Chapter 61 Acquired Heart Disease
Valvular
Historical Perspective
Heart failure from mitral stenosis was well recognized by the late 19th century, and efforts at surgical correction began well before the heart-lung machine was available.1 In 1897, Samways suggested (but never acted on) the possibility of dilating the stenotic mitral valve. Based on his postmortem studies of rheumatic heart disease in London, Brunton in 1902 proposed surgical intervention for mitral stenosis by passing a dilator through the wall of the left ventricle retrograde into the mitral valve orifice. His proposal was shunned by London physicians, and Brunton never tried this maneuver. The concept, however, was applied 20 years later in Boston when the first report of successful surgical correction of mitral stenosis appeared in 1923. Cutler and Levine reported successful relief of mitral stenosis by incision of the valve with a knife introduced through an apical left ventriculotomy. In 1925, Soutter performed the first successful closed mitral commissurotomy at the London Hospital by introducing his index finger through the left atrial appendage. Despite Soutter’s success, he received no more patient referrals, and another 20 years elapsed before the procedure became widespread. In June 1948, Bailey in Philadelphia and Harken in Boston each performed a successful closed mitral commissurotomy. Thereafter, it became widely used to treat mitral stenosis.
Surgical attempts to correct aortic stenosis also began in the early 20th century.1 In 1912, Tuffier, in Paris, unsuccessfully attempted transaortic digital dilation of a stenotic aortic valve. In Charleston, South Carolina, in 1948, Smithy performed the first successful aortic valvotomy in a 21-year-old woman from Ohio. Smithy died later that same year of aortic stenosis at the age of 34 years. Three years later, in Philadelphia, Bailey reported successful aortic valvotomy by insertion of a mechanical dilator across the stenotic valve of patients to open fused commissures. In 1952, Hufnagel and Harvey at Georgetown University placed the first prosthetic ball valve into the descending aorta of a patient with aortic insufficiency. Surgery on the aortic valve under direct vision required the development of cardiopulmonary bypass by Gibbon in 1954. In 1955, Swann performed the first successful aortic valvotomy using hypothermia and inflow occlusion. Initially, open aortic valve operations were limited to aortic valve commissurotomy and débridement of calcified aortic valve leaflets. Harken, in Boston, in 1960, and Starr, in Portland, Oregon, in 1963, however, reported replacement of the aortic valve with a prosthesis. In 1962, Ross in London successfully performed orthotopic homograft valve replacement. In 1967, Ross performed the first pulmonary autograft procedure (Ross procedure) for correction of aortic stenosis. In the mid-1960s, stent-mounted porcine aortic valves were implanted, but these formaldehyde-fixed valves degenerated rapidly. In 1974, Carpentier, in Paris, reported superior longevity of the glutaraldehyde-preserved porcine valve; thereafter, their usage was well established. By 1981, bileaflet mechanical valves were widely implanted in the aortic and mitral positions and largely supplanted the use of ball cage mechanical valves. In the mid-1990s, bovine pericardial valves were shown to have durability similar to porcine valves and both types of bioprostheses became widely implanted. By 2004, most valves implanted in the United States were tissue valves. In 2002, transcatheter aortic valve replacement was performed by Cribier in Rouen, France.
Diagnostic Considerations
Although most valve lesions may be accurately diagnosed by echocardiography, cardiac catheterization may be necessary to confirm the diagnosis or to provide additional information pertaining to ventricular function. Before surgery, it may be appropriate to exclude the presence of coronary artery disease. Mitral or aortic valve areas may be determined at cardiac catheterization using the Gorlin formula,2 which permits calculation of the valve area, as follows:
where C is an empirical constant, 44.5 for the aortic valve and 38 for the mitral valve.
Mitral Valve
Surgical Anatomy of the Mitral Valve
The leaflets are suspended from the mitral annulus, a collagenous structure that encircles the orifice between the left atrium and ventricle. Although the two leaflets have approximately the same surface area, they have different shapes (Fig. 61-1). The anterior leaflet is rectangular. Its base is attached to the mitral annulus anteriorly and the width of the base is approximately one third the circumference of the mitral annulus. This attachment of the anterior leaflet to the mitral annulus extends to the aortic annulus through fibrous tissue, providing fibrous continuity between the aortic and mitral valves; the left ventricular side of the anterior leaflet of the mitral valve is visible immediately as the surgeon looks down through the aortic valve into the left ventricle. The posterior leaflet is rectangular and its attachment to the mitral annulus extends for approximately two thirds of the circumference of the mitral annulus. The two leaflets are separated by two distinct commissures.
There are three important surgical landmarks (see Fig. 61-1). First, the circumflex coronary artery runs along the epicardial surface of the heart overlying the posterior mitral annulus. Only millimeters of left atrial muscle separate the artery from the annulus, making it susceptible to injury during mitral valve surgery. Second, the aortic valve is in close approximation to the anterior leaflet of the mitral valve (aortomitral continuity). The noncoronary leaflet of the aortic valve is therefore susceptible to injury during mitral surgery. Third, the atrioventricular node is located deep to the posteromedial commissure of the mitral valve.
Mitral Stenosis
Diagnosis
Diagnostic Tests
Natural History
Currently, the natural history of mitral stenosis is impossible to know precisely because of successful surgical intervention. Data collected from the era before widespread surgery for mitral stenosis, however, have indicated that after diagnosis, the mean survival of patients with asymptomatic mitral stenosis was 15 to 20 years; on the other hand, patients with symptoms had a mean survival of only 2 to 7 years.3 Left atrial distention predisposes to atrial fibrillation and its associated intra-atrial thrombus formation. Up to 20% of patients with mitral stenosis and atrial fibrillation may sustain systemic embolization, especially strokes.
Treatment
Balloon Mitral Valvuloplasty
First performed in 1984, balloon mitral valvuloplasty has become the treatment of choice for select patients with mitral stenosis.4 Echocardiography may be used to determine patients considered to be good candidates, including those with pliable valve leaflets but without significant valvular calcification or deformation of the chordae tendineae. Contraindications to this procedure include the presence of moderate mitral regurgitation, thickening and calcification of the mitral leaflets, and scarring and calcification of the subvalvular apparatus.5 Performed in the cardiac catheterization suite under fluoroscopic guidance, the technique entails advancement of one or two balloon catheters across the interatrial septum and inflation of the balloon within the stenotic mitral valve.
Balloon mitral valvuloplasty has provided good short-term and intermediate-term results in appropriately selected patients. Balloon inflation should increase the mitral valve area to approximately 2 cm2. This increase in mitral valve area is usually associated with a significant decline in left atrial pressure and transvalvular gradient and with at least a 20% increase in cardiac output. The mortality rate associated with balloon mitral valvuloplasty is 0.5% to 2%. Other risks associated with this procedure include systemic embolism, cardiac perforation, and creation of mitral regurgitation; the risk of each of these complications is approximately 1% to 2%. Increased pulmonary vascular resistance has been shown to decline after successful balloon valvuloplasty. Approximately 10% of patients are left with a residual interatrial septal defect. Three years after balloon valvuloplasty, at least 66% of patients are free of subsequent intervention. In appropriately selected patients, the results of balloon valvuloplasty compare favorably with those of surgical valvuloplasty.6
Open Mitral Commissurotomy
The mortality rate associated with open mitral valvuloplasty is less than 2%.6 When performed in appropriately selected patients, the freedom from subsequent mitral valve intervention is approximately 75% at 5 years. Nonetheless, because of less procedure-related morbidity, balloon valvuloplasty is the procedure of choice.
Mitral Valve Replacement
The contribution of the mitral apparatus to overall left ventricular function is now well appreciated.7 A mechanical advantage is afforded the left ventricle by the connection of its apex (via the papillary muscles) to the mitral annulus through the chordae tendineae; elimination of this connection by removal of the entire mitral apparatus leads to loss of left ventricular function. Convincing data have demonstrated that preservation of at least some of the chordae tendineae at the time of mitral valve replacement results in better long-term left ventricular function than mitral valve replacement with chordal separation. Therefore, if mitral valve replacement is required, efforts should be made to preserve the posterior and, in some cases, anterior leaflets of the native mitral valve.
The operative mortality rate associated with mitral valve replacement for mitral stenosis is 2% to 10%.8 Operative mortality is increased with advanced age and the presence of coronary disease. Pulmonary hypertension typically resolves after valve replacement, but several weeks or months may be required. The 5-year survival rate after replacement is 70% to 90%.6,9
Mitral Regurgitation
Pathophysiology
The compensatory mechanism whereby the left ventricle adapts to maintain an adequate systemic blood flow (forward cardiac output) is volume overload; it must pump the combined volume of systemic and regurgitant flows (Fig. 61-2). Volume overload leads to cardiac dilation as well as left ventricular hypertrophy. Because the left ventricle ejects into the reduced resistance of the left atrium, parameters of systolic function (ejection fraction) are increased in mitral regurgitation. As with aortic insufficiency, however, the left ventricle ultimately fails with chronic volume overload. Normal parameters of systolic function indicate significant contractile dysfunction of the left ventricle. An ejection fraction less than 40% in the setting of mitral regurgitation indicates significant left ventricular contractile dysfunction.
< div class='tao-gold-member'>
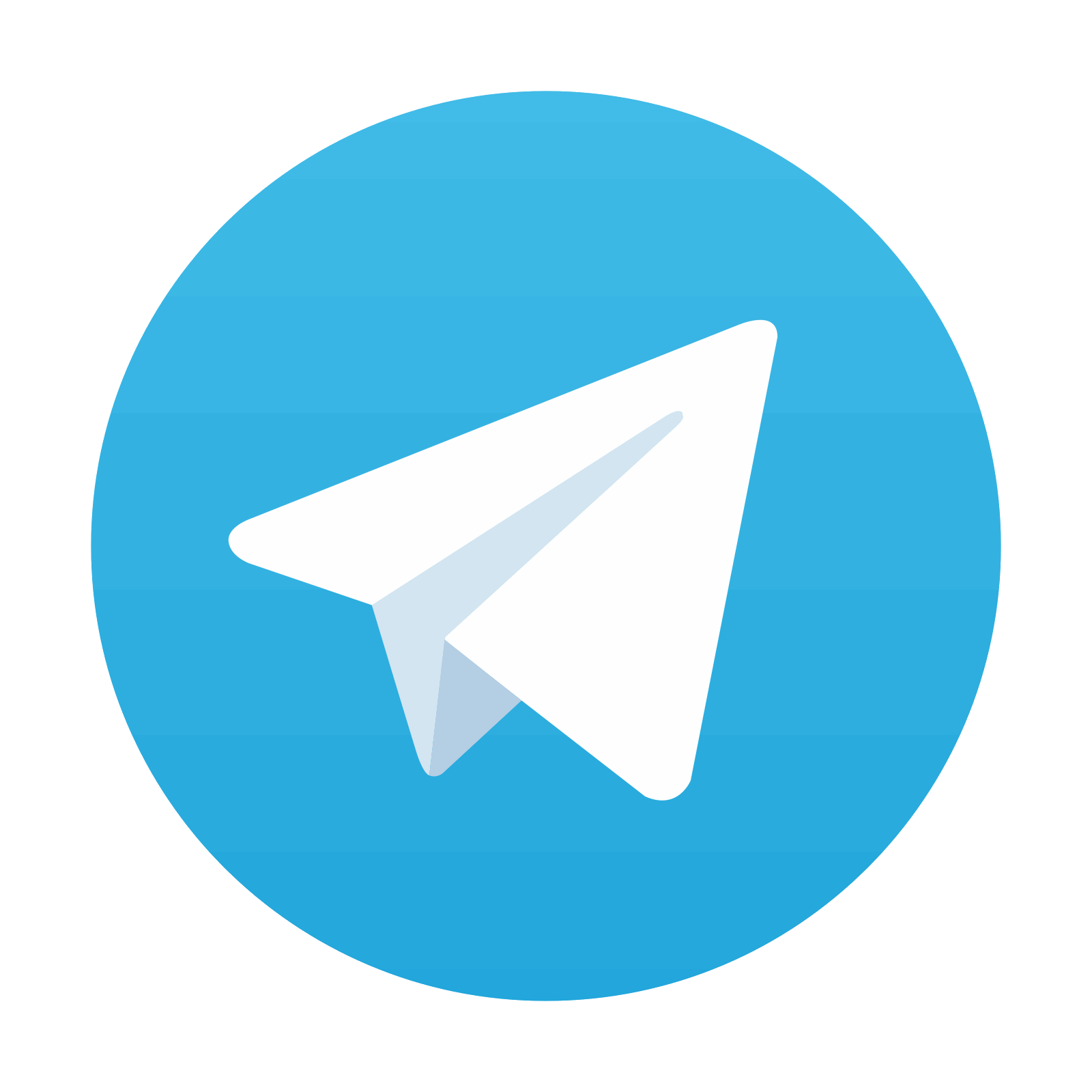
Stay updated, free articles. Join our Telegram channel

Full access? Get Clinical Tree
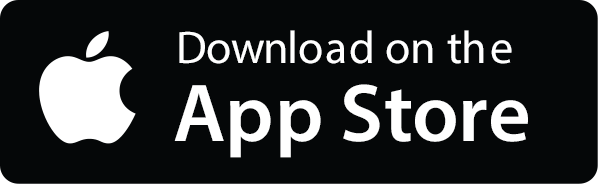
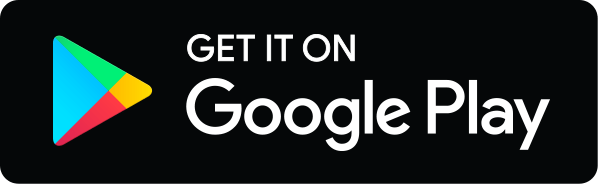