Abstract
Acute respiratory distress syndrome (ARDS) is the most severe form of acute lung injury, characterized by acute pulmonary infiltrates, abnormal respiratory system compliance, and moderate to severe hypoxemia. The etiology of ARDS is either direct (e.g., pneumonia) or indirect (e.g., sepsis) insult to the lungs by various inflammatory mediators. The definition of ARDS has evolved over time from the earlier description by Ashbaugh in 1967 to a major revision in 1994 by the North American-European Consensus conference committee (NAECC) to the most recent Berlin definition in 2012. In 2015 a panel of investigators recommended adopting the Berlin definition in children (PARDS, pediatric acute respiratory distress syndrome) with some modifications. The pathophysiology is complex with an inflammatory process starting at the alveolar capillary interface with resultant endothelial and epithelial disruption leading to increased alveolar capillary permeability and flooding of the alveoli with protein rich edema fluid. ARDS is a progressive disease with four stages (exudative, fibroproliferative, fibrosing alveolitis, and recovery stage). Not every patient goes through all stages, and whether children go through similar stages to adults is not clear. Despite significant advancement in critical care, mortality in severe ARDS remains high, especially in immunocompromised children. Mechanical ventilation, while protecting the lungs from iatrogenic injuries using lung protection strategies, is the mainstay of treatment of ARDS. The employment of adjuvant treatment with corticosteroids, prone positioning, exogenous surfactant, or nitric oxide administration has not been proven to be beneficial in randomized trials, and these are used in selected patients according to clinician or center preferences. Extracorporeal membrane oxygenation offers hope in certain patients with refractory ARDS.
Keywords
respiratory failure, ARDS, PARDS, respiratory distress, Berlin definition, lung injury, mechanical ventilation
Epidemiology
The available epidemiological studies are based on the former North American European Consensus Conference Committee (AECC) definition of acute respiratory distress syndrome (ARDS) from 1994. The incidence of ARDS in children is 3–12.8/100,000 per year, which is about 5–8 times less than in adults, and constitutes 2%–10% of all pediatric intensive care unit (PICU) admissions per year. Approximately 80% of children with mild ARDS will progress to moderate and severe ARDS, and two-thirds of them will require early invasive mechanical ventilation. About 60% of pediatric patients with ARDS are less than 4 years of age and 30%–70% of them suffer from a significant underline disease (e.g., prematurity, genetic or neurologic abnormality, cardiac disease). ARDS affects males and females equally.
Etiology
A variety of heterogeneous direct (pulmonary ARDS) or indirect (extrapulmonary ARDS) insults to the lungs may provoke a similar cascade of inflammatory reactions, which leads to ARDS ( Box 38.1 ). In case of pulmonary ARDS, the insult is direct in the lungs, and in the case of extrapulmonary ARDS, the insult is indirect and the pulmonary lesions are caused by circulating mediators released from extrapulmonary foci into the blood (e.g., sepsis, pancreatitis; Box 38.1 ). Data provided by Flori and colleagues from a large epidemiological study suggest that pneumonia (bacterial or viral; 35%) and aspiration pneumonia (13%) are the most common causes for direct ARDS, whereas sepsis (13%) is the most common cause for indirect ARDS.
Outcome
The mortality from ARDS among children has decreased significantly from the 65% to 80% reported in the 1980s. This reduction can be attributed to the establishment of dedicated pediatric critical care units with improved general management, including the reduction in the administered tidal volumes (V T ) during mechanical ventilation. However, in the last two decades the mortality has remained unchanged.
A recent large meta-analysis of 32 studies reported a mortality rate of 33.7% in children with ARDS when defined according to the previous AAEC definition of ARDS. It should be noted that studies performed in western countries reported mortality to be between 4.3% and 30.5%, and studies from Asia reported mortality as high as 61%. The differences in mortality among centers are mainly attributed to patient mix and local resources. In general, mortality is higher among children with underlying immune deficiency, malignancy, status post bone marrow transplantation, and with comorbidities (e.g., nonpulmonary organ dysfunction, central nervous system dysfunction) ; it is minimal among children with bronchiolitis (<3%). In general, it is perceived that the mortality in children is lower than in adults, and the overall mortality is 27%–45%.
Only one study thus far has reported mortality using the New Berlin definition of ARDS (see below). This European multicenter study reported mortality rates of 13.9% for mild, 11.3% for moderate, and 25% for severe ARDS; however, it was limited to young children aged 1–18 months.
In contrast to adults and premature infants where the quality of life after respiratory failure was studied, the long-term quality of life and lung morbidity among children who recover from ARDS has not been studied. However, we believe that children without underlying lung or cardiac disease recover from a single episode of ARDS without significant mechanical or functional sequelae to the lungs.
Definition and Diagnosis
ARDS is a form of acute respiratory failure characterized by a diffuse, progressive inflammatory lung disease that was first reported in 1967 by Ashbaugh and colleagues in a cohort of 12 surgical patients. They noted that the patients exhibited “acute onset of tachypnea, hypoxemia and loss of compliance.” Further reports of patients with ARDS altered the acronym to indicate adult respiratory distress syndrome, to differentiate it from the respiratory distress syndrome of neonates, even though Ashbaugh’s original description included an 11-year-old child. It was not until 1980 that regular reports of ARDS in children established that it was also a pediatric syndrome, and the 1994 North American–European Consensus Conference Committee (NAECC) recommended that the acronym “ARDS” revert to its original meaning (i.e., acute). Most studies in ARDS published until 2014 used the AECC definition of ARDS. The diagnostic criteria have been modified in the Berlin ARDS Conference in 2012 ( Table 38.1 ). The Berlin four-point definition eliminated the group of acute lung injury (ALI) and created three ARDS entities—mild, moderate, and severe—according to the severity of hypoxemia extrapolated from the PaO 2 /FiO 2 (P/F) ratio. The new definition, like the previous AECC, focuses on adult patients and does not consider different risk factors, epidemiology, and pathophysiology in children. In addition, the adult definition requires arterial blood gases to calculate the P/F ratio, which are not always available in children.
Timing | Within 1 week of known clinical insult or new or worsening respiratory symptoms |
Chest imaging | Bilateral opacities—not fully explained by effusions, lobar/lung collapse, or Nodules |
Origin of edema | Respiratory failure not fully explained by cardiac failure or fluid overload Need objective assessment (e.g., echocardiography) to exclude hydrostatic edema if no risk factor present |
Oxygenation | — |
Mild | PaO 2 /FIO 2 201–300 mm Hg with PEEP or CPAP ≥ 5 cm H 2 O a |
Moderate | PaO 2 /FIO 2 101–200 Hg with PEEP ≥ 5 cm H 2 O |
Severe | PaO 2 /FIO 2 ≤ 100 mm Hg with PEEP ≥ 5 cm H 2 O |
a This may be delivered noninvasively in the mild acute respiratory distress syndrome group.
In 2015 a panel of investigators proposed adaptation of the Berlin definition to children, the pediatric ARDS (PARDS; Table 38.2 ). The PARDS definition uses the P/F ratio or SpO 2 /FiO 2 ratio for noninvasive ventilated children and the oxygenation index (OI) or the oxygenation saturation index (OSI), when arterial blood is not available, for ventilated children. The request for bilateral pulmonary infiltrates was eliminated, and a better definition for children with underlying congenital heart disease or chronic lung disease was offered.
Age | Exclude patients with perinatal lung disease | |||
Timing | Within 7 days of known clinical insult | |||
Origin of edema | Respiratory failure not fully explained by cardiac failure or fluid overload | |||
Imaging | Chest imaging findings of new infiltrate(s) consistent with acute pulmonary parenchymal disease | |||
Oxygenation | Noninvasive MV Invasive MV | |||
CPAP ≥ 5 cm H 2 O a | Mild | Moderate | Severe | |
P/F ≤ 300 | 4 ≤ OI < 8 | 8 ≤ OI < 16 | OI ≥ 16 | |
SpO 2 /FiO 2 ≤ 264 b | 5 ≤ OSI < 7.5 b | 7.5 ≤ OSI < 12.3 | OSI ≥ 12.3 |
a For nonintubated patients treated with supplemental oxygen on nasal modes of noninvasive ventilation.
b Use PaO 2 when available; if not available, wean the FiO 2 to maintain SpO 2 97% to calculate the OSI or SpO 2 /FiO 2 .
The classical histological description of ARDS was published in 1976 by Katzenstein and colleagues as diffuse alveolar damage (DAD) associated with the presence of hyaline membranes, interstitial edema, cell necrosis with proliferation, and fibrosis. The validity of the Berlin definition has been tested against lung histopathologic findings in adults undergoing autopsy following clinical ARDS. Among all patients who met clinical criteria for ARDS, DAD was found in only 45%. The proportion of DAD depended on the severity of ARDS, and was more frequent in severe ARDS (58%). Using DAD as the reference standard, the sensitivity and specificity of the diagnosis in adults were 89% (95% confidence interval [CI], 84%–93%) and 63% (95% CI, 59%–67%), respectively. There has been no similar study in children. However, we may expect similar findings, as a comparable study that was performed with the old NAECC definition reported a sensitivity of 81% and a specificity of 71%. The main limitation of these studies was selection bias because they were limited to patients who died; survivors may have had different pathophysiologic findings.
Over the years, various single specific biomarkers or panels of biomarkers, such as surfactant proteins, cytokines, and markers of pulmonary epithelial and endothelial injury, have been investigated for the early diagnosis of ARDS or pending ARDS. However, all these attempts failed because of low sensitivity and specificity. We are still in the search for the “troponin of ARDS.”
Pathophysiology
ARDS arises because of an inflammatory process at the alveolar-capillary interface in the lung. The resultant endothelial and epithelial disruption leads to increased alveolar-capillary permeability and flooding of the alveoli with protein-rich edema fluid. Alveolar gas exchange is impaired and surfactant function is disrupted. In addition, there is uncontrolled activation of coagulation along with suppression of fibrinolysis. Classically, ARDS is described as a progressive clinical condition characterized by four stages, although these are rarely identified in pediatric cases. ARDS is triggered by an acute direct injury (e.g., aspiration) or indirect lung injury (e.g., sepsis), resulting in the onset of an acute exudative phase characterized by pulmonary edema, cytokine release, and activation of neutrophils. The acute exudative stage is associated with increased intrapulmonary shunting, increase in dead space, reduced functional residual capacity (FRC), and a decrease in lung and chest wall compliance. Before the advent of computerized tomography (CT), the acute exudative stage was synonymous with the radiologic finding of bilateral homogenous parenchymal disease; however, pulmonary CT imaging changed our outlook on ARDS, revealing heterogeneity in the extent of regional lung injury, with the coexistence of severely injured (dependent) lung and less injured lung and healthy lung regions. Gattinoni and Pesenti suggested that the lung in ARDS be conceptualized as three functional regions: (1) fully aerated normal (usually nondependent) region(s); (2) poorly aerated injured but recruitable lung; and (3) nonaerated consolidated/atelectatic lung. This group also introduced the term baby lung to describe the small volume of normal aerated tissue in the nondependent lung regions of the lung that exhibits normal compliance.
During the acute phase of inflammation, abnormalities in coagulation contribute to the development of small vessel occlusion. In rare cases, pulmonary hypertension, due to vascular obstruction and complicated by right ventricular failure, has been described. The acute exudative phase may resolve within hours or days, and some patients do not progress to the subsequent fibroproliferative early repair phase. Unfortunately, “repair” may not result in disease resolution in all patients, but may presage the development of fibrosing alveolitis characterized by increased alveolar dead space, hypoxia, and reduced lung compliance; progression to this third stage is associated with worse outcome. The fourth stage, the recovery stage, occurs within 10–14 days, with gradual improvement in lung compliance and oxygenation. The mechanism of resolution of the acute inflammatory process and fibrosis is not well established, and the rate of recovery is not well characterized; however, a return to normal lung function in those patients without underlying chronic lung disease, is the norm.
Thille and coworkers examined the histopathological changes in a large adult population with clinical ARDS who demonstrated DAD at autopsy examination. The histological findings closely correlated with the duration of evolution of ARDS. The exudative changes were observed in 90% of patients with ARDS of less than 1-week duration, and decreased over time. The occurrence of proliferative changes increased over time and peaked between the second and third week (78%). Fibrosis was noted rarely in the first week (4%) and after 3 weeks it was noted in 61% of patients. Fibrosis occurred more frequent in ARDS of primary pulmonary origin (direct) than that of nonpulmonary origin (indirect).
A somewhat different approach to the pathophysiology of ARDS was proposed by Albert who suggested that ARDS was mainly iatrogenic and not the result of a primary inflammatory process. He proposed that it is a form of ventilation-induced lung injury where the primary problem is atelectasis induced by surfactant abnormalities, and that mechanical ventilation initiates the inflammatory process. If the proposed scenario is correct, at least some instances of ARDS might be prevented by routinely administering a protective strategy of ventilation with sigh breaths, low V T s, and positive end-expiratory pressure (PEEP), avoiding supine positioning, increasing the frequency of repositioning, using prone or semi-prone positioning, and limiting the use of sedation.
Pulmonary (Direct) Versus Extrapulmonary (Indirect) Acute Lung Injury/Acute Respiratory Distress Syndrome
In the initial description of ARDS, Ashbaugh and colleagues observed that different insults appeared to induce a similar injury to the lung; therefore they postulated that a common mechanism of injury must exist. However, careful observation of the clinical progression and histological lung appearance in patients with ARDS suggested that the pattern of lung injury might depend on the etiology. In 1994, the AECC made a distinction between a direct or pulmonary injury (ARDSp), such as might occur following aspiration pneumonia, and contrasted this pattern with indirect or extrapulmonary (ARDSexp) injury associated with a systemic inflammatory response, such as sepsis or acute pancreatitis ( Table 38.3 ).
Direct (Pulmonary) | Indirect (Extrapulmonary) | |
---|---|---|
Morphology | Consolidation | Atelectasis |
Histology | Epithelial injury, alveolar edema | Endothelial injury, interstitial edema |
Respiratory mechanics | Reduced lung compliance | Reduced chest wall compliance |
RESPONSE TO THERAPY | ||
Inhaled NO | ↑Pa o 2 | →Pa o 2 |
Prone position | ↑Pa o 2 | →Pa o 2 |
Surfactant | Possible improved outcome | No effect on outcome |
Recruitment | →PaO 2 | ↑Pa o 2 |
Data provided by Flori and colleagues suggest that ARDSp constitutes 60% of pediatric ARDS cases, like the reported incidence of ARDSp in adults (47%–75%). The same authors also reported sepsis as the major cause (13%–21%) of ARDSexp in children. Others have reported a higher incidence of sepsis (70%).
The differences between ARDSp and ARDSexp may be most apparent in the acute exudative phase of the disease. ARDSp is characterized by a primary injury to the alveolar epithelium resulting in intra-alveolar edema, and reduced lung compliance with preservation of the chest wall compliance. In ARDSexp, the primary insult is systemic and the major injury is to the capillary endothelium; here the edema is predominantly interstitial, and a greater reduction of compliance occurs in the chest wall. That was recently demonstrated in a study conducted in adults where patients with ARDSp had a significantly higher level of a biomarker of lung epithelial injury (surfactant protein D) and significantly lower levels of biomarkers of endothelial injury (angiopoietin-2, von Willebrand Factor) and inflammatory biomarkers (interleukin [IL]-6 and IL-8) than those with ARDSexp. The gross pathology of the lung in ARDSp suggests predominantly consolidation, and in ARDSexp the striking finding is atelectasis. One of the earliest attempts to characterize ARDS based on the mechanism of injury (i.e., direct vs. indirect), was made by Gattinoni and colleagues, who reported differences in respiratory system mechanics. Subsequently, histologic and morphologic evidence supported the distinction between direct and indirect lung injury. These differences may impact the response to treatment and outcome; indeed, a study in children reported that ARDSexp had a greater mortality than ARDSp. Such differences in functional pathology may explain why in ARDSp there is a greater incidence of refractory hypoxemia and resistance to recruitment maneuvers and prone positioning, but a better response to surfactant administration and inhaled nitric oxide (iNO). In the majority of patients, clinical differentiation between ARDSexp and the ARDSp is simple but of uncertain importance, while in other situations it can be more complex (e.g., trauma complicated by sepsis).
Severity Score
Blood gas analysis, specifically oxygenation, is regarded as the standard for assessment of severity of ARDS. While the P/F ratio provides the threshold value for the definition of ARDS, the (A–a) O 2 difference (difference between “alveolar” vs. arterial P o 2 ) can express the degree of hypoxemia. Both indices should be used with caution because neither incorporates any measure of the respiratory system mechanics nor the ventilatory assistance being provided to the patient. In this regard, the OI = [(mean airway pressure × FiO 2 ) × 100]/PaO 2 attempts to correct that deficiency. However, even this may mislead if the ventilation strategy employed is not optimized, such as inappropriately high or low airway pressures or Fi o 2 in the case of an intrapulmonary shunt, which is minimally responsive to altered Fi o 2 or airway pressure.
Although death in ARDS is usually attributable to multiorgan failure rather than persistent hypoxemia, the severity of oxygenation failure, expressed as the OI, or to a lesser extent as the P/F ratio does correlate with the duration of mechanical ventilation and with mortality in children. In contrast to oxygenation, the ventilation index : [Pa co 2 × peak airway pressure × respiratory rate]/1000 has been employed to reflect the difficulty involved in clearing CO 2 , (i.e., the pulmonary dead space). The magnitude of dead space in the first days of ARDS has been shown to predict outcome in adults and recently in children.
Genetic Modifiers of Acute Respiratory Distress Syndrome
Other than confirmation that high-pressure, high-volume ventilation is injurious to the lung, the history of ARDS research is replete with interventions that failed to demonstrate clinical benefit. These disappointments often occurred against a background of sound physiologic reasoning and encouraging results from animal studies. It appears that part of the problem has been considering ARDS as a homogeneous disease process. It is already known that only a minority of patients exposed to recognized risk factors develop the ARDS syndrome.
Increasingly, there is recognition that heterogeneity exists in ARDS regarding the development and course of the disease, which is dependent on the interaction of the individual and the inciting event. Consideration of patient-derived factors likely to influence the manifestation of an inflammatory process leads inevitably to scrutiny of the genome. In 2002, Marshall and colleagues were the first investigators to describe a preliminary association between a gene variant and ALI mortality. This group described the increased incidence of a high producer polymorphism of the angiotensin converting enzyme gene in patients with ARDS. Since this report, similar candidate gene studies have revealed a growing number of gene polymorphisms associated with the susceptibility to ALI/ARDS or its clinical course, for example, gene encoding: pulmonary surfactant-associated protein B (SFTPB), IL-6, IL-10, tumor necrosis factor (TNF), angiotensin-converting enzyme, coagulation factor V (F5) and others. It is anticipated that an enhanced knowledge of the role of polymorphisms in ALI/ARDS will permit appropriate grouping of patients for investigation and will facilitate personalized therapy.
Differences Between Children and Adults
Compared to adults, children demonstrate a spectrum of lung and chest wall development as maturation of the lung continues until 8 years of age. Infants and young children have fewer alveoli compared to adults (approximately 20 million alveoli after birth compared to 300 million alveoli by the age of 8 years; the size of each individual alveolus is also smaller in children (150–180 µm vs. 250–300 µm diameter). Together, these two anatomic differences markedly decrease the surface area available for gas exchange. This anatomic variation is prevalent until approximately 8 years of age. The mechanical properties of the lungs of children and infants are also different from those of adults. The infant lung has low inherent elastic recoil, which may protect against lung collapse, so lower PEEP levels may be required to maintain lung recruitment. In contrast, the chest wall of an infant is more compliant, providing less opposition to the natural recoil (deflating tendency). The ribs in infants are more horizontally aligned compared to adults and, in combination with a more compliant chest, it is more difficult to generate negative pressure in the presence of poor lung compliance. Chest wall compliance is inversely related to age, and with pressure preset ventilation, higher chest wall compliance may increase delivered V T and thereby increase the risk for ventilator-associated lung injury (VALI) in young children. The ratio of lung volume to body weight is greatest in the first 2 years of life, and this suggests that indexing the V T to body weight may result in a smaller fraction of lung volume being inflated in the young infant compared with the older child. Experimental data indicate that for a given level of inflation, the inflammatory response to either sepsis or high V T , as well as the propensity to structural injury, might be less in infant versus adult lungs. These physiologic differences are important because adult guidelines for lung protective ventilation are predicated on the behavior of a respiratory system that is very different to that of the infant and young child.
Treatment
Multiple clinical trials for ARDS have been performed in the last three decades, nearly all of them in adults; however, only sporadic trials have shown beneficial effects on mortality. Tonelli and coworkers examined 159 randomized, controlled trials (RCTs) and 26 meta-analyses and found a significant effect on mortality in only two trials for two interventions (low V T , and prone positioning). Moreover, it was difficult to consistently replicate these results in other trials. This does not mean that clinical strategies do not affect the outcome of the patients, but it emphasizes the difficulties faced in studying ARDS. The two main factors that explain this are the heterogeneity in study populations and the lack of standardization of outcome measures.
Conventional Mechanical Ventilation
Since the introduction of new definitions of ARDS and PARDS, no good epidemiological data are available regarding the requirement for invasive mechanical ventilation among children with PARDS. Based on older studies, we estimate that about 30% of patients with PARDS do not require invasive mechanical ventilation at the onset of the disease, However, almost half of the patients eventually require intubation and mechanical ventilation for disease progression, and almost all children with severe PARDS require invasive mechanical ventilation.
Substantial clinical evidence indicates that the injudicious use of mechanical ventilation can initiate or exacerbate lung injury and contribute to the mortality associated with ALI/ARDS. Studies in animal models have demonstrated that mechanical ventilation may induce lung injury through physical disruption of the alveoli (barotrauma); overdistension of the lung (volutrauma); recruitment and derecruitment of collapsed alveoli (atelectrauma); activation of the inflammatory process (biotrauma); and possibly, toxicity from high levels of oxygen. Such factors may produce histologic damage and increased permeability of the alveolar-capillary interface, which is indistinguishable from the lesions seen in other forms of ALI.
The ventilation factors associated with this iatrogenic lung injury are elevated levels of end-inspiratory airway pressure, large tidal volumes, low levels of end-expiratory airway pressure, and possibly high Fi o 2 . Understanding the roles of elevated levels of inspiratory pressure and V T from laboratory and clinical studies led to the design of two important outcome studies that compared lower versus higher tidal volumes in patients with ARDS. These studies demonstrated a clear association between higher V T (or inspiratory pressures) and worse outcome. This approach, along with the belief from laboratory and clinical trials that higher levels of PEEP were protective, led to a gradual adoption of the idea that lung protective ventilation, lower tidal volumes, recruitment, and higher levels of PEEP would ensure sufficient gas exchange while minimizing lung injury. Hence, we now employ lower V T , up to 10 mL/kg; lower inflation pressure (<30 cm H 2 O plateau pressure); higher PEEP (~5 to 15 cm H 2 O), and lower Fi o 2 than two decades ago. It should be noted that the plateau pressure limitations do not consider differences in chest wall compliance between patients. To take this variable into account, one would need to measure the transpulmonary pressure (esophageal pressure). The physiologic goals of mechanical ventilation are not to attempt to achieve normal blood gases values without regard for the V T , Fi o 2 , or inflation pressures delivered to the patient. Mechanical ventilation is employed with consideration of a risk/benefit estimation for each patient. If the achievement of normal pH, Pa co 2 , and Pa o 2 levels requires respiratory support strategies that may injure the lungs, then lower pH, Pa o 2 , and higher Pa co 2 (permissive hypercapnia) are tolerated. Despite the lack of data regarding the effect of relative hypoxia on human organ systems in general, and on the developing infant brain, the maintenance of Sa o 2 >90% (Pa o 2 60–80 mm Hg) using the lowest oxygen concentration necessary to achieve that result is considered by most pediatric intensivists as an optimal approach. Because the likelihood of lung injury is greater if the airway pressure, V T , and concentration of inspired oxygen are elevated, many clinicians will reduce the target SaO 2 (85%–88%) if necessary. Permissive hypercapnia (Pa co 2 60–80 mm Hg) with a pH >7.2 appears to be well tolerated by most children (although it is avoided in the presence of raised intracranial pressure or pulmonary hypertension). The “protective” approach has not been validated in children, and the mechanical ventilation strategies used in children mirror the recommendations of the adult critical care community. This approach is physiologically sound, but given the increasingly recognized heterogeneity of ARDS, it is likely that some subtle disease features peculiar to pediatrics have been ignored.
Few studies of mechanical ventilation have been performed in children, and, as a result, no specific approaches have been proven superior to others. Indeed, most approaches for mechanical ventilation in children have been extrapolated from adult studies. Currently there are no data to support the superiority of one mode of ventilation over another (e.g., pressure control vs. volume control). However, advocates of pressure control ventilation argue that it is more physiologic because of the decelerating flow, and that the V T generated during each breath better reflects dynamic lung characteristics. Interestingly, two surveys reported that clinicians demonstrate a preference for pressure targeted ventilation and pressure regulated volume control (PRVC) ventilation when treating children.
Other Modalities of Ventilation
Noninvasive Ventilation
To limit complications associated with endotracheal intubation, noninvasive ventilation (NIV) has been gaining increasing attention in the last few years and it is the focus of several studies. NIV may be administered with positive or negative pressure ventilation and, more recently, through high-flow nasal cannula (HFNC). Since no data are available on the role of negative pressure ventilation in PARDS; we focus here on the use of noninvasive positive pressure ventilation (NIPPV) and HFNC. The role of NIPPV in the pediatric population is not fully characterized and only one RCT in children with acute respiratory failure has been published. In that study of 50 children with acute hypoxemic respiratory failure, NIPPV was compared with standard care. The authors found that the frequency of intubation was significantly lower in the group that received NIPPV (28% vs. 60%). A trial of NIPPV may be attempted in any child with early respiratory failure and mild to moderate PARDS; especially in children with immunodeficiency who are at greater risk of complications from invasive mechanical ventilation. However, one should not persist with its use if there is no clinical benefit within a short time. It appears that patients who are hemodynamically unstable, have a greater severity of illness, or have moderate to severe PARDS are less likely to benefit from NIPPV. Almost any ventilator may be used to provide NIPPV in volume, pressure modes, or a bilevel controlled or continuous positive pressure (CPAP) device can be used. Recently it was reported that the use of neurally adjusted ventilator assist (NAVA) mode resulted in a dramatic reduction in patient-ventilator asynchronies and in trigger delay compared with the pressure control mode of ventilation in infants with severe bronchiolitis. NIPPV may be administered using facial, oronasal or nasal masks. Compared with oronasal masks, nasal masks are better tolerated in younger children. However, nasal interfaces are associated with significant more air leaks and patient-ventilator asynchrony. In contrast, the full facemask is less tolerated and increases the risk of aspiration. In addition, both interfaces do not permit pressures greater than 20 cm H 2 O on an ongoing basis.
In the last 5–10 years, HFNC replaced the traditional nasal cannulas for oxygen administration and the “conventional” NIPPV. The old nasal cannulas used flows of 0.5–4 L/min of dry unheated gas. In contrast, HFNC consists of an air–oxygen blender, which delivers heated humidified gas through nasal prongs with flows as high as 60 L/min. It has been proposed as an alternative to CPAP and “conventional” NIPPV.
Most of the studies on the use of HFNC in infant and children are small observational studies, which have shown beneficial effects in bronchiolitis. Other studies show that the system is well tolerated, safe, and reduces the breathing effort. However, the potential benefit in reducing the rate of intubation in PARDS has not been studied. In a recent RCT trial in adults with acute hypoxemic respiratory failure, treatment with high-flow oxygen, standard oxygen administration, or conventional NIV did not result in significantly different intubation rates.
The mechanism of action of HFNC is not well understood and includes (1) the washout of nasopharyngeal dead space, resulting in increased FiO 2 and reduced carbon dioxide in the dead space; (2) the reduction of inspiratory resistance; and (3) the reduction of the metabolic cost of gas conditioning by providing air with 100% relative humidity and providing an end-expiratory positive pressure.
High-Frequency Oscillatory Ventilation
A variety of high-frequency ventilation devices are available for clinical use; however, high-frequency oscillatory ventilation (HFOV) has achieved the greatest acceptance in neonatal, pediatric, and adult critical care practice. According to several studies, between 5% and 52% of children with acute respiratory failure are ventilated using HFOV at some stage during their disease. HFOV achieves effective gas exchange while avoiding high peak airway pressures and the inflation–deflation cycles characteristic of conventional ventilation. Lung volume (and hence oxygenation) is maintained by the application of a high continuous mean airway pressure. CO 2 removal is achieved despite small tidal volumes (2–4 mL/kg) by imposing a breath frequency of 300–900 (5–15 Hz) per minute, resulting in large minute volumes. Therefore HFOV involves the application of the open lung strategy accompanied by small swings in pressure and volume in the distal airways and alveoli. Hence, the cyclical application of high distending airway pressures (barotrauma) and the associated cyclical delivery of large tidal volumes (volutrauma) are avoided. In animal models of ALI/ARDS, HFOV has demonstrated comparable or superior gas exchange when compared with conventional ventilation while producing less lung injury. HFOV would appear to represent an optimal lung protective ventilatory device; however, with the progress in protective conventional mechanical ventilation (CMV), it is being deployed only when CMV fails. Although there are no established criteria to define CMV failure, in general HFOV is considered when, despite a plateau pressures of 30–32 cm H 2 O and Fi o 2 > 0.6, there is inadequate oxygenation and/or significant hypercapnia. Two studies comparing HFOV to CMV in children with respiratory failure were published recently. The first study was a secondary analysis of a sedation study, compared the early (24–48 hours) application of HFOV in 181 children to 883 children that received CMV and/or late application of HFOV. The early application of HFOV was associated with a longer duration of mechanical ventilation, but the mortality was similar in the two groups. The second study was a retrospective study that included 1764 patients in 98 hospitals. It showed that early or late application of HFOV was associated with increased mortality (17.3% vs. 8.4%), increased duration of mechanical ventilation, and increased length of stay in the intensive care unit (ICU). The results of these studies are similar to two recently published RCTs in adults, where the early application of HFOV in unselected etiologies of ARDS showed no effect, or an increase in mortality (47% vs. 35%). A different study in adults, in a selected group of patients with severe pulmonary ARDS, hypercapnia, and acidosis, showed a rapid and persistent reduction in CO 2 without adverse effect.
This supports the argument that a trial of a therapeutic intervention in a heterogeneous disease like ARDS should be examined only in selected, well-defined groups of patients.
Airway Pressure Release Ventilation
Airway pressure release ventilation (APRV) is a ventilator modality characterized by cyclical alternation between two levels (high and low) of positive airway pressure, while permitting spontaneous breathing activity at both levels of pressure support. Typically, 80%–95% of the ventilatory cycle is accounted for by the higher pressure (P high , usually 15–30 cm H 2 O) and is only briefly interrupted by drops to a lower level of airway pressure (P low , usually 0–15 cm H 2 O). The duration of both the P high and P low periods are time cycled and continue even when spontaneous breathing is not detected. In such a case, APRV will resemble inverse (inspiratory: expiratory) ratio pressure-control ventilation with a very long inspiratory time. APRV, facilitates an open lung ventilatory approach, avoids cyclical recruitment and derecruitment of alveolar units, permits homogenous gas distribution during inspiration, minimizes overdistension of healthy lung (volutrauma), and reduces the risk of low-volume lung injury (atelectrauma). There have been few studies of the role of APRV in pediatric and adult ARDS. It has been shown that in children with mild to moderate lung disease, APRV provides good efficacy in ventilation and oxygenation compared with conventional ventilation with significantly lower peak and plateau airway pressures. Despite the potential benefits of APRV, it is not a common modality of ventilation in children. A recent survey of the daily practice of mechanical ventilation among 18 medical centers in Italy reported that less than 4% used APRV.
Neurally Adjusted Ventilatory Assist
Patient-ventilator dyssynchrony is a major issue in the delivery of mechanical ventilation to patients capable of spontaneous breathing. More than 25% of adult ventilated patients exhibit dyssynchrony. Key considerations in patient-ventilator synchrony include signaling of the onset of the patient’s inspiratory effort, the pressure/flow profile of gas delivery during the breath, and mechanisms to signal termination of the patient’s inspiratory effort. NAVA is a relatively recent innovation to improve such synchrony in invasive and, more recently, in noninvasive ventilation. The NAVA system utilizes the electrical activity of the diaphragmatic (right crural) muscle to signal the initiation of patient inspiratory effort. The signal is detected using electrodes embedded in the distal end of a gastric tube that is positioned astride the esophageal hiatus of the diaphragm. Because muscle electrical activity is proportional to the strength of the phrenic signal (which itself reflects the respiratory demands of the patient), the NAVA system offers both improved triggering and variable ventilatory support proportionate to the patient’s respiratory demands. This is an improvement over circuit pressure or gas flow triggering systems and the use of a fixed amount of ventilatory support with each breath. There are only few reports of NAVA use in either adults or children. It has been demonstrated that NAVA prevents excessive lung distension, efficiently unloads respiratory muscles, and improves, but does not completely abolish, patient-ventilator dyssynchrony. The penetration of this modality of ventilation in pediatric critical care units is unknown; however, we speculate that it is very low, as most surveys do not mention it.
Adjuvants to Mechanical Ventilation
Prone positioning, surfactant, and nitric oxide administration have all been employed as adjuvant therapies in ventilated patients with ALI/ARDS. To date, none of these interventions has demonstrated an ability to improve patient outcome except for prone positioning in adults when employed as a routine part of care.
Corticosteroids
The use of corticosteroids for the treatment of ARDS was suggested by Ashbaugh in 1967. Almost 50 years and several clinical trials later, it is still the subject of debate. ARDS is characterized by inflammation that may resolve within a short period; alternatively, it may progress to the unresolving inflammatory (i.e., fibroproliferative) stage. The antiinflammatory properties of corticosteroids and the potential inhibition of both fibroblast proliferation and collagen deposition make corticosteroids an attractive option.
The use of corticosteroids has been examined (in adult patients) in different disease stages, including prophylactically (before development of ALI/ARDS), during the early stages of disease (<7 days), and in later stages of the disease (>7 or 14 days). Corticosteroids have been used for different durations and at different doses. Early studies focused on the prevention of ARDS after events that are known to potentially lead to ARDS (e.g., sepsis). High doses (≥30 mg/kg/day) of corticosteroids for a short period of time (≤24 hours) either had no impact on mortality or, in one case, increased mortality. A relatively recent study of early low-dose corticosteroids (1 mg/kg/day) for 25 days demonstrated a treatment benefit with improvements in lung injury score, days of ventilation, and mortality. However, methodological issues provoked significant controversy. Several studies that examined the role of corticosteroids late in the course of the disease (>7 days) reported contradictory results, and there is some evidence that steroids introduced after 2 weeks of illness may result in increased mortality. An examination of adult patients with sepsis-induced ARDS who were nonresponders to adrenocorticotropic hormone (ACTH) stimulation testing demonstrated improved lung function and decreased mortality with low-dose steroid use in early disease. This study underscores the importance of selecting groups likely to benefit from steroids when studying their potential use in ARDS. A recent analysis of patient data from four randomized trials demonstrated that early and prolonged glucocorticoid treatment accelerated resolution of ARDS and decreased mortality without increasing the risk of infection. In children, only a few case series are available. Recently, it was reported in an observational single study that corticosteroid exposure (>24 h) in children with PARDS is associated with fewer ventilation-free days after adjustment for key potential confounders, including severity of illness, OI, immunocompromised status, and number of organ failures.
In summary, current evidence from clinical trials does not support the use of corticosteroids in any phase of ARDS in adults. Further investigations are required to address the paucity of data in children and to specifically identify potential responders among the population of ARDS patients.
Clinicians should remain vigilant for steroid-responsive diseases, such as acute eosinophilic pneumonia, hypersensitivity pneumonitis, diffuse alveolar hemorrhage from vasculitis, nonspecific interstitial pneumonitis and others, which may present as PARDS.
Prone Positioning
Prone positioning is safe and has been reported to produce a rapid and sustained improvement in arterial oxygenation in 90% of children with PARDS. This response rate was superior to that reported in adults (60%). However, the improvement in oxygenation did not translate into a reduction in days of ventilation or patient mortality in children. Prone positioning is best reserved for patients with persistent refractory hypoxemia when acceptable oxygenation cannot be achieved within the parameters of a lung protective ventilatory strategy. However, where it does not improve oxygenation, it should be discontinued. A multicenter comprehensive study reported that 17.6% of children with ALI/ARDS receive prone positioning as part of their management at some time during their disease.
Inhaled Nitric Oxide
Nitric oxide is a potent short-acting selective pulmonary vasodilator. It has been shown to result in short-term improvements in oxygenation in some patients with ARDS/ALI, but it has no substantial impact on the duration of ventilator support or mortality when used as a routine part of care. Currently, only a minority (12%) of children with ALI receive iNO during the disease. The routine use of iNO is not recommended, but it may be considered in a selected group of patients with pulmonary hypertension or significant right ventricular dysfunction. In addition, it may be considered for patients with refractory hypoxemia, with Fi o 2 >0.6, and in whom a trial of iNO demonstrated benefit. Where inhaled iNO is being used, some recommend that a daily trial be conducted to ensure that the minimal effective dose is being used, because it has been shown that the iNO dose can be reduced during the course of the disease.
A recent RCT in adults with moderate to severe ARDS demonstrated significantly better pulmonary function tests 6 months after treatment among adult patients treated with a low dose of iNO (5 PPM) compared to similar patients who received placebo. The potential long-term protective role of iNO in patients with ARDS or PARDS should be explored.
Surfactant
The potential role of surfactant dysfunction in the pathogenesis of ARDS was suggested by Ashbaugh. The concept of surfactant administration to patients with ARDS is very attractive, especially when seen in the context of its benefits in infant respiratory distress. However, at this stage, routine surfactant administration is not recommended. Comparison of results among studies is complicated by the differences in surfactant preparations and delivery methods. Walmrath and colleagues reported improvement in oxygenation when a high dose of bovine surfactant was administered to patients with ARDS and sepsis. Spragg and colleagues reported transient improvement in oxygenation when recombinant surfactant was administered to adult patients with ARDS in a phase III trial; in a post hoc analysis, they also reported a potential improvement in mortality in ARDSp but not in “extrapulmonary” ARDSexp. In children, a relatively small RCT reported a reduction in mortality (19% in the surfactant vs. 36% in the placebo group) following administration of natural calf surfactant ; however, there was an unequal distribution of immunocompromised patients among the groups, and controlling for immunocompromised state in multifactorial analysis rendered no difference in mortality between the two treatment groups. Furthermore, there was no effect on ventilator-free days or length of hospitalization. In summary, the routine administration of surfactant in children with PARDS is not recommended. Whether the lack of effect on outcome is a matter of surfactant preparation, patient selection, or method of administration is unclear. However, the transient improvement in oxygenation associated with surfactant administration is tempting, and a survey from 59 centers indicated that more than 50% of intensivists administer surfactant to selected patients with PARDS.
Neuromuscular Blocking Agents
In contrast to paralyzed patients, where it has been shown that most of the tidal breath is delivered directly to the nondependent regions, during spontaneous ventilation, tidal breathing is directed to the dependent better perfused regions, thus reducing dead space and shunt. Therefore until recently, maintaining spontaneous breathing has been recommended in ventilated patients with ARDS. A relatively recent RCT in adults with severe ARDS reported that continuous neuromuscular blockade (NMB; with cisatracurium besylate) during the first 48 hours of ventilation in severe ARDS significantly reduced mortality. Mortality at 90 days in the NMB group was 32% compared with 41% in the controls. This followed earlier mechanistic studies demonstrating that administration of NMB for the first 48 hours of ventilation improved oxygenation and lowered inflammatory markers (IL-8 and IL-6) in the lung bronchoalveolar lavage [BAL]) and serum of patients with ALI/ARDS.
The mechanism of benefit from NMB is speculative. Paralysis of ventilated patients may facilitate protective ventilation through optimization of patient/ventilator synchronization through more accurate adjustment of V T , or by reduction in inspiratory plateau pressure (because of the reduction in chest wall compliance). Moreover, the use of NMB may reduce the oxygen consumption, which, in turn, will reduce cardiac output and lung perfusion, and may reduce lung injury.
A recent study in 23 children with respiratory failure reported that short-term NMB resulted in a significantly improved OI in all patients while the distribution of V T and regional lung filling characteristics were unaffected. No data are available regarding the prevalence of use of neuromuscular blocking agents among children. Until more pediatric data are available, the use of NMB should be reserved for patients in whom sedation alone is inadequate to achieve effective mechanical ventilation.
Beta-Adrenergic Agonists
Alveolar edema in ALI/ARDS is mainly due to increased permeability and, to a lesser extent, to increased capillary hydrostatic pressure. The resolution of alveolar edema is critical to recovery from lung injury. β 2 agonists may reduce alveolar edema by several mechanisms. Alveolar fluid clearance is enhanced through upregulation of Na + transport in the alveolar epithelial cells by β 2 agonists. In addition, pulmonary vasodilatation and the resulting reduction of pulmonary vascular pressure results in lowered capillary hydrostatic pressures, and β 2 agonists may independently decrease endothelial permeability.
An RCT in a small number (40) of adult patients demonstrated that intravenous (IV) salbutamol (albuterol) reduced the extravascular lung water content and, perhaps as a result, lessened the inspiratory airway pressures required for ventilation. An observational review of children with ARDS suggested that inhaled bronchodilators were associated with a lower mortality. Whether inhaled or IV treatment may affect outcome in patients with ARDS is still to be established in an RCT. However, the current evidence discourages the use of β 2 agonists in ALI/ARDS patients.
Extracorporeal Life Support in Pediatric Acute Respiratory Distress Syndrome
Although pediatric extracorporeal life support (ECLS) is predominantly employed for cardiovascular support, it may have an important role in some pulmonary cases, especially where ARDS results from trauma. For pure respiratory failure in the absence of cardiovascular compromise, venovenous cannulation is appropriate. This provides oxygenated blood into the right ventricle, but does not augment cardiac output. In contrast, venoarterial cannulation, providing oxygenated blood into the aorta under pressure, effectively augments the cardiac output while concomitantly increasing systemic oxygenation.
The survival rate (60%) for ECLS in ARDS has not substantially changed in the last 15 years, in contrast to the improved outcomes associated with conventional management. Because of the improvement in outcomes associated with conventional ventilation, and because ECLS is used only when conventional ventilation has “failed,” the efficacy of ECLS may be progressively underestimated because of “referral bias” of progressively more futile cases. Currently, there are no nationally agreed criteria for ECLS in PARDS, and its use is mainly decided by the center. The two potential major criteria for severity of PARDS and ECLS criteria are OI and the P/F ratio. The consensus at this stage is that ECLS may be considered in severe PARDS with persistent inadequate gas exchange (mainly hypoxia), and where the cause of respiratory failure is potentially reversible.
Tracheostomy
Ventilation via a tracheostomy tube has many purported benefits in facilitating weaning from mechanical ventilation: it improves patient comfort, reduces the work of breathing, facilitates bronchopulmonary toilet, reduces the incidence of pneumonia, and improves airway security. In addition, it may reduce the length of stay in the hospital or ICU, and reduce hospital costs, but most importantly, a tracheostomy facilitates patient weaning from mechanical ventilation and lowers patient mortality. For these reasons, tracheostomy has become almost routine in adult critical care, with around 8%–25% of all ventilated adult patients undergoing the procedure during their stay. Considering tracheostomy in an adult patient who is expected to require prolonged ventilation (>14 days) is recommended. In contrast, there is no literature describing the preemptive use of tracheostomy in children in whom prolonged ventilation is anticipated. The prevalence of tracheostomy among children requiring prolonged mechanical ventilation was reported in a limited number of studies and varied from less than 1.5%–2%. The differences in prevalence between adult and children may be explained by the faster resolution of ARDS in children compared with adults, as well as the introduction of a percutaneous dilatational tracheostomy technique, which is performed at the bedside in adults. However, this technique is not suitable for children younger than 12 years of age, and tracheostomy is perceived as an aggressive procedure with high complication rates in children. A recently published study revealed a significant risk for morbidity and mortality among children under the age of 2 years undergoing tracheostomy.
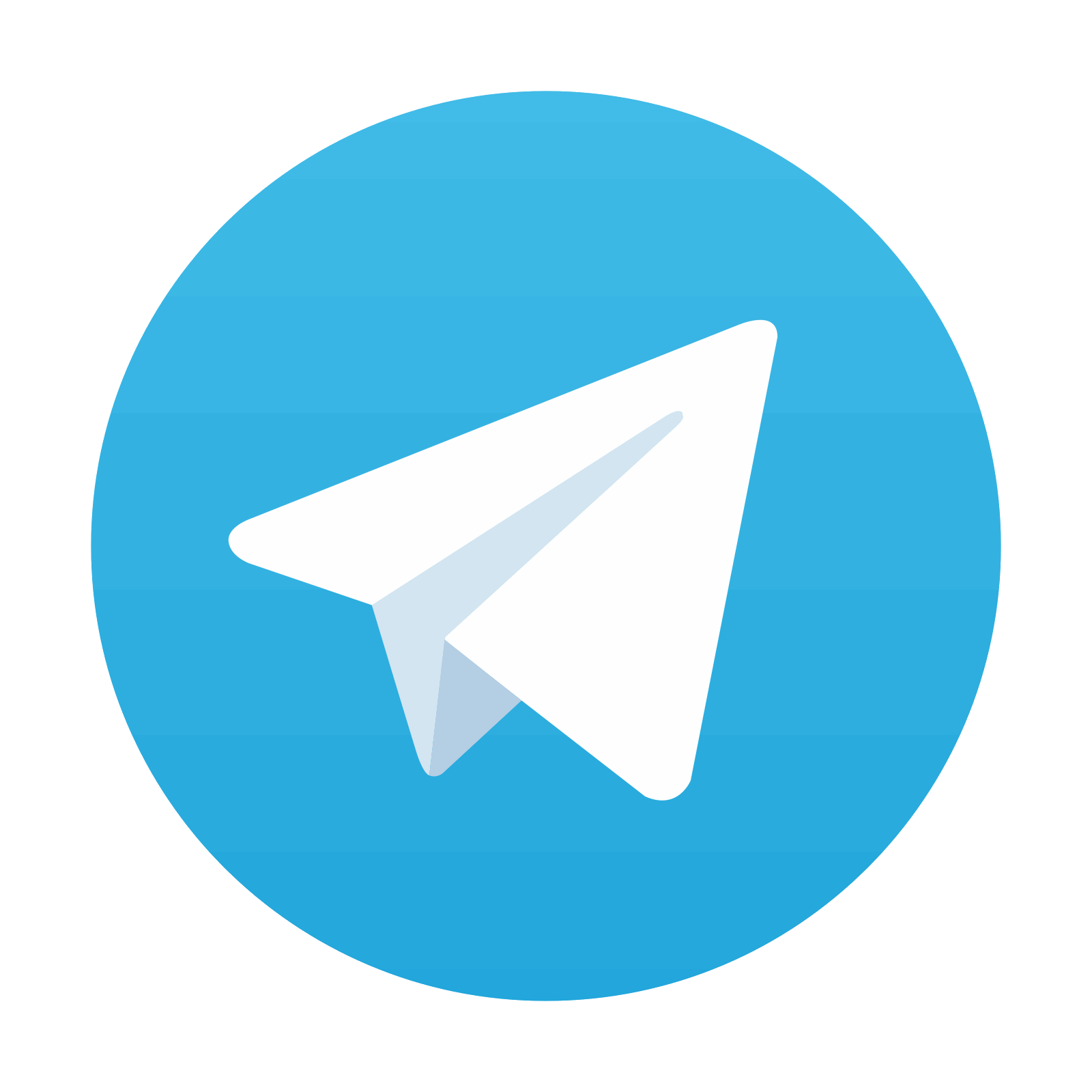
Stay updated, free articles. Join our Telegram channel

Full access? Get Clinical Tree
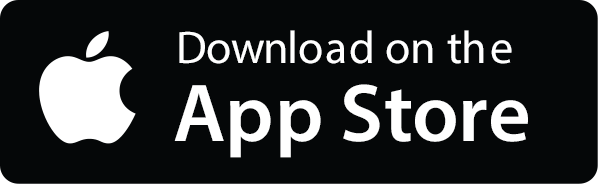
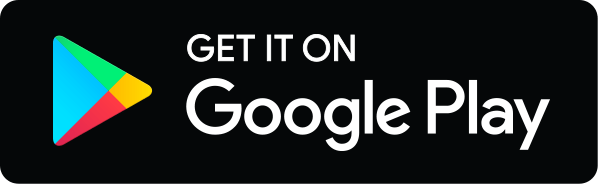