomalous bronchus is one that arises at a lower level than normal in the bronchial tree. If this same bronchus also supplies the same lung segment, it is more aptly labeled supernumerary. Although several hypotheses have been advanced to account for these anomalies (62), as proposed by Wu et al. (63), the following slightly modified classification has proved especially clinically useful.
Anomalies arising from normal higher order bronchial divisions. This includes accessory superior segmental bronchi, for example, identifiable as two parallel
bronchi both supplying the superior segment of the right lower lobe, and axillary bronchi, identifiable as a supernumerary segmental bronchus supplying the lateral aspect of the right upper lobe.
Anomalies arising from sites typically lacking branches. Included in this group are tracheal bronchi, accessory cardiac bronchi, and bridging bronchi (Figs. 5-15 and 5-16). The most common of these is the tracheal or pig bronchus (Fig. 5-15) (64, 65, 66, 67). Present in up to 1% to 2% of normal individuals, tracheal bronchi exclusively arise on the right side and may involve displacement of the entire right upper lobe, the apical segmental bronchus only, or additional supernumerary lobar or separate apical segmental bronchi (68). Although this anomaly may superficially mimic the rarer entity of tracheal diverticula endoscopically, CT readily distinguishes between these entities (Fig. 5-17) (69). Tracheal bronchi also need to be distinguished from tracheoceles (Fig. 5-18). Resulting from foci of muscular weakness within the trachealis muscle, tracheoceles have been divided into two groups: those with wide mouths, likely acquired, and those with narrow mouths, likely congenital in origin. These lesions may grow to be capacious and thus may serve as reservoirs for debris, leading to dumping of their contents into the tracheobronchial tree (70,71).
Another rare congenital anomaly of the tracheobronchial tree, the accessory cardiac bronchus is the only true supernumerary anomalous bronchus (72), with an incidence of less than 0.5% in the general population (73, 74, 75). It arises from the medial wall of the bronchus intermedius before the origin of the superior segmental bronchus to the right lower lobe and usually before the origin of the middle lobe bronchus (Fig. 5-19). The bronchus is then directed caudally toward the mediastinum, hence the “cardiac” appellation. The length of the cardiac bronchus is variable, ranging from a short, blind-ending diverticulum to a longer, branching structure. The short type is usually a simple bronchial stump without associated alveolar tissue, whereas the longer subtype has been noted both with and without associated rudimentary alveolar tissue (73). Investigators have speculated that this anomaly, in fact, represents a rudimentary accessory lobe. Rarely, this blind-ending airway may serve as a potential reservoir for infectious material, leading to chronic inflammation and hypervascularity that result in recurrent episodes of aspiration pneumonitis or hemoptysis (73,74,76).
Anomalies associated with abnormalities of situs. In this category, simple reversal of the right- and left-sided airways is the most commonly encountered anomaly (Fig. 5-7). Less frequent are anomalies due to bronchial isomerism, in which the pattern of airway branching and pulmonary lobation is identical in the two lungs (63,68). This includes bilateral left-sided airway anatomy, either as an isolated finding or associated either with the venolobar syndrome or less commonly polysplenia, and bilateral right-sided airway anatomy. Right-sided airway isomerism is usually associated with asplenia and severe congenital heart disease and is only rarely seen in adults.
![]() Figure 5-17 Tracheal diverticulum. A, B: Axial and coronal multiplanar reconstructions (MPRs), respectively, show a well defined blind-ending tracheal diverticulum originating from the lateral aspect of the mid trachea. This entity should be distinguished from both anomalous bronchi, which typically occur nearer to the carina (see Fig. 5-15), and tracheoceles, which typically originate at a higher level (see Fig. 5-18). (From Naidich DP, Webb WR, Grenier PA, et al. Imaging of the airways: functional and radiologic correlations. Philadelphia: Lippincott Williams & Wilkins; 2005 , with permission.) |
Central Airways
Computed Tomography/Bronchoscopic Correlations
For purposes of analysis, the airways can be divided into central airways, extending from the trachea to the segmental bronchi, and peripheral airways, extending from the subsegmental bronchi to the bronchioles. This approach has the advantage of correlating with the approximate range of visualization of the airways by routine FB.
Along with rapid advances in CT and magnetic resonance (MR) technology, the past decade has seen remarkable advances in the field of bronchoscopy, both in the development of new devices such as ultrathin bronchoscopes or endobronchial ultrasound (EBUS) as well as sophisticated interventional bronchoscopic techniques (77,78). Accurate assessment of the relative value of
these various techniques necessitates a thorough understanding of CT-FB correlations (79). In this chapter, attention is focused on CT-FB correlations in evaluating and diagnosing central airway lesions; correlation between CT and FB in diagnosing peripheral lesions is discussed in Chapter 6.
these various techniques necessitates a thorough understanding of CT-FB correlations (79). In this chapter, attention is focused on CT-FB correlations in evaluating and diagnosing central airway lesions; correlation between CT and FB in diagnosing peripheral lesions is discussed in Chapter 6.
Principles of Interpretation
Compared with CT, bronchoscopic evaluation of the airways has numerous advantages. Most important, bronchoscopy allows direct visualization of airway lumina to the fifth generation, enabling identification of subtle mucosal, submucosal, and endobronchial abnormalities. Bronchoscopy also allows acquisition of bacteriologic, cytologic, and histologic material from endobronchial, peribronchial, and parenchymal sites with minimal risk (80). In select cases, bronchoscopy may provide localization of bleeding sites before surgery. In addition, an increasing number of therapeutic interventions may now be performed endoscopically, including traditional procedures, such as removal of mucus plugs or foreign bodies, as well as a growing number of more sophisticated applications, including the use of various stents to provide airway patency. Despite these advantages, FB has important limitations, especially for those patients without evidence of endobronchial disease in whom a specific histologic diagnosis is sought (80,81).
In comparing CT with FB, terminology appropriate for bronchoscopic evaluation may not be appropriate for CT. Descriptive terms such as mucosal or submucosal, in particular, may be misleading (Fig. 5-20) (82,83). CT evidence of smooth airway narrowing, for example, may be associated endoscopically with endobronchial, submucosal, or peribronchial disease (Fig. 5-21). In fact, as a general rule, CT is imprecise in characterizing abnormalities identified bronchoscopically (82). Exceptions include the finding of a discrete polypoid endoluminal lesion (Figs. 5-22 and 5-23) or, less commonly, calcific endobronchial filling defects, as may occur, for example, in patients with aspirated foreign bodies (Fig. 5-24) or broncholiths. Also diagnostic is the finding of a fat-containing intraluminal lesion characteristic of an endobronchial hamartoma (Fig. 5-25). In the case of broncholithiasis, CT may prove more sensitive than FB by identifying both intraluminal and peribronchial calcifications distal to inflamed and narrowed airways rendered inaccessible to bronchoscopy (84, 85, 86). As documented by Conces et al. (84) in a study of 15 patients with proved broncholithiasis, whereas CT correctly localized 6 of 10 endobronchial lesions and 4 of 5 calcified peribronchial nodes, the diagnosis of broncholithiasis was established endoscopically in only 5 cases.
CT has proved of greatest value prior to bronchoscopy by defining the precise location and extent of disease within and surrounding the central airways in patients with known or suspected lung cancer (Figs. 5-6, 5-22, 5-23, 5-26). In patients with central airway obstruction, CT often allows distinction between central tumor and peripheral atelectasis (Figs. 5-6 and 5-22) (87,88). In patients with evidence of direct invasion of adjacent mediastinal organs, CT can identify those with unresectable tumor (Figs. 5-6, 5-22, 5-26, 5-27). CT also allows visualization of distal airways that otherwise cannot be evaluated bronchoscopically because of proximal airway obstruction (Fig. 5-28). Additionally, in patients with central obstructing tumors, CT can be used to predict which patients will benefit from interventional bronchoscopic techniques, including selecting optimal sites for transbronchial needle aspiration (TBNA), identifying candidates for the placement of endobronchial stents (Figs. 5-28 and 5-29), and defining the extent of extraluminal disease in candidates for potential laser therapy, for example (89,90).
Despite these advantages, important limitations with regard to bronchoscopy have also been noted. CT is of limited value, for example, in the detection of endobronchial lesions smaller than 2 to 3 mm (Fig. 5-30). In a prospective study of 105 lesions in 98 patients with radiographically occult squamous cell carcinoma (SCC) identified on the basis of positive sputum cytology, for example, Usuda et al. (91) showed that although 55 (53%) of these lesions were larger than 2 mm (including 22 characterized as either polypoid or nodular, with the remainder appearing flat or as focal areas of bronchial irregularity), 27 lesions (26%) proved to be smaller than 2 mm, and 23 lesions (22%) were endoscopically invisible, being identified by bronchial brushings alone. From these data, it is unlikely that CT, even optimally performed, would have identified more than 20% of these lesions, rendering CT of limited value in routine screening of the central airways in patients with positive sputum cytology. It should be emphasized that, although FB can detect subtle endobronchial disease not identifiable with CT, it should not be assumed that all such lesions are significant. Even in patients presenting with hemoptysis, investigators have shown that many such lesions are nonspecific. In one prospective study evaluating both CT and FB in 57 consecutive patients presenting with hemoptysis (3), focal endobronchial lesions could be identified bronchoscopically in a total of 18 (32%) cases. Although 6 of these lesions proved to be due to bronchogenic carcinoma, all prospectively identified by CT, in the remaining 12 cases, transbronchial biopsies proved diagnostic in only 1, a patient with mucosal Kaposi sarcoma.
Transbronchial Needle Aspiration
The ability of CT to provide lymph node “mapping” to assist in the planning and performance of TBNA serves as a paradigm for the integration and correlation of CT and bronchoscopy (92). TBNA allows access to mediastinal and hilar tissue through the bronchoscope, potentially obviating more invasive procedures such as mediastinoscopy or thoracotomy. Although CT may be
indispensable by identifying optimal biopsy sites, CT may also be of value by identifying cases for which TBNA is not indicated, as it has been shown that the yield of TBNA in patients with negative CT scans is sufficiently low to obviate this procedure (92a). CT can also help to avoid potentially false-positive TBNA by identifying lesions that abut the central airways, mimicking mediastinal or hilar adenopathy, thus making the procedure unreliable as a method for staging.
indispensable by identifying optimal biopsy sites, CT may also be of value by identifying cases for which TBNA is not indicated, as it has been shown that the yield of TBNA in patients with negative CT scans is sufficiently low to obviate this procedure (92a). CT can also help to avoid potentially false-positive TBNA by identifying lesions that abut the central airways, mimicking mediastinal or hilar adenopathy, thus making the procedure unreliable as a method for staging.
![]() Figure 5-21 CT-bronchoscopic correlation: diffuse squamous cell carcinoma. See Color Figure 5-21D. A–C: Sequential axial CT images through the carina, right upper lobe bronchus, and lower lobe bronchi, respectively, imaged with lung windows in a patient presenting with a chronic cough show diffuse thickening of the airway walls, associated with extensive mediastinal and hilar adenopathy identifiable even with wide windows, and small bilateral effusions. This appearance is nonspecific and compatible with a diffuse infiltrative or inflammatory process involving the entire tracheobronchial tree. D: Flexible bronchoscopic images in this same case demonstrate diffuse mucosal thickening and nodular irregularity covering most of the surface of the airways. Forceps biopsy revealed diffusely infiltrative squamous cell carcinoma. In this case, CT and bronchoscopy proved complementary by disclosing different aspects of this patient’s clinically unsuspected unresectable tumor. (From Naidich DP, Webb WR, Grenier PA, et al. Imaging of the airways: functional and radiologic correlations. Philadelphia: Lippincott Williams & Wilkins; 2005 , with permission.) |
To date, TBNA has most often been used to evaluate patients with suspected intrathoracic neoplasia, with most series reporting a diagnostic yield of between 60% and 80% sensitivity for diagnosing and staging lung cancer (94, 95, 96, 96a). In one multicenter trial, TBNA resulted in a positive diagnosis in 48% of 279 patients with non–small cell lung cancer (NSCLC), and 62% of 81 patients with small cell carcinoma. Importantly, TBNA also provided the only bronchoscopic specimen diagnostic for lung
cancer in 18% of patients (93). Similarly, in a study of 123 patients with documented malignancy evaluated by TBNA, Sharafkhaneh et al. (94) reported a sensitivity of 69%, with significant correlations noted between a positive TBNA and cell type (p < 0.001) and nodal size (p < 0.05). Comparable with other reported series, TBNA proved most successful in diagnosing small cell carcinoma, less often successful in diagnosing non–small cell carcinoma, and least successful in diagnosing lymphoma (95,96).
cancer in 18% of patients (93). Similarly, in a study of 123 patients with documented malignancy evaluated by TBNA, Sharafkhaneh et al. (94) reported a sensitivity of 69%, with significant correlations noted between a positive TBNA and cell type (p < 0.001) and nodal size (p < 0.05). Comparable with other reported series, TBNA proved most successful in diagnosing small cell carcinoma, less often successful in diagnosing non–small cell carcinoma, and least successful in diagnosing lymphoma (95,96).
![]() Figure 5-23 Central tumor: CT-bronchoscopic correlations. See Color Figure 5-23C,D. A, B: Sequential axial images through the right mainstem bronchus show a well-defined intraluminal mass in this patient status post prior left pneumonectomy. C: Endoscopic view confirming the presence of a well-defined endoluminal tumor consistent with recurrent non–small cell lung cancer. Note the close correlation between CT and bronchoscopy in this case. D: Endoscopic view following cauterization showing near complete removal of the tumor. |
![]() Figure 5-24 CT-bronchoscopic correlation: endobronchial foreign body. See Color Figure 5-24C,D. A, B: Magnified CT sections through the right mainstem–right upper lobe bronchus imaged with wide and narrow windows, respectively, show an unusual-appearing intraluminal filling defect in a patient presenting with bacterial pneumonia and empyema. C: Endoscopic view revealing a foreign body coated with secretions partially obstructing the right main bronchus. This was successfully removed by forceps. D: Image of the same foreign body following removal revealing this to be an aluminum foil packet. The patient subsequently admitted accidentally aspirating the packet containing crack cocaine that he had hidden in his mouth while fleeing the police a month earlier. In this case, the unchanging nature of the endobronchial density on sequential CT scans several days apart prompted the suspicion of a foreign body rather than the initial impression of retained secretions. (From Naidich DP, Webb WR, Grenier PA, et al. Imaging of the airways: functional and radiologic correlations. Philadelphia: Lippincott Williams & Wilkins; 2005 , with permission.) |
![]() Figure 5-26 Central tumor: CT-bronchoscopic correlations. See Color Figure 5-26I. A–D: Sequential axial images through the left hilum imaged with lung windows show a large mass causing marked narrowing/obstruction of the left lower lobe bronchus just distal to the origin of the superior segmental bronchus (arrows in B and C) as well as the superior lingula segment (curved arrows in B and C). Note that this mass crosses the left major fissure to involve both the left upper and lower lobes. E–H: Identical sections as shown in A–D, imaged with mediastinal windows. The precise margins of the tumor are well defined following a bolus of intravenous contrast. The subcarinal nodes are slightly enlarged. I: Endoscopic view of the proximal left lower lobe bronchus confirms the presence of a well-defined intraluminal mass just below the origin of the superior segmental bronchus (arrow). This case again illustrates the complementary nature of CT by delineating the true extraluminal extent of tumor (compare with Figs. 5-6 and 5-21) |
![]() Figure 5-27 CT-bronchoscopic correlation: tumor localization—adenocarcinoma of the lung. See Color Figure 5-27C. A, B: Sequential contrast-enhanced CT images through the subcarinal space and aortic root, respectively, imaged with narrow windows, in a 48-year-old ex-smoker presenting with two episodes of scant hemoptysis, shows extensive tumor throughout the mediastinum. Note that the carina is splayed, and there is slight deformity of the medial and posterior aspect of both the right and left mainstem bronchi (arrows in A) as well as marked narrowing of the middle lobe bronchus (arrowhead in B) and apparent obstruction of the proximal right lower lobe bronchus (arrow in B). This appearance is nonspecific but most often indicative of diffuse extrinsic compression of the airways by extensive malignant adenopathy. Note, as well, marked irregularity of the posterior wall of the left atrium (curved arrow in B). Although these findings are all suggestive of unresectable tumor, evaluation requires more definitive confirmation. C: Sequential images obtained at the time of bronchoscopy in this same patient reveal extrinsic compression of the bronchus intermedius resulting from enlarged adjacent nodes. In this case there is also evidence of eruption of malignant subcarinal lymph node through the wall of the left main bronchus (arrows in C), resulting in endobronchial tumor. Forceps biopsy in the left main bronchus revealed adenocarcinoma. Although the presence of malignant subcarinal lymph nodes staged this patient as at least IIIA (based on an N2 node), confirmation of involvement of the (contralateral) left main bronchus confirmed that there was T4 unresectable tumor. This case points out both the complementary and the competing nature of CT and bronchoscopy for assessing the true extent of tumor. (From Naidich DP, Webb WR, Grenier PA, et al. Imaging of the airways: functional and radiologic correlations. Philadelphia: Lippincott Williams & Wilkins; 2005 , with permission.) |
Less well appreciated is the fact that CT also may be of value as a guide to TBNA in patients with benign mediastinal or hilar disease (96, 97, 98). Morales et al. (97), in a study of 51 consecutive patients with suspected sarcoidosis who underwent both transbronchial lung biopsy (TBBx) and flexible TBNA of mediastinal or hilar nodes, showed that 23% of patients with stage 1 disease and 10% of patients with stage 2 disease had their diagnoses established only with TBNA. Overall, the combined use of TBBx and TBNA allowed a histologic diagnosis in 83% of these cases. In
patients for whom TBNA is contemplated, CT may also prove cost-effective. In the same study, 9 of 51 patients who would otherwise have required surgical assessment to obtain a definitive diagnosis were successfully diagnosed by TBNA; thus, in the judgment of these investigators, the results more than financially justify the routine prebronchoscopic use of HRCT (97). Similar findings have been reported by Cetinkaya et al. (96) in a study of 60 consecutive patients with mediastinal or hilar adenopathy in which TBNA with a 22-gauge Wang cytology needle successfully diagnosed tuberculosis (TB) in 13 (65%) of 20 cases, providing the sole means of diagnosis in 8 (40%). Similar findings have been reported by Trisolini et al. (99), who reported that TBNA revealed non-necrotizing granulomas in 23 (72%) of 32 patients with subsequently documented sarcoidosis. Importantly, TBNA provided the sole means of diagnosing sarcoid in 7 patients who underwent both TBNA and TBBx, improving the diagnostic rate by 47% (96).
patients for whom TBNA is contemplated, CT may also prove cost-effective. In the same study, 9 of 51 patients who would otherwise have required surgical assessment to obtain a definitive diagnosis were successfully diagnosed by TBNA; thus, in the judgment of these investigators, the results more than financially justify the routine prebronchoscopic use of HRCT (97). Similar findings have been reported by Cetinkaya et al. (96) in a study of 60 consecutive patients with mediastinal or hilar adenopathy in which TBNA with a 22-gauge Wang cytology needle successfully diagnosed tuberculosis (TB) in 13 (65%) of 20 cases, providing the sole means of diagnosis in 8 (40%). Similar findings have been reported by Trisolini et al. (99), who reported that TBNA revealed non-necrotizing granulomas in 23 (72%) of 32 patients with subsequently documented sarcoidosis. Importantly, TBNA provided the sole means of diagnosing sarcoid in 7 patients who underwent both TBNA and TBBx, improving the diagnostic rate by 47% (96).
CT has also proved of value in patients with acquired immunodeficiency syndrome (AIDS). In immunocompromised patients, the finding of low-density mediastinal or hilar lymph nodes, in particular, strongly correlates with active mycobacterial infection (100). In these patients, TBNA may represent the least invasive means of obtaining diagnostic material, especially when sputum smears prove nondiagnostic. As documented by Harkin et al. (98), TBNA proved diagnostic in 23 (51%) of 45 procedures performed in 42 human immunodeficiency virus (HIV–positive) patients. This included identification of 21 of 23 documented cases of mycobacterial infection, of which TBNA provided the only diagnostic specimen in 13 (57%) (98).
![]() Figure 5-30 CT-bronchoscopic correlation: sarcoidosis. See Color Figure 5-30C,D. A, B: CT sections through the right and left mainstem bronchi, respectively, imaged with wide windows show well-defined focal lesions (arrows) protruding into the proximal right main (A) and proximal and distal left main (B) bronchi, respectively. Note the presence of diffuse nodular infiltrates in both lungs with a predominantly perilymphatic distribution. C, D: Bronchoscopic images obtained the next day revealed no evidence of focal endobronchial lesions in the right (C) or left (D) main bronchi but did show focal areas of fine mucosal nodularity unsuspected from the CT (not shown). Presumably the large “lesions” shown by CT represented secretions that were no longer present by the time of bronchoscopy, although the mucosal nodularity was too subtle to be detected by CT. In this case, although CT disclosed findings characteristic of parenchymal sarcoidosis, apparent endobronchial lesions proved to represent retained secretions. In the setting of focal endobronchial lesions, performing a repeat CT following strenuous coughing may be of value by minimizing the number of false-positive examinations. (From Naidich DP, Webb WR, Grenier PA, et al. Imaging of the airways: functional and radiologic correlations. Philadelphia: Lippincott Williams & Wilkins; 2005 , with permission.) |
Alternate Methods for Performing Transbronchial Needle Aspiration
Despite the proved efficacy of TBNA as a diagnostic tool, unfortunately, this technique remains underutilized. In a survey of 871 North American bronchoscopists, only 12% routinely used TBNA to diagnose malignancy and only 2% to diagnose benign disease (101). Lack of utilization primarily reflects the widely disparate results reported by most bronchoscopists. As a consequence, a variety of techniques have been recently developed in an attempt to improve the diagnostic yield of TBNA.
Computed Tomography Fluoroscopy and Virtual Bronchoscopy
One approach has been to perform TBNA under direct CT guidance (Fig. 5-31). CT fluoroscopy, in particular, has the advantage of real-time cine visualization of the tip of the needle. Rong and Cui (102), for example, compared their diagnostic yield first performing routine TBNA without and then under direct CT guidance and found this technique improved their yield from 20% to 60%. Using CT fluoroscopy it has been demonstrated that 41% of 116 needle passes were improperly positioned, and subsequent
passes established diagnoses in enlarged lymph nodes in all 12 patients studied (103). All patients in this group had previously undergone a nondiagnostic TBNA by the conventional method. Similar results have been reported by White et al. (104), who reported a yield for CT fluoroscopy–guided TBNA of 83% in 12 patients, all of whom had also undergone a nondiagnostic TBNA by the conventional method.
passes established diagnoses in enlarged lymph nodes in all 12 patients studied (103). All patients in this group had previously undergone a nondiagnostic TBNA by the conventional method. Similar results have been reported by White et al. (104), who reported a yield for CT fluoroscopy–guided TBNA of 83% in 12 patients, all of whom had also undergone a nondiagnostic TBNA by the conventional method.
Additional alternate approaches have made use of VB to plan for optimal biopsy sites (105, 106, 107). VB as a method for preplanning TBNA, for example, has been shown to improve the yield of TBNA (108,109). In one study (110), volumetric rendering was used to first highlight a total of 36 sites in 14 patients in whom enlarged nodes could be identified on routine 3-mm axial images. Although VB did not alter the approach to subcarinal or aorticopulmonary window nodes, virtual bronchoscopic imaging with nodal highlighting increased the successful choice of biopsy sites for hilar nodes (from 37% to 83%; p = 0.001) and for pretracheal nodes (from 59% to 85%; p = 0.07), respectively.
Endobronchial Ultrasound
Over the past decade, considerable interest has focused on the potential of EBUS to diagnose both central (111, 112, 113, 114, 115, 116, 117, 118, 119, 120) and peripheral lesions (120a,121,122). In comparison to all other imaging modalities, including direct endoluminal visualization, EBUS has the great advantage of being able to differentiate the various layers of the bronchial wall. Specifically, as many as seven layers within the wall of large airways have been identified, including two of the inner mucosa, three of cartilage, and two of the outer adventitia (Fig. 5-32). Vessels can be reliably identified by their pulsation and low internal echo density and are easily differentiated from lymph nodes as small as 2 mm that can be identified by their high echo density. In distinction, hypoechoic areas and invasion of the cartilage layer have been shown to characterize malignant tissue (112).
![]() Figure 5-32 A–E: Endobronchial ultrasound (EBUS). See Color Figure 5-32C,D. A: In vitro endobronchial ultrasound appearance of the normal trachea showing five identifiable portions of the airway wall. From inside out these include one hyperechoic layer representing mucosa and submucosa, a second anechoic layer representing cartilage, and a third echogenic layer representing the adventitia. As illustrated in this figure, these layers measure 1, 1.1, and 0.9 mm, respectively. Note that the endobronchial probe lies eccentrically within the tracheal lumen and that the definition of the tracheal wall is nonuniform, resulting from lack of uniform contact between the probe and the mucosa. (From Herth F, et al. Endobronchial ultrasound improves classification of suspicious lesions detected by autofluorescence bronchoscopy. J Bronchol. 2003;10:249–252 , with permission.) B: Endoscopic ultrasound image shows evidence of asymmetric wall thickening measuring 3 mm (vs. normal 1.4 mm), in this case due to tumor confined to the bronchial wall without evidence of adenopathy. C, D: Endoscopic view of a bulky adenoid cystic carcinoma prior to and following laser resection. In this case, laser resection appears to have eradicated the tumor. E: Follow-up endobronchial ultrasound examination shows that there is residual tumor eccentrically located in the anterolateral tracheal wall (arrow). These cases illustrate the advantage of EBUS for exquisite delineation of tracheal and bronchial wall anatomy. (B–E courtesy of Heinrich Becker, MD, University of Heidelberg, Germany.) |
As a result, EBUS provides a unique method for assessing pathologic changes both in and adjacent to the airways. Takahishi et al. (123), for example, in a study of 22 patients with radiographically occult lesions, have shown that EBUS can evaluate the depth of tumor invasion of airway walls differentiating tumors that remained separate from those extending to the cartilaginous layer with a sensitivity of 86%, a specificity of 67%, and an accuracy of 80%. Despite its overall accuracy for detecting bronchial pathology, CT is incapable of such fine distinctions. Herth et al. (124), for example, comparing the ability of CT versus EBUS to distinguish extrinsic airway compression from tumor invasion in 105 patients, confirmed the superior sensitivity (89% vs. 75%), specificity (100% vs. 28%), and accuracy (94% vs. 51%) of EBUS. This compares favorably with a previous report of 93% accuracy for endobronchial
ultrasonographic evaluation of tracheobronchial invasion by tumor in 15 surgically resected patients (111).
ultrasonographic evaluation of tracheobronchial invasion by tumor in 15 surgically resected patients (111).
Not surprisingly, given the ability of EBUS to accurately identify nodes as small as 2 mm adjacent to the central airways, attention has focused on the use of this technique for guiding TBNA. To date, numerous reports have documented the efficacy of EBUS for staging lung cancer, in particular (113,114,116, 117, 118, 119, 120,125, 126, 127, 128, 129), as well as in the diagnosis of benign disease (130,131). Herth et al. (117), for example, using a dedicated EBUS probe, reported successful sampling by EBUS-guided TBNA of lymph nodes ranging from 8 to 43 mm in 207 (86%) of 242 patients with malignancy confirmed in 72% (117). In a follow-up randomized trial, EBUS-guided TBNA was found to be significantly better than conventional TBNA for all nodal stations combined except those in the subcarinal space (84% vs. 58%) (132). Currently, the major limitations of EBUS-guided TBNA are the expense of this procedure, as well as limitations due to the necessity for endoscopic probes to be specially configured to provide consistent positioning adjacent to bronchial walls. Although a dual-channel bronchoscope has been developed, allowing direct visualization of the TBNA needle entering lymph nodes directly, this scope is currently limited to this one use only.
Therapeutic Correlations
In addition to the use of CT for detecting bronchial lesions and guiding diagnostic procedures, CT also plays an essential role in treatment planning. This is especially important given the wide range of potential interventional bronchoscopic techniques now available for treating both benign and malignant airway disease, making interventional bronchoscopy an essential option for treating endobronchial disease (9). In this category are a number of different and competing techniques, including neodymium: yttrrium-aluminum-garnet (Nd:YAG) laser phototherapy,
photodynamic therapy, cryotherapy, electrocautery, brachytherapy, and argon plasma coagulation (77). In these cases, the main role of CT is to delineate the true extraluminal extent of tumor (Fig. 5-23). CT also plays an especially important role in the use of airway stents by predetermining their optimal diameter and length (78). As shown by Miyazawa et al. (132a), in conjunction with spirometry, ultrathin bronchoscopy, and EBUS, multiplanar CT images are of use in determining the choke point of flow limitation in malignant endobronchial lesions prior to and following stent placement. CT is also of proven value for follow-up evaluation to identify stent migration or obstruction resulting from excessive granulation tissue or tumor recurrence (Fig. 5-33) (37).
photodynamic therapy, cryotherapy, electrocautery, brachytherapy, and argon plasma coagulation (77). In these cases, the main role of CT is to delineate the true extraluminal extent of tumor (Fig. 5-23). CT also plays an especially important role in the use of airway stents by predetermining their optimal diameter and length (78). As shown by Miyazawa et al. (132a), in conjunction with spirometry, ultrathin bronchoscopy, and EBUS, multiplanar CT images are of use in determining the choke point of flow limitation in malignant endobronchial lesions prior to and following stent placement. CT is also of proven value for follow-up evaluation to identify stent migration or obstruction resulting from excessive granulation tissue or tumor recurrence (Fig. 5-33) (37).
![]() Figure 5-33 CT-bronchoscopic correlation: use in therapeutic planning—stent evaluation. See Color Figure 5-33B,C. A: CT of a patient with esophageal carcinoma who had previously required endobronchial stent placement in the left main bronchus resulting from extrinsic compressive obstruction of the bronchus by the primary tumor. CT was obtained to evaluate dyspnea 4 weeks after an uncovered self-expanding metal stent had been placed within the left mainstem bronchus. Note that in addition to extensive posterior mediastinal tumor, there is soft tissue density filling the lumen of the stent (arrow). B: Flexible bronchoscopy confirmed the presence of tissue obstructing the stent. Forceps biopsy revealed invasive esophageal carcinoma. C: Flexible bronchoscopic appearance of the left main bronchus after removal of the obstructing tumor by argon plasma coagulation via the flexible bronchoscope. Patent distal airways are seen (arrow). Because of the electroconductive nature of metal stents, this technique is safer than electrocautery within the lumen of a stent and is much less likely to damage the stent than is the Nd:YAG laser. (From Naidich DP, Webb WR, Grenier PA, et al. Imaging of the airways: functional and radiologic correlations. Philadelphia: Lippincott Williams & Wilkins; 2005 , with permission.) |
Abnormalities Involving the Trachea and Mainstem Bronchi
Identification of tracheal disease is notoriously difficult. Clinically, it has been estimated that lesions need to occlude more than 75% of the lumen before the development of symptoms related to airway obstruction, and even then these are frequently mistaken as being due to asthma. Early diagnosis is rare and usually occurs as a result of hemoptysis. Radiographically, the trachea has been described as the blind spot in the chest, with lesions characteristically identified only when large (133). For descriptive purposes, this section addresses first focal and then diffuse tracheal abnormalities, with an acknowledgment that this distinction may be arbitrary (Table 5-3).
Table 5-3 Classification of Diseases of the Trachea and Mainstem Bronchi | ||||||||||||||||||||||
---|---|---|---|---|---|---|---|---|---|---|---|---|---|---|---|---|---|---|---|---|---|---|
|
Focal Tracheal Abnormalities
Tracheal Trauma
It is unusual for patients suffering tracheal trauma to live long enough to be evaluated: not surprisingly, therefore, most series are limited in number (134). Trauma may be either penetrating or more often blunt (Fig. 5-34). Although penetrating tracheal injuries typically involve the neck, blunt traumatic injuries usually involve the intrathoracic portions of the trachea, often extending to the mainstem bronchi (135). Blunt trauma may also result in pulmonary contusion or laceration, thoracic spine injuries, and esophageal disruption, as well as multiple fractures. Extrathoracic organ injury is also frequently encountered (135a). Most often the result of deceleration or so-called steering wheel injuries, proposed mechanisms of injury include compression of the airways between the sternum and thoracic spine with lateral expansion of the thorax, shearing at fixation points especially at the carina, and elevated intratracheal pressure, especially against a closed glottis (135b). These result either in transverse lacerations, in disruption of the posterior, membranous portion of the trachea or, less commonly, in shearing of the mainstem bronchi from the carina (Fig. 5-34). Although the entire length of the trachea is vulnerable following blunt trauma, more than 80% of cases occur within 2.5 cm of the carina (134). The left mainstem bronchus is less frequently disrupted, likely because of its longer course through the mediastinum, which offers some extra measure of protection. Additional causes of tracheal rupture include overdistension of endotracheal tubes and traumatic intubation (136).
![]() Figure 5-34 Blunt trachea trauma: imaging techniques. See Color Figure 5-34D. A, B: Multiplanar (MPR) and minimum projection (MinIP) coronal reconstructions, respectively, through the trachea show extensive mediastinal and deep cervical emphysema. Note the absence of a pneumothorax. C: Axial image through the mid trachea shows a focal defect in the posterior membranous trachea with resultant extensive pneumomediastinum. D: Virtual bronchoscopic image pointing from within the mid trachea toward the carina shows a deep groove in the posterior membranous trachea (arrow) extending toward the origin of the right mainstem bronchus. Subsequent bronchoscopic evaluation confirmed the presence of a membranous tear corresponding anatomically to the virtual bronchoscopic image. (Case courtesy of James Gruden, MD, Phoenix, Arizona.) |
Chest radiographic findings most often include nonspecific pneumomediastinum and/or deep cervical emphysema (137). Pneumomediastinum most commonly occurs secondary to air leaks originating in the pulmonary interstitium, the so-called Macklin effect (138). Pneumothoraces are also common, presumably the result of disruption of the mediastinal pleura. In those cases in which the airway is partly occluded by blood clots or compression by an adjacent mediastinal hematoma, the first sign of tracheal rupture may be a persistent air leak following chest tube insertion. In more severe cases, bronchial avulsion may lead to a falling away of the involved lung from the hilum due to disruption of central attachments, the so-called fallen lung sign (137).
CT findings in patients with blunt trauma have been described (138, 139, 140, 141, 142). In one retrospective study of 14 patients with tracheal rupture either due to trauma or following intubation, CT proved diagnostic in 85% of cases, providing direct visualization of tracheal tears in 10 (71%). Direct signs of tracheal rupture included focal wall defects in more than 50% of cases (Fig. 5-34) and, less commonly, a deformed tracheal lumen. In this same series, overdistension of the tube balloon was noted to be particularly common, occurring in 5 of 7 intubated patients. Of particular value was the finding of herniation of the endotracheal tube balloon outside the confines of the tracheal wall. MPR or VB images should be obtained in all cases in which tracheal injuries are suspected, as these may disclose subtle abnormalities not readily identified in cross section (Fig. 5-34).
Despite the use of advanced imaging techniques, definitive diagnosis and therapy nearly always require bronchoscopy. Rare exceptions include patients in whom the diagnosis can be made presumptively owing either to direct observation or to evidence of a massive air leak following chest tube placement (143). Although treatment typically involves surgical repair, conservative therapy has been advocated for individuals with postintubation membranous tracheal ruptures if these are less than 2 cm in length (136,144). Late manifestations of tracheal rupture include wheezing secondary to tracheal stricture, potentially mistakenly diagnosed as asthma.
Tracheal Stenosis
Benign airway strictures may be either congenital or acquired. In either case, accurate evaluation requires that the precise length and degree of stenosis be assessed along with associated peritracheal or bronchial abnormalities. Among acquired strictures, the most common cause is iatrogenic injury resulting from intubation. Although investigators have estimated that complications occur in up to 10% to 15% of patients after intubation and in up to 40% of patients after tracheotomies, the incidence of serious complications after intubation has decreased dramatically since the introduction of high-volume/low-pressure tubes (145). The two principal sites of postintubation stenosis are at the stoma or at the level of the endotracheal tube or tracheostomy tube balloon (48). In both cases, strictures result from pressure necrosis, which causes ischemia and subsequent scarring. Stenosis also may be due to inflammation with subsequent thinning and weakening of the tracheal wall (tracheomalacia) in the segments between the stoma and the cuff; narrowing may also be caused by granulation tissue resulting from direct tracheal injury by the endotracheal or tracheostomy tube tip (48). Other complications of intubation include tracheal rupture and tracheo-brachiocephalic artery or tracheoesophageal fistulas, the latter usually occurring in the presence of combined tracheal and esophageal intubation.
In addition to postintubation stenoses, tracheobronchial strictures also occur as a result of infection, in particular, TB and histoplasmosis, and after lung transplantation. After lung transplantation, complications commonly occur at the level of the bronchial anastomosis and include dehiscence, necrosis, and stenosis from malacia, fibrous stricture, prominent granulation tissue formation, or a combination of these conditions (35,146, 147, 148, 149, 150, 151, 152, 153).
Regardless of the etiology, CT, especially when performed using volumetric techniques, has proved a reliable method for assessing tracheobronchial strictures (Fig. 5-35) (1,154, 155, 156, 157, 158). In all cases, accurate evaluation requires that the precise length and degree of stenosis be assessed as well as associated peritracheal abnormalities. As documented by Whyte et al. (158), in their study of 25 patients with known or suspected stenosis, CT with MPRs demonstrated the site and degree of tracheal or main bronchial stenoses with a sensitivity of 93% and a specificity of 100%. As discussed in the section on trauma, in select cases the degree of stenoses may also be accurately judged by use of VB (40).
Although endoscopic management of tracheal stenoses has been proposed, especially the use of lasers or stents, these typically provide only temporary benefits. Primary tracheal and/or laryngotracheal resection and reconstruction remain the optimal method for treating non-neoplastic stenoses, with resection up to 4 cm in length feasible, the equivalent of eight cartilaginous rings (159). In one series of 58 cases of postintubation or idiopathic stenoses, major complications occurred in 12%, including anastomotic dehiscence and vocal cord paralysis (159).
Tracheal Neoplasia
Etiology
Although many different tracheal tumors, both benign and malignant, have been reported, primary tracheal neoplasia is rare (160). These tumors include both primary epithelial and mesenchymal neoplasms and secondary neoplasia due to either metastases or, more commonly, direct tracheal invasion by adjacent mediastinal neoplasms, especially carcinomas arising from the lung, thyroid, and esophagus (161). In fact, only a few lesions occur with any frequency, with SCCs and adenoid cystic carcinomas (AACs) together accounting for up to 86% of cases (162). Other, relatively rarer malignant lesions encountered include carcinoid and mucoepidermoid tumors, whereas hamartomas and bronchial papillomas are the most common benign tumors encountered. Although clearly distinct histologically, most of these entities clinically present with nonspecific symptoms including cough, wheezing, stridor, and hemoptysis, and radiographic and CT appearances of both benign and malignant tumors similarly tend to overlap.
Squamous Cell Carcinoma
SCC most often occurs in middle-aged male smokers, and not surprisingly it has been found to be associated with other malignant diseases of the respiratory tract in as many as one third of patients
(Fig. 5-36) (162). In approximately 10% of cases, these tumors prove to be multifocal and are frequently found to extend into the mainstem bronchi or adjacent esophagus, resulting in malignant esophageal-bronchial fistulas (162).
(Fig. 5-36) (162). In approximately 10% of cases, these tumors prove to be multifocal and are frequently found to extend into the mainstem bronchi or adjacent esophagus, resulting in malignant esophageal-bronchial fistulas (162).
Sialadenoid Tumors
Sialadenoid tumors are pulmonary analogs of the salivary glands arising from tracheobronchial mucous glands and most often prove to be AACs. The second most frequent lesions in this group are mucoepidermoid cancers, making up approximately 5% of the total. Less commonly encountered are mucous gland adenomas, pleomorphic adenomas, and acinic cell carcinomas, and still more rare are pulmonary oncocytomas and myoepitheliomas (163, 164, 165). AACs are low-grade lesions that typically arise from the tracheobronchial mucous glands, most frequently on the posterolateral wall of the trachea (Figs. 5-29 and 5-37). Along with SCC, ACC shows a marked propensity for local, submucosal, and perineural lymphatic invasion. Metachronous hematogenous metastases have been reported to be common, occurring in
44% of cases in one series (166). Unlike SCCs, but in common with mucoepidermoid tumors and carcinoids, no known association exists between ACCs and cigarette smoking and there is no predilection for either sex. ACC typically occurs in slightly younger patients with a mean age of between 40 and 50 years.
44% of cases in one series (166). Unlike SCCs, but in common with mucoepidermoid tumors and carcinoids, no known association exists between ACCs and cigarette smoking and there is no predilection for either sex. ACC typically occurs in slightly younger patients with a mean age of between 40 and 50 years.
Carcinoid Tumors
Carcinoid tumors are neuroendocrine neoplasms that arise from bronchial or bronchiolar epithelium. They are presumed to arise either from existing Kulchitsky cells, neuroepithelial bodies, or pluripotential bronchial epithelial stem cells. They present a spectrum of histologic subtypes ranging from low- to high-grade neoplasms and are classified as typical carcinoids, atypical carcinoids, and large cell neuroendocrine carcinomas, with the highest grades merging with small cell carcinoma (167). In addition to findings related to bronchial obstruction, carcinoid tumors cause hemoptysis in up to 50% of cases, reflecting their rich hypervascular stroma. In addition, carcinoid tumors are known to produce a variety of hormones and neuroamines, including adrenocorticotropic hormone (ACTH), serotonin, and somatostatin, among others. Cushing syndrome has been reported, although rarely. Carcinoid syndrome may also occur, although only in patients with known liver metastases (167).
Most endobronchial carcinoid tumors are central in location. Easily identified bronchoscopically, they typically appear as smooth, cherry-red lesions with a propensity to bleed on biopsy (Fig. 5-38). Although massive hemoptysis has been reported, in fact, these lesions are usually safely biopsied. They tend to exhibit variable growth patterns. Most often they extend extraluminally, resulting in so-called iceberg lesions. With more peripheral lesions, airway obstruction may result in focal mucoid impaction.
On CT, endobronchial carcinoid tumors most often present either as hilar masses or discrete intraluminal lesions (Fig. 5-38). A characteristic triad of features has been described, including the finding of a well-defined round or slightly lobulated tumor causing narrowing of the adjacent airway, associated with eccentric calcifications (168). These latter are commonly seen, with calcifications identifiable in up to a third of cases (169,170). Carcinoid tumors are also well known to markedly enhance following administration of intravenous contrast media.
Benign Tracheal Neoplasms
The most common benign tracheal neoplasm is squamous cell papilloma. Although, like SCCs, these most often occur in middle-aged male smokers, squamous cell papillomas typically involve the larynx or bronchi, with tracheal involvement uncommon: More important, involvement, when present, is limited to the tracheal wall (161).
Laryngotracheobronchial papillomatosis refers to a condition associated with multiple papillomas (Fig. 5-39) (171, 172, 173). Histologically similar to solitary papillomas, this condition most often occurs in children younger than 5 years and usually is restricted to the larynx, with spontaneous resolution noted in most cases. Furthermore, papillomatosis has been shown to be etiologically linked to infection with human papillomavirus, either contracted at birth or acquired through sexual transmission. Papillomatosis has been noted to occur in patients with AIDS. Widespread disease occurs in a few patients. As shown by Kramer et al. (172) in a literature review of 532 patients, tracheal and bronchial involvement occurs in only approximately 5% of cases, whereas pulmonary parenchymal disease occurs in less than 1%. Unfortunately, in this population, spontaneous remission is unusual, with malignant transformation to SCC reported in adults. The origin of parenchymal disease is controversial. Because tracheobronchial and parenchymal disease is rarely encountered without coexistent laryngeal disease, investigators have postulated that spread is by endobronchial dissemination. An association between distal spread and prior tracheostomy further supports this cause. Typically, lesions appear first as well-defined nodules, occasionally identifiable as centrilobular, that subsequently undergo central necrosis resulting in multiple thin-walled cavities. Bronchiectasis, with or without parenchymal consolidation and atelectasis, is often identified in association with nodular densities, presumably the result of bronchial occlusion and subsequent inflammation or infection.
Another lesion rarely encountered is an endobronchial hamartoma. Commonly presenting as a solitary pulmonary nodule (Fig. 5-25), endobronchial hamartomas are rare, estimated to represent between 10% and 20% of intrathoracic hamartomas (174). Hamartomas are mesenchymal lesions composed of a mixture of cartilage, fat, and fibrous tissue and most often occur in segmental bronchi; tracheal involvement is distinctly unusual. In a report of 43 patients with documented endobronchial hamartomas, the most common presenting symptoms were recurrent respiratory tract infections and hemoptysis, occurring in 44% and 33% of cases, respectively (174). On CT, although infrequently reported, the presence of fat is considered diagnostic of this entity (Fig. 5-15) (175,176).
Tracheal and Mainstem Bronchial Neoplasms: CT Findings
Not surprisingly, the CT appearances of tracheal neoplasms overlap. Indeed, regardless of cell type, these tumors have in common the finding of an endobronchial soft tissue mass or asymmetric tracheal or bronchial wall thickening causing eccentric narrowing of the tracheal or carinal lumen (Figs. 5-29 and 5-36, 5-37, 5-38, 5-39) (177). Asymmetric tracheal or bronchial wall thickening may be exceedingly subtle, especially in patients with benign lesions such as papillomas. Because retained secretions may also have this appearance, the examiner often needs to acquire additional images after requesting patients to cough (Fig. 5-40). Although the presence of calcification should raise the suspicion of a mesenchymal tumor, especially chondromatous lesions, definitive diagnosis still
requires histologic evaluation in cases of focal tracheal disease. However, whereas endoscopic biopsy can reliably establish the diagnosis, adequate preoperative evaluation requires accurate definition of the extent of extraluminal involvement if present. This is especially important because as much as one half of the length of the trachea may be successfully resected in the absence of mediastinal invasion (48).
requires histologic evaluation in cases of focal tracheal disease. However, whereas endoscopic biopsy can reliably establish the diagnosis, adequate preoperative evaluation requires accurate definition of the extent of extraluminal involvement if present. This is especially important because as much as one half of the length of the trachea may be successfully resected in the absence of mediastinal invasion (48).
![]() Figure 5-40 Retained secretions. A, B: Magnified views of the carina imaged with wide and narrow windows, respectively, show a well-defined filling defect in the left mainstem bronchus mimicking the appearance of an endobronchial tumor (arrow) (compare with Fig. 5-38). C, D: Magnified views at the same levels as shown in A and B following cough. On occasion retained secretions and/or endoluminal hematomas may simulate neoplasia. |
Tuberculosis of the Trachea and Mainstem Bronchi
TB typically involves the distal trachea and proximal mainstem bronchi; isolated tracheal disease is rare (178, 179, 180, 181, 182, 183, 184). Active inflammation causes irregular circumferential bronchial wall thickening, resulting in narrowed or even obstructed airways. Enhancement of the tracheal wall has been reported in patients with acute inflammation after the administration of intravenous contrast material (183). In most cases, active infection results from extension from peribronchial lymphatic disease, although endobronchial implantation from contaminated sputum has also been postulated as a potential mechanism (Fig. 5-41) (181,183). Evidence of associated mediastinal inflammation, in the form of diffuse increased density of mediastinal fat or enlarged mediastinal or pericarinal nodes, may also be identified (178,181, 182, 183). If this condition is untreated, ulceration with resulting fistulas between nodes and adjacent airways may develop. With appropriate therapy, these changes are usually, although not invariably, reversible. Unlike active infection, fibrotic TB results in smoothly narrowed airways without evidence of either wall thickening or active peribronchial inflammation (178,181, 182, 183). Unlike active infection, which involves both mainstem bronchi equally, fibrotic TB usually involves the left main bronchus, perhaps because of its greater length. CT findings in patients with central airway TB are rarely specific. As noted by Moon et al. (183), findings that help to differentiate tuberculous disease from bronchogenic carcinoma include longer circumferential involvement and the absence of an intraluminal mass. Notwithstanding these distinctions, accurate diagnosis usually requires histologic evaluation.
Diffuse Tracheal Disease
In addition to tracheal tumors, many different inflammatory abnormalities, both infectious and noninfectious, diffusely affect the trachea and mainstem bronchi (Table 5-3) (185). These include both primary tracheal abnormalities and those resulting from spread of adjacent mediastinal inflammation, and they may manifest as either diffuse narrowing or dilatation.
Diffuse Tracheal Widening/Tracheobronchomegaly
Also referred to as Mounier-Kuhn syndrome, the term tracheobronchomegaly refers to a heterogeneous group of patients who have marked dilatation of the trachea and mainstem bronchi, frequently in association with tracheal diverticulosis, recurrent lower respiratory tract infections, and bronchiectasis (186, 187, 188). The etiology of this disorder
is controversial. Findings in favor of a congenital etiology include histopathologic evidence of deficiency of tracheobronchial muscle fibers and absence of the myenteric plexus as well as an association with other congenital or connective tissue disorders, including ankylosing spondylitis, Marfan syndrome, cystic fibrosis (CF), Ehlers-Danlos syndrome, and cutis laxa in children (189,190). In distinction, findings in favor of an acquired etiology include the fact that tracheobronchomegaly most often is diagnosed in men in their third and fourth decades without an antecedent history of respiratory tract infection, often in association with chronic cigarette smoking (189,190). An association between tracheomegaly and diffuse pulmonary fibrosis has also been reported, presumably the result of increased traction on the tracheal wall due to increased elastic recoil pressure in both lungs exerting opposing force (189,190).
is controversial. Findings in favor of a congenital etiology include histopathologic evidence of deficiency of tracheobronchial muscle fibers and absence of the myenteric plexus as well as an association with other congenital or connective tissue disorders, including ankylosing spondylitis, Marfan syndrome, cystic fibrosis (CF), Ehlers-Danlos syndrome, and cutis laxa in children (189,190). In distinction, findings in favor of an acquired etiology include the fact that tracheobronchomegaly most often is diagnosed in men in their third and fourth decades without an antecedent history of respiratory tract infection, often in association with chronic cigarette smoking (189,190). An association between tracheomegaly and diffuse pulmonary fibrosis has also been reported, presumably the result of increased traction on the tracheal wall due to increased elastic recoil pressure in both lungs exerting opposing force (189,190).
![]() Figure 5-41 Tuberculous tracheitis. See Color Figure 5-41C. A, B: Contrast-enhanced axial images through the mid trachea, imaged with wide and narrow windows, respectively, show extensive low-density, rim-enhancing right paratracheal nodes causing eccentric narrowing of the posterolateral tracheal wall. Note apparent small nodule in the anterior tracheal wall in A (arrow). C: Bronchoscopic image corresponding to the CT section in A shows extrinsic compression of the posterolateral tracheal wall on the right side (asterisk), associated with diffuse, irregular nodularity of the tracheal mucosa. Suspected polypoid lesion seen on CT is seen to better effect endoscopically. Endoscopically proved tuberculosis. (From Naidich DP, Webb WR, Grenier PA, et al. Imaging of the airways: functional and radiologic correlations. Philadelphia: Lippincott Williams & Wilkins; 2005 , with permission.) |
CT findings in patients with tracheobronchomegaly have been extensively described (188,191, 192, 193). Using a tracheal diameter of greater than 3 cm measured 2 cm above the aortic arch, and diameters of 2.4 and 2.3 cm for the right and left main bronchi, respectively, the diagnosis of tracheobronchomegaly is relatively straightforward (191). Additional findings include tracheal scalloping or diverticula, the latter especially common along the posterior tracheal wall. Also common is the finding of marked tracheal flaccidity, identifiable as a marked decrease in the diameter of the trachea on expiration, even to the point of airway occlusion, indicative of tracheomalacia (27). Optimal evaluation of this disorder requires volumetric data acquisition as a minimum through the length of the trachea, ideally through the entire thorax (193a). In addition to allowing the reconstruction of high-quality MPRs or 3D volumetric images,
volumetric data acquisition also provides invaluable information regarding peripheral air trapping, a frequent ancillary finding. Although precise quantitative estimation of the severity of tracheomalacia has also been reported using electron beam CT (EBCT), this technique remains generally less available (193b).
volumetric data acquisition also provides invaluable information regarding peripheral air trapping, a frequent ancillary finding. Although precise quantitative estimation of the severity of tracheomalacia has also been reported using electron beam CT (EBCT), this technique remains generally less available (193b).
The importance of tracheomegaly lies in its association with distal airway inflammation. In a study of 75 consecutive patients referred for CT evaluation of possible bronchiectasis, Roditi and Weir (189) found that, overall, 12% of their patients proved to have dilated tracheas, including 7 (17%) of 42 patients with CT evidence of bronchiectasis as well as 3 (6%) of 32 without. These data suggest that tracheomegaly may play a causative role in the development of bronchiectasis as a result of a predisposition to infection from abnormal mucus clearance in patients with inefficient cough and stagnant mucus.
Tracheomalacia may also be seen in association with a number of disorders in addition to tracheobronchomegaly, including most causes of diffuse tracheal inflammation such as relapsing polychondritis, as well as following trauma or especially in patients with chronic bronchitis, among others. Symptoms are typically nonspecific and include chronic cough, dyspnea, and occasionally hemoptysis (194). Tracheomalacia has also been associated with spontaneous tracheal rupture (144). In cases for which this diagnosis is suspected, additional expiratory scans may be essential. Aquino et al. (195) showed that the probability of tracheomalacia varied between 89% and 100% when the change in the sagittal diameter of the trachea exceeded 29%.
![]() Figure 5-42 Saber-sheath trachea. See Color Figure 5-42B. A: Axial section at the level of the aortic arch shows that the sagittal length of the trachea is more than twice the width of the trachea—a finding characteristic of a saber-sheath trachea. B: Bronchoscopic image in the same patient shows similar appearance of marked narrowing of the width of the trachea. Note that, although the lumen of the trachea appears smooth on CT, accurate evaluation of the tracheal mucosa requires endoscopy. (From Naidich DP, Webb WR, Grenier PA, et al. Imaging of the airways: functional and radiologic correlations. Philadelphia: Lippincott Williams & Wilkins; 2005 , with permission.) |
Diffuse Tracheal Narrowing
Included in the group of patients with diffuse narrowing are those with infectious disorders, including bacterial, viral, and fungal infections either primary or secondarily resulting from adjacent mediastinitis, as well as patients with a variety of noninfectious disorders, including tracheopathia osteochondrolytica, relapsing polychondritis, Wegener granulomatosis (WG), amyloidosis, sarcoidosis, and ulcerative colitis (185,186,196). Although many of these have similar appearances in cross section, some are sufficiently distinctive to allow a definitive diagnosis with appropriate clinical correlation (197).
Saber-Sheath Trachea
Saber-sheath trachea is a common variation in tracheal morphology (Fig. 5-42) in which the internal side-to-side diameter of the trachea is decreased to half or less the corresponding sagittal diameter. Although this condition is classically described as a static deformity, further narrowing of the tracheal lumen can be documented when patients are examined using CT obtained during forced expiration or a Valsalva maneuver (196,198). Although it has long been appreciated that saber-sheath tracheas are always associated with chronic obstructive pulmonary disease (199), only recently has it been shown that there is a significant relationship between tracheal dimensions and the severity of emphysema (200). In a study evaluating tracheal morphology in patients before and after lung volume reduction surgery, the length of the trachea decreased while the width increased in size. Unfortunately, these changes proved to be poor predictors of postoperative lung function (200).
Infectious Tracheobronchitis
A number of infections, both acute and more often chronic, may affect the trachea and proximal bronchi, resulting in both focal and diffuse airway disease. Of particular interest is the rare presentation of acute bacterial tracheitis and rhinoscleroma.
Acute tracheitis is almost always seen in children, only rarely presenting in adults, usually in association with immunodeficiency syndromes or AIDS (201). It is characterized by acute upper airway obstruction resulting in stridor. Endoscopically there is evidence of purulent debris, mucosal ulcerations, and edema (Fig. 5-21).
Rhinoscleroma (RS) is a slowly progressive granulomatous infection caused by the bacterium Klebsiella rhinoscleromatis, a capsulated gram-negative bacterium (202, 203, 204). Characterized by involvement of the upper respiratory tract, including the nose, upper lip, hard palate, and maxillary sinuses, this infection may also involve the trachea and proximal bronchi in up to 10% of cases (204). Although RS is endemic in tropical and subtropical areas, with more than 80% of cases reported from Central and South America, Africa, India, Indonesia, and Eastern and Central Europe (205), the prevalence of this disease is rising in the United States because of increasing ease of international travel. The diagnosis is generally established either by biopsy or positive cultures, present in approximately 50% of cases.
![]() Figure 5-43 Rhinoscleroma. See Color Figure 5-43D. A–C: Axial CT sections at the level of the thoracic inlet, distal trachea, and proximal lower lobe bronchi, respectively, in a 26-year-old immigrant complaining of persistent cough and chest pain. These images show a combination of nodular thickening of the tracheal and bronchial walls, resulting in marked luminal narrowing associated with mediastinal and hilar adenopathy. D: Endoscopic biopsy of the tracheal wall shows squamous mucosa with acute inflammation, characterized by dense infiltration with plasma cells, lymphocytes, and histiocytes. Subsequent evaluation revealed intracellular bacilli consistent with rhinoscleroma. (From Naidich DP, Webb WR, Grenier PA, et al. Imaging of the airways: functional and radiologic correlations. Philadelphia: Lippincott Williams & Wilkins; 2005 , with permission.) |
On CT there is evidence of nonspecific diffuse nodular thickening of the trachea and proximal bronchi causing airway narrowing (Fig. 5-43). There may also be evidence of mediastinal or hilar adenopathy. With airway obstruction, patchy parenchymal consolidation may also be identified. Although antibiotics generally result in immediate improvement, RS is difficult to eradicate, with the infection frequently relapsing.
Fungal Tracheobronchitis/Aspergillosis
Airway disease caused by aspergillosis takes many forms, including saprophytic colonization, tracheobronchitis, ulcerative tracheobronchitis with or without pseudomembrane formation, and necrotizing (invasive) aspergillosis (206), the latter further subdivided into acute and chronic airway invasive forms (207). Acute tracheobronchitis typically occurs only in severely immunocompromised patients, including those with underlying malignancies or AIDS or following bone marrow, lung, or heart transplants (208). Histologically, there is evidence of respiratory epithelial ulceration and submucosal inflammation typically restricted to the central airways. CT examination reveals nonspecific multifocal or diffuse tracheobronchial wall thickening, causing either smooth and/or nodular narrowing of the airway lumen (206,207,209). Unfortunately, antifungal therapy generally proves unsuccessful, especially in severely immunocompromised patients. Acute obstruction of the right mainstem bronchus with subsequent bronchial wall necrosis and rupture of the adjacent pulmonary artery due to necrotizing tracheobronchitis in an immunocompromised patient has been reported as a cause of mortality (210).
![]() Figure 5-44 CT-bronchoscopic correlations: tracheobronchopathia osteochondroplastica (TO). See Color Figure 5-44B. A: Magnified view of the mid trachea imaged with narrow windows shows characteristic appearance of calcified nodules resulting in mild narrowing of the tracheal lumen. Note the absence of involvement of the posterior membranous tracheal wall. This proved to be an incidental finding. B: Corresponding flexible bronchoscopic evaluation demonstrates precise CT-endoscopic correlation with characteristic nodular excrescences protruding into airway, pathognomonic of this disease. Spirometry and flow-volume loop revealed minimal obstructive dysfunction. (From Naidich DP, Webb WR, Grenier PA, et al. Imaging of the airways: functional and radiologic correlations. Philadelphia: Lippincott Williams & Wilkins; 2005 , with permission.) |
Tracheobronchopathia Osteochondroplastica
Tracheobronchopathia osteochondroplastica is a rare disorder involving the trachea and main bronchi in which multiple submucosal nodules representing bone, cartilage, or calcified acellular protein matrix may be identified, resulting in diffuse narrowing of the airway lumen (Fig. 5-44) (211, 212, 213). Characteristically, these nodules spare the membranous posterior wall of the trachea. Often asymptomatic, tracheopathia osteochondroplastica has been reported to cause hemoptysis in as many as 25% of cases (214). Other symptoms, including cough, dyspnea, hoarseness, stridor, and recurrent lower respiratory tract infections, have also been noted. Typically occurring in middle-aged men, the course of this disease is generally benign, and in most cases the diagnosis is made only at autopsy.
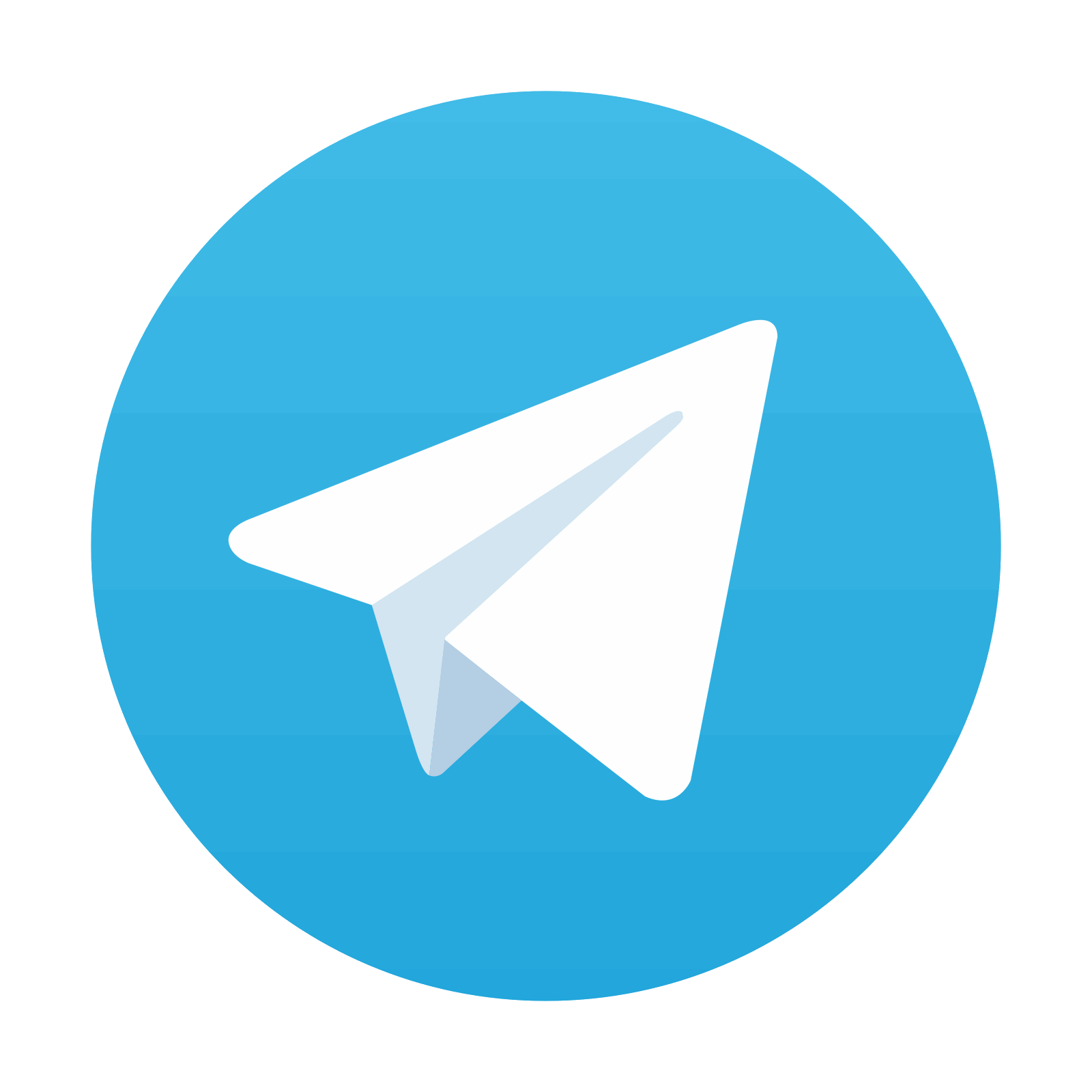
Stay updated, free articles. Join our Telegram channel

Full access? Get Clinical Tree
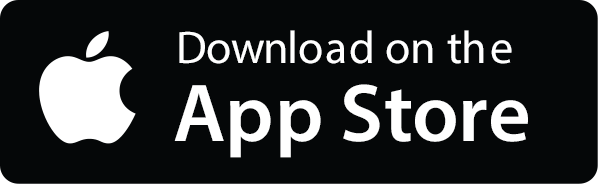
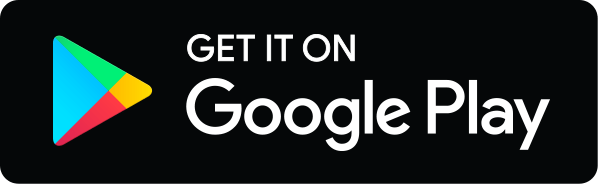
