Chapter 46 Acute Arterial Occlusion
Epidemiology of Acute Limb Ischemia
Acute limb ischemia is a rare vascular event, and its incidence eludes exact quantification. It has been influenced by the ever-changing medical landscape. Increasing numbers of patients treated with antiplatelet and antithrombotic therapies, effective therapy for atrial fibrillation, and advances in treatment of valvular and ischemic heart disease have had an impact on the incidence of acute limb ischemia by decreasing the number of embolic events. This may be counterbalanced by increasing numbers of patients undergoing elective surgical and endovascular revascularization therapies, which carry a low but measurable risk of graft or stent thrombosis. An estimate in the 1990s proposed that a vascular center serving a community of 500,000 may expect an annual incidence of 75 patients with acute limb ischemia of the lower extremity.1 Other studies report similar data, estimating the incidence of acute lower-limb ischemia between 13 and 17 cases per 100,000 people per year, with mortality as high as 18% even in the modern era.2,3 Contemporary studies report amputation rates as high as 13% in patients presenting with acute limb ischemia of the lower extremity.4
Acute nontraumatic ischemia of the upper extremity is even more uncommon. It is less likely to result in limb loss, and thus its importance has been overshadowed by lower-extremity ischemic syndromes. Few published series have been reported, and there are no randomized trials evaluating this clinical syndrome and its treatment. However, the consequences of functional impairment in the upper extremity can be equally devastating to the patient.5 On average, acute arm ischemia accounts for 16.6% of cases of acute ischemia of the extremities and, by extrapolation, occurs with an incidence of 1.2 to 3.5 cases per 100,000 per year.6 However, these estimates are based primarily on surgical series, which usually include only patients who underwent surgical treatment. Surveys of all patients presenting with acute arm ischemia estimate an incidence of 1.13 per 100,000 per year.7 In the absence of more meticulous population studies, the true incidence can only be estimated. Patients with upper-extremity ischemia tend to be older than those with lower-extremity ischemia, with mean ages of 74 and 70 respectively.8
Amputation-free survival is influenced by many modifiable and nonmodifiable factors.9 Among the former, delay in diagnosis stands out as a major factor. Non-Caucasian race, older age, malignancy, congestive heart failure (CHF), and low body weight decrease, whereas systemic atherosclerosis increases the likelihood of amputation-free survival.9,10 Among patients older than 75 years of age, overall 30-day mortality rates approach 42%.11 Survival and functional recovery among patients with acute limb ischemia are directly related to underlying comorbidities and delay in diagnosis and treatment.
Etiology of Acute Limb Ischemia
Acute Upper-Extremity Ischemia
The most common sites of arterial occlusion in the upper extremity are the brachial and axillary arteries, representing 85% of cases of embolic occlusion.6 The subclavian artery is thought to be the most frequent site of occlusion in uncommon cases of in situ thrombosis (Fig. 46-1).
Iatrogenic causes
In the past, acute ischemia of the arm was primarily caused by cardiac catheterization performed via brachial artery access (Fig. 46-2). In a series from the 1980s reporting on 37 cases of acute arm ischemia treated surgically over a period of 5 years, 56% of cases were caused by this iatrogenic complication, 24% were related to embolic events, and the remainder were due to stab wounds.12 Since brachial artery catheterization fell out of favor, causes of upper-extremity ischemia have changed. In a later series of 65 patients with acute arm ischemia treated surgically over a span of 8 years, a cardioembolic source was identified in 41% of patients, 17% of events were attributed to an arterial source of embolism, and 28% of cases were related to iatrogenic occlusion, mainly a result of cardiac catheterization.13 The resurgence of interest in radial artery access for coronary procedures is unlikely to result in a rise in frequency of upper-extremity ischemia. Occlusion of the radial artery, seen in up to 5% of procedures, is unlikely to compromise perfusion of the hand in a patient with proper preprocedural assessment of a patent palmar arch.
Embolism
Embolic occlusion is the most frequent cause of acute arm ischemia, accounting for 74% to 100% of cases in several reported series.6,7,13–15 Of these, 72% are thought to be cardioembolic in origin, 12% originate from the proximal vessel, and the remainder are of unknown origin.6 Atrial fibrillation and left ventricular (LV) thrombus in patients with ventricular dysfunction are the most frequent causes of cardiac emboli. Common causes of embolization include atrial myxoma16,17 and paradoxical embolism.18 Proximal arteries of the arm can be a source of arterial embolism. Artery-to-artery embolization may cause occlusion of a large- or medium-caliber artery but more commonly presents with digital embolization. Atherosclerotic stenosis of the subclavian artery is a rare cause of embolism but can result in acute hand or arm ischemia.19–21 The rare primary subclavian artery aneurysm or one caused by external compression in a thoracic outlet syndrome (TOS) can result in thromboembolic occlusion of upper-extremity arteries.20,22–24 Aortic arch atheroma has also been implicated as the source of acute arm ischemia.15,25 Other rare arterial sources of embolic events are malignant emboli or paradoxical embolism through intracardiac shunting26,27 (Fig. 46-3).
Thrombosis
Atherosclerotic disease is much less frequent in the upper extremity than the lower limb. Consequently, in situ thrombosis is uncommon and has been estimated to account for 5% of ischemic cases in population studies and 5% to 35% of cases in surgical series.7,14,15,28 Many of the proximal arterial lesions responsible for distal embolization can cause in situ thrombosis. Arteritis, radiation injury, and hypercoagulable syndromes have been reported as rare causes of in situ arterial thrombosis of the upper extremity.20,22,29,30
Acute Lower-Extremity Ischemia
The distinction between embolism and in situ thrombosis should not detract from the need to establish a rapid diagnosis and institute immediate therapy. Nevertheless, embolic etiology is more commonly associated with rapid onset of symptoms, history of cardiac disease, and absence of prior history of PAD. The contralateral limb is likely to have a normal exam, without stigmata of systemic atherosclerosis. Some of the causes of acute limb ischemia are listed in Box 46-1.
Box 46-1 Causes of Acute Limb Ischemia
In Situ Thrombosis
In situ thrombosis
In situ thrombosis rather than embolism was responsible for 85% of the acute limb ischemia cases enrolled in the Thrombolysis or Peripheral Arterial Surgery (TOPAS) trial. Rates of embolic cases have been decreasing over the last few decades. In a Greek study evaluating the causes of acute limb ischemia at a referral center between 2000 and 2004, 40% of cases were caused by embolic events, in situ thrombosis was responsible for 50% of cases, and the remaining 10% were due to trauma, iatrogenic injury, vasculitis, or dissection.31 As many as 78% of embolic events were due to a cardiac source; the source of 9% of embolic events could not be determined. Among cases of in situ thrombosis, 30% involved native arteries, and 70% involved thrombosis of vessels after an intervention (65% represented graft thrombosis and 5% iliac or infrainguinal stent thrombosis). Surgical graft thrombosis represented 30% of all cases of acute limb ischemia. Patients with surgical grafts can develop graft thrombosis and symptoms of acute limb ischemia due to graft degeneration or mechanical problems such as anastomotic stenosis or retained valves. Graft compression or kink can also cause its thrombosis. With the advent of stent grafting for aortoiliac aneurysmal disease, acute stentgraft thrombosis has been added as a cause of acute limb ischemia (Fig. 46-4).
In situ thrombosis of a popliteal artery aneurysm usually presents with acute limb ischemia. A review of nearly 900 patients presenting with acute limb ischemia secondary to a thrombosed popliteal aneurysm reported amputation rates of 14%. In this study, catheter-directed thrombolysis prior to surgery did not lower the likelihood of amputation, but it significantly improved the long-term patency of the graft, presumably by maximizing patency of the tibial vessel.32 The decision to perform catheter-directed thrombolysis must depend on the clinical situation and urgency of revascularization. In a Swedish vascular registry, amputation rates for acute thrombosis of the popliteal aneurysm were 17% in patients presenting with acute ischemia and only 1.8% for asymptomatic electively repaired aneurysms.33
Embolism
Acute limb ischemia is often caused by an embolic event, commonly from a cardiac source. The embolus most frequently lodges in the aortoiliac bifurcation, femoral bifurcation, or popliteal trifurcation. Over the last several decades, the etiology of cardioembolic events has evolved. Embolic events caused by rheumatic mitral stenosis with left atrial enlargement have become a rare occurrence because the prevalence of rheumatic valve disease has decreased substantially. Age-related atrial fibrillation and LV dysfunction with apical thrombus formation are the most common causes of cardioembolic events (Fig. 46-5). Less common causes include endocarditis, intracardiac myxoma, or paradoxical embolism due to a patent foramen ovale allowing transit of venous thrombus into the arterial circulation. Acute embolic occlusion related to aortic aneurysmal disease and intramural thrombus is rare.
Other causes
Intense vasospasm, such as can be caused by ergotism34,35 or cocaine ingestion,36 have been reported to cause acute limb ischemia. Aortic dissection can result in occlusion of the distal aorta and iliac vessels when the true lumen is compressed by a pressurized false lumen. Iliofemoral deep vein thrombosis (DVT) with massive swelling of the thigh can compromise arterial inflow to the leg. The syndrome of phlegmasia cerulea dolens requires urgent catheter direct thrombolysis of the venous thrombus to restore venous outflow and thus arterial inflow to the limb.
Pathophysiology of Acute Limb Ischemia
Most emboli lodge in points of arterial branching: aortic, iliac, femoral, or popliteal bifurcations in the leg, and brachial bifurcation in the arm. In situ thrombosis most commonly affects the femoral or popliteal artery, particularly in the setting of an existing arterial bypass, ruptured atherosclerotic plaque, or low-output state. Sudden cessation of arterial flow to the extremity triggers a series of complex pathophysiological processes. Malperfused tissues shift from aerobic to anaerobic metabolism. The shift in lactate-to-pyruvate ratio further increases lactate production, increases the concentration of hydrogen ions, and induces acidosis. Progressive ischemia results in cell dysfunction and eventual cell death. Muscle hypoxia depletes intracellular adenosine triphosphate (ATP) stores, and the consequent dysfunction of the sodium/potassium-ATPase and calcium/sodium pumps causes leakage of intracellular calcium into myocytes.37 Intracellular free calcium levels rise and interact with actin, myosin, and proteases, leading to necrosis of muscle fibers. As the cellular membranes and microvascular integrity fail, intracellular potassium, phosphate, creatinine (Cr) kinase, and myoglobin leak into the systemic circulation. Reperfusion further amplifies these cellular changes.
Nerve and muscle tissue are quite susceptible to ischemic injury, so presence or absence of neuromotor deficit is of paramount importance in assessing the severity of acute limb ischemia. Irreversible muscle damage begins after 3 hours of ischemia and is nearly complete after 6 hours.38 In addition to myocyte injury, progressive microvascular damage follows skeletal muscle injury. The more severe the cellular damage, the greater the microvascular changes. In the setting of muscle necrosis, microvascular flow stops within a few hours. Traditionally, a window of 6 hours has been assumed before irreversible functional injury occurs. This time window may be longer in a “preconditioned” limb with collateral pathways.
Ischemic insult sets the stage for reperfusion injury, a process triggered by restoration of perfusion and mediated by a complex cascade of cytokines, reactive oxygen species (ROS), and neutrophils. Reactive oxygen species (e.g., superoxide anion, hydrogen peroxide, hydroxyl radicals, peroxynitrite) are produced by activated neutrophils and xanthine oxidase, an enzyme located on microvascular endothelial cells (ECs) of skeletal muscle and activated during ischemic conditions.39 Under normal conditions, xanthine dehydrogenase uses nicotinamide adenine dinucleotide to oxidize hypoxanthine to xanthine. Xanthine dehydrogenase is converted to xanthine oxidase after 2 hours of ischemia.40 During ischemia, ATP is degraded to hypoxanthine, but xanthine oxidase requires oxygen to convert hypoxanthine to xanthine. Thus, hypoxanthine accumulates during ischemia. When oxygen is reintroduced during reperfusion, xanthine dehydrogenase isoform becomes active again. Conversion of massive amounts of hypoxanthine generates reactive oxygen species.41
The essential substrate for production of these radicals, molecular oxygen, is provided by reperfusion. Xanthine oxidase–derived oxidants mediate the increased vascular permeability in postischemic muscle. The importance of elemental oxygen and the role of oxygen radicals in reperfusion injury is underscored by studies showing that reperfusion initially with deoxygenated autologous blood prevents increase in permeability after ischemia. Changing the perfusate to oxygenated blood during reperfusion mimicked the microvascular injury response seen after normoxic reperfusion.42 Similarly, gradual reintroduction of oxygen early in reperfusion decreases postischemic injury.42 Additional supplementation with free radical scavengers and reduced oxygen delivery further reduces injury of postischemic necrosis.43
Activated neutrophils are the principal agents responsible for local and systemic damage caused by reperfusion. Leukocytes play an equally important role in reperfusion injury. Activated neutrophils accumulate in the reperfusing muscle and produce reactive oxygen metabolites, release cytotoxic enzymes, and occlude microcirculation pathways.44 Leukocyte depletion has been shown to reduce the ischemia-reperfusion injury. Reperfusion with oxygenated blood depleted of leukocytes by the use of filters completely prevents development of vascular permeability in canine skeletal muscle.45,46 Interestingly, inducing neutropenia before ischemia in rats restores transmembrane potential and contractile function in postischemic rat muscle.47,48
Skeletal muscle ischemia and reperfusion triggers a number of additional inflammatory cascades that include complement activation, increased expression of adhesion molecules, cytokine release, eicosanoid synthesis, free radical formation, cytoskeletal alterations, adenine nucleotide depletion, alterations in calcium and phospholipid metabolism, leukocyte activation, and endothelial dysfunction.40 Interleukin (IL)-1β and tumor necrosis factor (TNF)-α are detected soon after reperfusion and induce adhesion molecules on the surface of endothelial cells, increase capillary leak, and stimulate production of IL-6 and IL-8, which further increase endothelial permeability, destroy endothelial integrity, and activate leukocytes.49–53
The clinical impact of these cellular responses to reperfusion results in tissue swelling, a catastrophic event in the closed spaces of the forearm, thigh, calf, and buttock. Elevated compartment pressures within fascial boundaries cause a compartment syndrome: elevated compartment pressures that reduce the perfusion gradient and capillary blood flow below the metabolic requirement, resulting in further ischemia and necrosis. Release of myoglobin can result in renal injury. Increased endothelial permeability can lead to acute lung injury, a process attenuated in animal models by chemically induced neutropenia, suggesting that activation and transmigration of neutrophils and loss of endothelial integrity are critical in acute lung injury in reperfusion injury.54 Thus, noncardiogenic pulmonary edema can develop after reperfusion of lower limbs, a process that can be prevented by granulocyte depletion.54,55
Diagnosis of Acute Limb Ischemia
The classic symptoms and physical examination findings of an acutely ischemic limb are commonly known as the six Ps: pulselessness, pallor, pain, poikilothermia, paralysis, and paresthesia. Pain is the most common symptom and progresses with ischemia. Pallor is an early finding in an ischemic extremity and is caused by complete emptying and vasospasm of the arteries (Fig. 46-6). Subsequent stagnation of microvascular circulation will cause mottling of the skin, which initially blanches with pressure. As ischemia continues, paresthesia develops, and numbness replaces pain, often falsely reassuring both patient and physician. In the final stages of ischemic injury, paralysis sets in, and skin mottling is fixed and nonblanching. Loss of motor function and marble-like appearance of the skin herald irreversible ischemic injury.
More importantly, it is the physical examination that allows classification of the severity of ischemia, urgency of revascularization, and prognosis after revascularization56 (Table 46-1). These clinical classes are also useful for determining the best intervention strategies. In general, Rutherford class I represents a viable and nonthreatened limb, akin to patients with chronic and noncritical ischemia. Rutherford class II symptoms describe a directly threatened limb. A class IIa limb is characterized by intact sensory and motor examination despite absent arterial Doppler signals in the foot. This limb is marginally threatened. Class IIb includes patients with an immediately threatened limb, characterized by sensory loss, mild motor function impairment, and absent Doppler arterial signals. This limb can be salvaged if treated immediately. Irreversible limb ischemia falls into Rutherford class III, with permanent nerve damage, profound sensory loss and motor paralysis, and absent arterial and venous Doppler signals. Revascularization of such a limb is harmful; amputation is required.
Treatment of Acute Limb Ischemia
Prompt recognition of acute limb ischemia and rapid restoration of arterial perfusion are cornerstones of therapy. The decision whether revascularization or primary amputation should be undertaken depends largely on the viability of the affected limb. In patients with a salvageable limb, selection of the type of revascularization therapy is equally important. The two major factors affecting morbidity and mortality among patients with acute limb ischemia are the burden of medical comorbidities and the delay in recognition and treatment of the ischemic limb. Other factors associated with lower amputation-free survival are increased age, race, diabetes, and absence of prompt initiation of anticoagulation.57
Surgical intervention has been traditionally associated with high perioperative mortality rates. In a compilation of 3000 patients treated surgically for acute limb ischemia in 30 centers between 1963 and 1978, 30-day mortality rates were as high as 25%.58 Despite rapid advances in surgical and anesthesia techniques, Jivegard reported a 20% mortality rate a decade later.10 Even in the 1990s, 30-day mortality after surgical intervention among selected patients enrolled in the TOPAS, Surgery versus Thrombolysis for Ischemia of the Lower Extremity (STILE), and Rochester randomized trials ranged from 5% to 18%.9,59,60
The high burden of cardiopulmonary disease and high surgical mortality in the population affected by acute limb ischemia provided an impetus for development of less invasive endovascular strategies. Evidence from randomized trials suggests equipoise between endovascular and surgical therapies in selected patients, particularly those with class I and IIa symptoms. The cause of limb ischemia, location of the occlusion, Rutherford class, as well as patient characteristics play a crucial role in selection of appropriate revascularization strategy. The Rochester, STILE, and TOPAS trials form a framework for selection of patients for endovascular therapies.9,59,60 These trials demonstrated that patients with underlying PAD or graft thrombosis and Rutherford class I and IIa thrombolytic-based endovascular therapies do indeed have better outcomes. Patients with cardioembolic events usually present with Rutherford class IIb symptoms and are best treated with prompt surgical embolectomy.4
In modern practice, a rigid division between open surgical and endovascular treatment is artificial. Although many patients can be treated with an entirely endovascular approach, and others require traditional surgical embolectomy, large numbers of patients are treated with hybrid approaches. Indeed, routine use of perioperative angiography suggests a high rate of residual thrombus, necessitating additional combined surgical and endovascular intervention in up to 90% of complex cases.61
Initial Medical Management
Regardless of the revascularization strategy selected, the basic principles of initial therapy are the same: fluid resuscitation, analgesia, and administration of antithrombin and antiplatelet therapy. After decades of clinical experience, heparin therapy has been shown to decrease ischemic injury, reduce thrombus propagation, and improve survival.4,62–64 Some studies dispute the benefit of perioperative anticoagulation, even in patients with a cardiac source of emboli, but the overwhelming amount of data support perioperative anticoagulation with heparin.65 Unfractionated heparin (UFH) should be administered at high doses (100-150 units/kg), with a goal of rapidly achieving a therapeutic level of anticoagulation and a rise in partial thromboplastin time (PTT) by a factor of 2 to 2.5 above baseline. Patients with heparin-induced thrombocytopenia (HIT) should be treated with intravenous (IV) direct thrombin inhibitors (DTI) such as lepirudin or argatroban. Bivalirudin, another DTI commonly used in coronary and endovascular interventions, has a relatively short half-life and is more familiar to most vascular specialists. The decision regarding long-term anticoagulation must be made based on the etiology of the ischemic event, outcome of revascularization, and the balance between bleeding and thrombotic risk.
Correction of laboratory abnormalities and stabilization of the underlying acute medical condition are imperative to achieve best clinical outcomes. Certain laboratory characteristics predict ultimate therapeutic success. Patients presenting with elevated Cr kinase and neutrophil count have a 50% risk of amputation as compared to a 5% risk among those with normal enzyme and neutrophil levels.66 This finding underscores the poor clinical outcomes in patients with advanced ischemic injury of skeletal muscle. In patients who present with irreversible tissue loss, alkalinization of urine may be required to prevent renal injury from myoglobinuria. In some cases, the cause of acute limb ischemia is itself immediately life threatening, such as myocardial infarction (MI) complicated by LV thrombus and cardiogenic shock, or aortic dissection or infective endocarditis with hemodynamic compromise due to valvular incompetence. In such cases, the principle of “life over limb” should guide best therapeutic strategy.
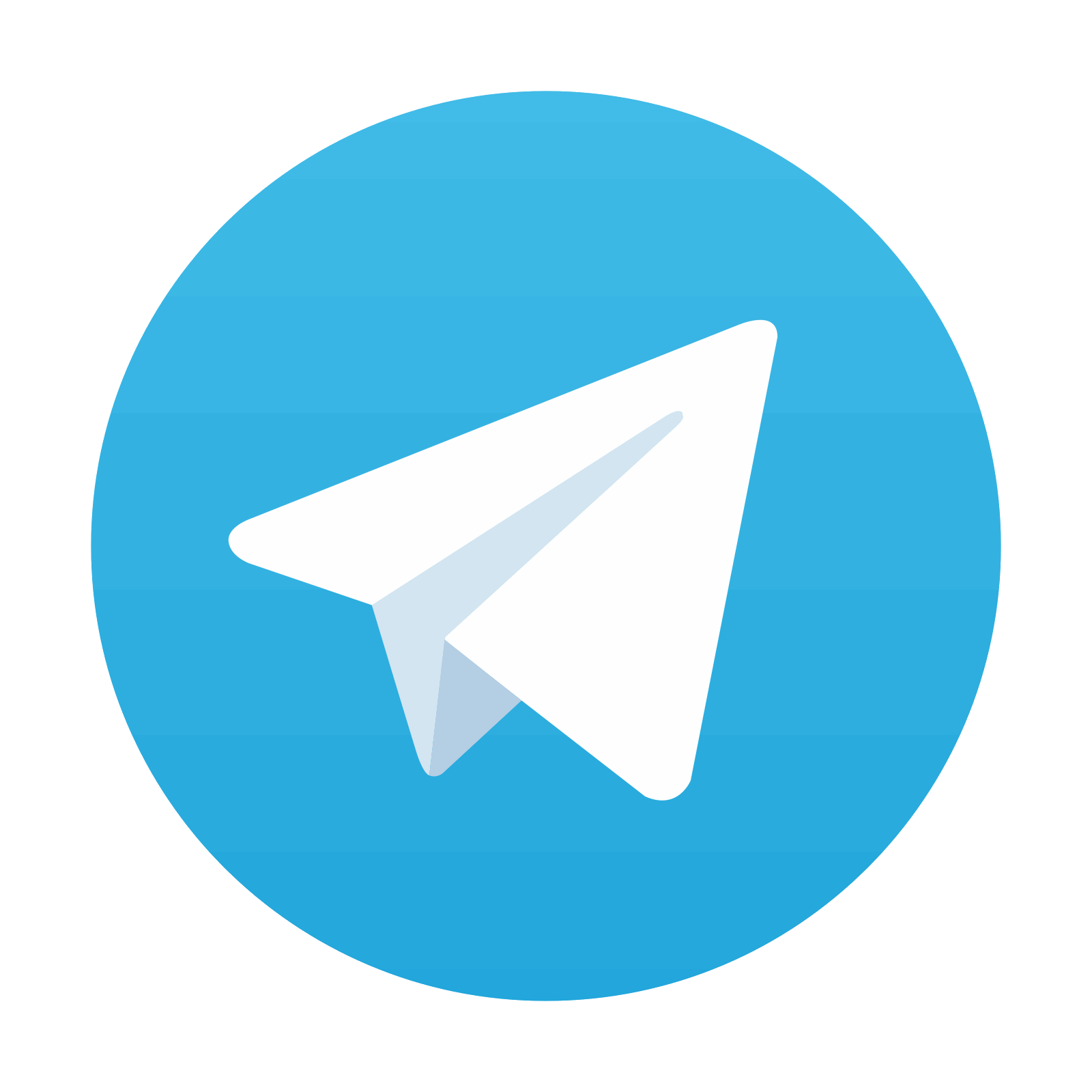
Stay updated, free articles. Join our Telegram channel

Full access? Get Clinical Tree
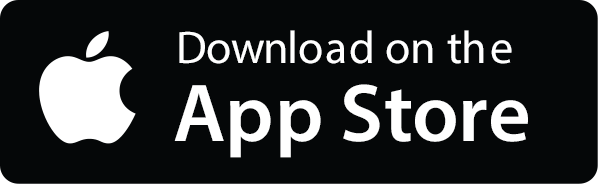
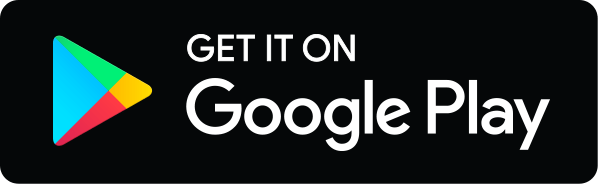