Two features characterize the propagation of the action potential: (1) how fast it propagates, i.e., conduction velocity; and (2) how fast it recovers and accepts new impulses, i.e., refractory period. Some tissues, like the slow AV nodal pathway, have a slow conduction yet a fast refractory period (in comparison to the fast AV nodal pathway). Other tissues, like the accessory pathway, have a fast conduction but a slow refractory period (in comparison to the AV node). An arrhythmia may result from the following mechanisms: EAD-triggered activity may be due to a congenital or acquired long QT syndrome, electrolyte disturbances, or antiarrhythmic drugs. Automaticity and DAD-triggered activity are generally due to ischemia, catecholamine excess, hypoxia, acid–base disorders, or calcium overload from digoxin toxicity. Automaticity may also be due to cavity stretch. A premature ventricular or atrial complex results from the same three mechanisms. In patients with heart disease, a change in sinus rate may create a ventricular reentry around the abnormal myocardial tissue, which could be isolated (PVC) or could become sustained into VT. PVC may also result from triggered activity arising from the abnormal tissue, which is a tissue prone to spontaneous depolarization, or fractionated late diastolic potential (a form of automaticity). Overall, the morphologies of the PVC and VT are frequently similar. Idiopathic PVCs arising from the RVOT are generally due to triggered activity. All arrhythmias can exhibit a warm-up phenomenon, wherein the rate quickly accelerates upon initiation, but this is most characteristic of automatic tachycardias (such as automatic atrial tachycardia). Induction of arrhythmias in the EP lab- Reentry can be initiated by pacing or by premature impulses. Reentry can be terminated by overdrive pacing or by premature impulses that enter the reentrant circuit and get blocked inside it, breaking it. Conversely, pacing and premature impulses are not effective in initiating or terminating automatic tachycardias, which are thus difficult to induce in the EP lab. In fact, in the EP lab, automatic tachycardia is defined as a tachycardia that cannot be initiated or terminated with programmed electrical stimulation. Also, as opposed to reentry, automatic tachycardias do not respond well to DC cardioversion, as the automatic focus keeps firing independently of the surrounding electrical stimuli. Figure 16.1 Action potential of atrial and ventricular myocardium. Phase 0 corresponds to myocardial depolarization and QRS on ECG, phases 1 and 2 (plateau) correspond to the ST segment, phase 3 corresponds to the T wave, and phase 4 corresponds to the TP segment. Phases 1–3 correspond to repolarization, during which the cells are refractory to a new depolarization (refractory period). All myocardial cells reach the same phase 2 plateau level around the same time, and thus the ST segment is normally a flat isoelectric segment without intramyocardial gradient. Conversely, the T wave is like the bell curve of the repolarization of all myocardial cells, some of which repolarize early, and some late. The width of T and the length of QT indicate, in a way, how heterogeneous and dispersed the repolarization is, and thus the risk of arrhythmia. The normal U wave is a diastolic wave related to the mechanical stretch of the myocardium in phase 4 (diastole). The abnormal U wave could be part of phase 4 (delayed afterdepolarization, DAD), or part of phase 3 in patients with prolonged repolarization (early afterdepolarization [EAD] seen in patients with prolonged QT). In the latter patients, the U wave is actually a T2 repolarization wave; their QT is a QTU interval, and the distinction between T and U may not be very relevant in these patients. The depolarization of one cell causes the sodium channels of adjacent cells to open and depolarize. There are two properties of electrical propagation: (1) conduction velocity, which depends on phase 0; (2) refractory period, which depends on the duration of phases 2–3. A reduction in the steepness of phase 0 (dashed phase 0, class I AAD) leads to slower velocity and wide QRS, while a prolonged phase 3 (dashed phase 3, class III AAD) leads to a prolonged QT interval. Phase 1 uses IKto (transient outward K channel), phases 2 and 3 use outward IKr and IKs, and phase 4 depends on inward IK1 and on Na/K pump, which pumps 3 Na out and 2 K in, creating the negative baseline intracellular potential (the cell potential is close to the equilibrium potential of K). The action potential duration is increased at lower heart rates, and is reduced with tachycardia or β-adrenergic stimulation. It is mainly the duration of phase 3 that is affected with changes in heart rate. ↓ indicates the entry of the ion inside the cell; ↑ indicates the exit of the ion. Figure 16.2 Action potential of the SA and AV nodes is characterized by a spontaneous depolarization in phase 4. In addition, phase 0 is characterized by calcium rather than sodium entry, explaining the effect of calcium channel blockers on slowing the SA and AV node. Phase 0 is also slower than phase 0 of the atrial and ventricular cells, thus explaining the slower conduction through the AV node. Phase 4 depends on the funny K and Na channel (If). Spontaneous phase 4 depolarization may be seen in ischemic atrial or ventricular cells (increased automaticity). Triggered activity can be spontaneous like automaticity, resulting from leakage of positive ions into the myocardial cell, but can also be induced with a premature beat or with a pause, particularly a pause introduced after fast pacing. Similarly to reentry, it may get entrained with pacing or terminated. Thus, during EP study, triggered activity resembles reentry. Since it depends on calcium channels, triggered activity may respond to calcium channel blockers (Table 16.1). Figure 16.3 Illustration of reentry as a mechanism of tachyarrhythmia. In order to have reentry, adjacent myocardial areas (A and B) need to have different refractory periods and different conduction velocities, separated by an anatomical or functional barrier. The pathway with the shorter refractory period needs to have a slower conduction. The larger the difference in refractory period between A and B, the easier it is to initiate an arrhythmia; this difference between refractory periods is called the tachycardia zone. The slower the conduction velocity in A, the more likely it is to sustain the arrhythmia (slow conduction leads to a large excitable gap, Figure 16.4). When the rhythm is regular, the electrical activity spreads through both areas simultaneously (top). After a premature beat, region B is still in its long refractory period, but region A has recovered (lower left). The electrical activity spreads through region A, then meets the tail end of region B; at that time, if region B has not recovered from its refractory period, arrhythmia does not occur (i). Since A conducts slowly, region B may well recover from its refractory period, allowing conduction to spread retrogradely through region B (ii). Eventually, this retrograde impulse reaches A faster than the electrical activity originating from the sinus node reaches A. Since A has a short refractory period, the impulse may propagate through A and stimulate the whole cardiac chamber again. Thus, this circle keeps repeating itself, stimulating the myocardial tissue at a rate faster than normal: this is the reentrant tachyarrhythmia. These areas of slow conduction and different refractory period are seen around a scar (prior infarct, fibrosis, cardiomyopathy), an ischemic area, or a functionally slow area (crista terminalis for atrial flutter, slow pathway of AVNRT) or fast area (accessory pathway of AVRT). Scarred myocardium is an anatomic barrier for reentry. The peri-scar myocardium has a slow conduction, but shorter action potential and shorter repolarization than the surrounding tissue. An area is functionally slow when fibers in that area are organized transversally rather than longitudinally; electrical activity spreads more slowly transversally than longitudinally through gap junctions.
16
Action Potential Features and Propagation: Mechanisms of Arrhythmias, Antiarrhythmic Drugs
I. Action potential (see Figures 16.1, 16.2)
II. Action potential propagation and mechanisms of arrhythmias
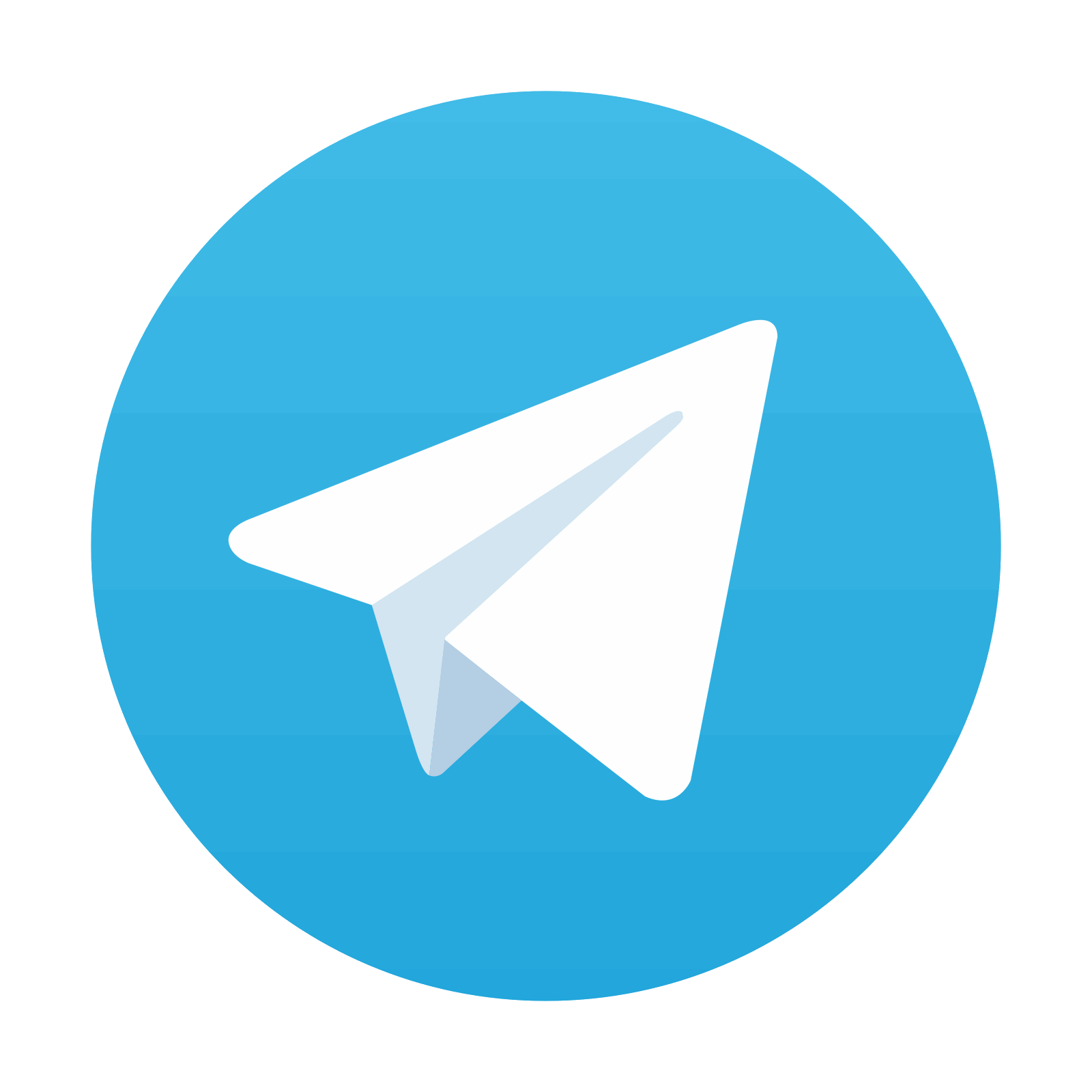
Stay updated, free articles. Join our Telegram channel

Full access? Get Clinical Tree
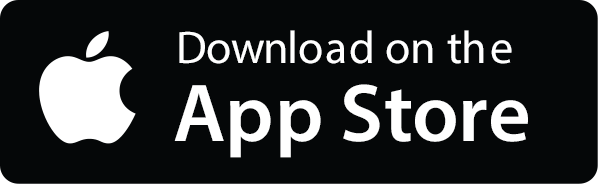
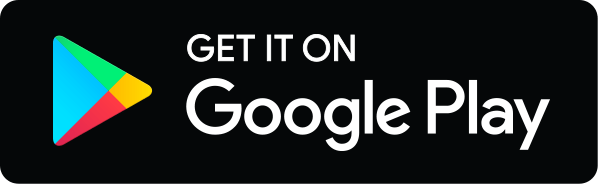