LEARNING OBJECTIVES
Learning Objectives
The student will be able to define the general contributions of airway design, receptors, reflexes, and secretions in lung defense against microbes and other respiratory particulates.
The student will be able to explain how impaction, sedimentation, Brownian motion, and mucociliary transport act to clear inhaled particles of various sizes.
The student will be able to identify key leukocytes of airways and parenchyma, their roles in recognizing pathogens in proximal and distal airspaces, and their secretions of cytokines, chemokines, and other host defense molecules.
Ventilation of the lung requires the daily inhalation of many thousands of liters of ambient air (Chap. 4), none of which can be presumed to be free of suspended microbes, inorganic particulates, organic fumes, or other noxious gases. Nevertheless, the delicate alveolar parenchyma remains remarkably intact and sterile in most individuals, unless airway defense mechanisms are disturbed. Those mechanisms include physical processes such as sedimentation, as well as biological barriers composed of resident leukocytes that begin in the nares and extend in various forms to, and even through the septal barrier.
NASAL CAVITY AND SINUS DEFENSE MECHANISMS
As described in Chap. 2, the nasal cavities are lined with vibrissae that are interspersed over the distal nasal turbinates, starting at the keratinized epithelial layers that exist inside the nares even before transition to the ciliated respiratory epithelium (Fig. 10.1). With their large size and scattered spacing, these hairs are sufficiently strong to ensnare larger objects that might be inhaled accidentally, such as small insects and large particles of ash. Tactile stimulation of these hairs is often sufficient to induce sneeze, cough, or glandular secretions that collectively act to consolidate and expel such foreign materials. However, they filter few objects that are smaller than the size that can be resolved with the unassisted human eye (~100 μm).
Less obvious are the delicate sensory nerve endings also present throughout the upper nasal passages. Collectively, these afferent fibers serve as irritant receptors capable of responding to both physical deformations, such as inhalation of a gnat, and physico-chemical stimuli like smoke, mineral dust, cold air, capsaicin, and ammonia vapors. Several classes of receptors present in this region send myelinated or unmyelinated fibers to the CNS via the vagus, trigeminal, and perhaps other cranial nerves. Additional details are presented in Chap. 11 about their sensitivity thresholds and their relative rapidity of adaptations to continued stimulation. Activating these receptors can induce bronchoconstriction, coughs, or sneezes that physically propel foreign debris out of the nasal passages. Receptor activation also stimulates serous or mucous glandular secretions, hypo- or hyperventilation, apnea, and potentially a full diving reflex that is particularly prominent in newborn infants and very young children (Chap. 39).
This combination of invoked responses in the nasal cavities to foreign objects or noxious stimuli is typical of reflexes that are present throughout the respiratory system. Virtually every child and adult has experienced the consequences of these responses, such as watery eyes, seasonal rhinitis, sinus congestion, and even emesis that may occur within minutes or hours of exposure to pollen, sawdust, animal dander, and foul odors. Interestingly, natural or pathological variations in the size and patency of the nasal vestibules will make individuals more or less susceptible to these stimuli. For example, deviations in the nasal turbinates or altered sinus drainage can confound the abilities of these defensive reactions to clear the offending stimuli, or drain the secretions they induce. Likewise, there is enormous range in innate or acquired responsiveness among otherwise healthy adults to the antigenicity of certain biological materials such as tree pollens, soil microbes, cats and other pets, or insect feces (see Chap. 21).
DEFENSE MECHANISMS OF THE OROPHARYNX AND UPPER AIRWAYS
Air streams inhaled through the nose or mouth eventually converge in the oropharynx. This makes the ventrolateral location there of the tonsils particularly important, often providing the respiratory system a first opportunity to commence innate immunity or the transition to adaptive immunity. These large, bilateral depots of predominantly lymphoid cells are ideally situated to sample all inspired gases. The tonsils are also continuously exposed to the mixture of normal mouth flora, exogenous microbes, and even refluxed gastrointestinal fluids that bathe the oropharyngeal surfaces. Less conspicuous than the tonsils are the lymphoid aggregates of variable sizes scattered in the lamina propria beneath the oropharyngeal epithelium and along the proximal and some distal airways (Fig. 10.2). These lymphoid aggregates were introduced in Chap. 2 as the mucosa-associated lymphoid tissues (MALT).
FIGURE 10.2
Scanning electron microscopic (SEM) view of the domed surface of a subepidermal lymphoid nodule, here surrounded by stratified squamous epithelium. Such foci are typical of respiratory MALT. Souma T: The distribution and surface ultrastructure of airway epithelial cells in the rat lung: a scanning electron microscopic study, Arch Histol Japon Oct; 50(4):419-436, 1987.
CLINICAL CORRELATION 10.1
The importance of such lymphoid tissues in normal host defense can be illustrated by the example in AIDS patients. Particularly in the early days of the epidemic, virtually all HIV-infected individuals developed oral thrush from oropharyngeal colonization with Candida albicans, due to their impaired mucosal immunity. A much smaller percentage of these AIDS patients ever developed fungal pneumonia, candidemia, or disseminated candidiasis unless they also developed neutropenia for another reason. The pivotal role of polymorphonuclear neutrophils (PMNs) in maintaining sterility of the lung parenchyma is discussed in more detail below.
Inhaled gas or oropharyngeal aspirates that traverse the epiglottis at the larynx and enter the trachea encounter a more elaborate and comprehensive array of host defense barriers. In conventional terms, these include purely physical processes like impaction and sedimentation, as well as cell-mediated mucociliary clearance, phagocytosis, and antigen presentation. Each of these is summarized here in turn.
Impaction reflects the tendency for particles suspended in flight to remain on linear trajectories until an obstacle is encountered. Not surprisingly, the constantly bifurcating airways present such a barrier to deep lung penetration by larger airborne objects that are being inspired at relatively high velocities. Such inhaled particles collide with the apposing airway wall of each Y-shaped branch point, becoming entrapped there in the mucus layer that lines these airways (Fig. 10.3). Modeling studies and experimental evidence suggest that impaction is particularly effective at clearing inhaled particles >5 μm diameter. The subsequent fate of impacted particles is discussed below.
FIGURE 10.3
Clearance of airborne particles from inhaled air by impaction (red circles) and sedimentation (green circles). Very small particles (purple circles) may be cleared by collisions with airway walls, due to their random Brownian motion. These combined effects essentially sterilize inspired gas by the time it reaches the respiratory bronchioles.
Smaller airborne particles (alive or inert) tend to remain suspended during rapid flight, despite the obstacles posed by approaching airway walls. However, these particles still have mass and respond to the effects of gravity, particularly as air speed diminishes with deeper penetrations into the conducting airways. For particles with diameters of 0.5-5 μm, sedimentation is an important clearance mechanism that resembles the way in which fine suspended particulates settle out of slowly moving water (Fig. 10.3). Once smaller particles sediment out and become entrapped in mucus, they also are expelled from the lungs so that the alveolar parenchyma is spared their effects.
Particles <0.5 μm in diameter are not effectively cleared by impaction or sedimentation. However, many of these become embedded in epithelial mucus by random Brownian motion (termed “diffusion” by some authors), notably as airspeed slows even more due to the high resistance to flow in the smallest bronchioles. The net effects of impaction, sedimentation, and such random Brownian collisions are to sterilize the inhaled air of most objects down to the size of bacteria well before this gas enters respiratory bronchioles. Nevertheless viruses, aerosolized molecules like bacterial endotoxins, and organic vapors may persist within the inspired airstream that challenge respiratory system defensive functions within the alveolar parenchyma.
CLINICAL CORRELATION 10.2
The consequences of these defensive processes can be dramatic. A commonly cited “clinical pearl” is that a pneumothorax caused by rupture of the visceral pleura covering the lungs creates a sterile wound. This is because the ambient air that entered the intrapleural space first passed through distal airways like respiratory bronchioles. Examples include spontaneous pneumothoraces that occur due to the rupture of a congenital emphysematous bulla (Chap. 37) or result from using excessive positive airway pressures during mechanical ventilation (Chaps. 28 and 30).
MUCOCILIARY CLEARANCE IN THE PROXIMAL AND DISTAL AIRWAYS
Most inhaled particulates become embedded in mucus secreted by respiratory mucosa. However, adhesion does not in itself protect the lung until this hazardous material is degraded or expelled. A second cleansing process depends upon the ciliated pseudo-stratified epithelium (Fig. 10.4), by which mucus-entrapped material is expelled by the synchronized and retrograde movement of cilia. The importance of ciliary function to host defense is underscored by the autosomal recessive disorder of primary ciliary dyskinesia
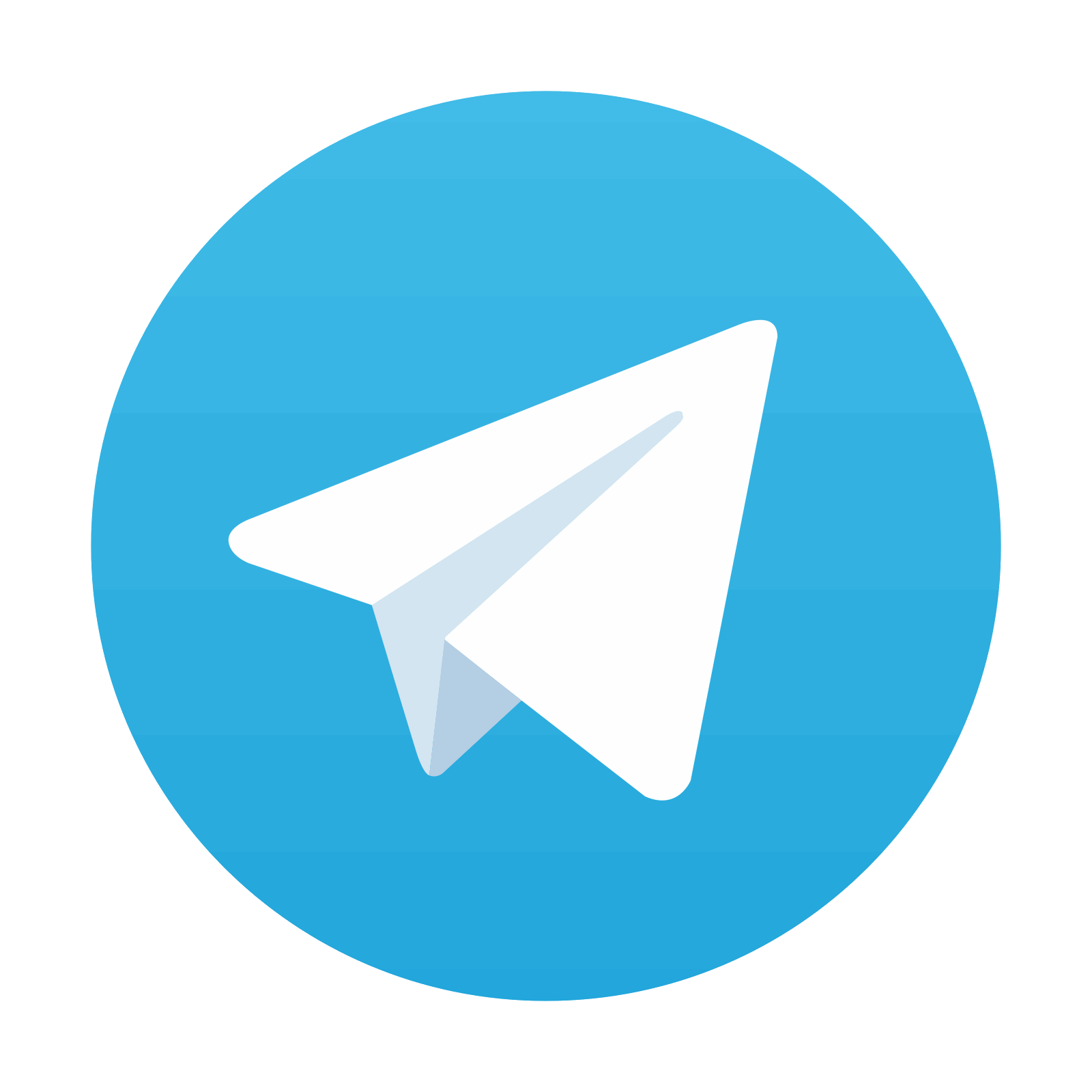
Stay updated, free articles. Join our Telegram channel

Full access? Get Clinical Tree
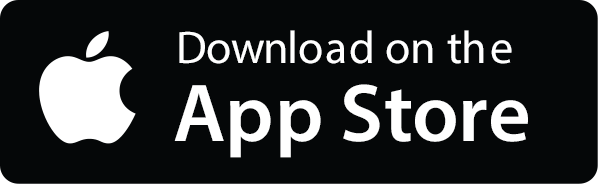
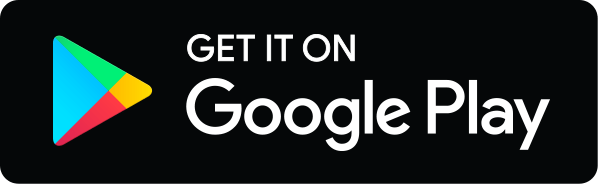
