Clinicians use branch pulmonary artery (BPA) blood flow distribution to help determine the need for intervention. Although phase-contrast magnetic resonance (PCMR) flow measurements are accurate, this has never been shown in the vicinity of a BPA ferromagnetic stent (FS) which produces significant susceptibility artifact. We retrospectively reviewed 49 consecutive PCMR studies performed from 2005 to 2012 on patients with repaired conotruncal anomalies and either left (n = 29) or right PA (n = 20) stents. Three methods of measuring the stented BPA flow were compared: (1) main PA (MPA) minus nonstented BPA, (2) direct PCMR of stented BPA away from the artifact, and (3) pulmonary venous flows (ipsilateral to stented BPA and derived pulmonary blood flow ratio from bilateral pulmonary venous flows). Internal consistency was tested with the Student t test, linear regression, Bland–Altman analysis, and intraclass correlation (ICC). The mean age was 11.7 ± 6.9 years with 5.8 ± 4.2 years between stent placement and CMR. There was good agreement without significant difference between MPA-derived stented BPA flow (method 1) and direct PCMR of stented BPA (method 2; 41 ± 19% vs 39 ± 19%, p = 0.59; R 2 = 0.84, p <0.001; ICC = 0.96). There was also good agreement between methods 1 and 2 compared to pulmonary venous flows, with the highest correlation occurring between method 2 and ipsilateral pulmonary venous flow (R 2 = 0.90, p <0.001; ICC = 0.97 for MPA-derived–stented BPA flow; R 2 = 0.94, p <0.001; ICC = 0.98 for direct PCMR of stented BPA). Eleven of the 49 patients (22%) underwent interventional catheterization after PCMR. In conclusion, in the vicinity of a BPA FS, accurate measurement of the net fractional pulmonary blood flow ratio is feasible. PCMR adjacent to the stent and ipsilateral pulmonary venous flows provide the most internally consistent data. These data underscore PCMR’s utility in managing patients with implanted FS.
After repair of Tetralogy of Fallot (ToF) and other related conotruncal anomalies, a substantial portion of patients will have residual branch pulmonary arterial (BPA) stenosis which will benefit from BPA stent implantation with the aim of distributing balanced flows to both lungs and optimizing cardiopulmonary efficiency. Over time, these stents are subject to stenosis secondary to in-stent endothelial proliferation or to the growth of the patient relative to the caliber of the stent at the time of implantation. Consequently, there is a need for continued surveillance to determine the timing of future balloon dilatations of the implanted stent. Many of the implanted BPA stents are composed of ferromagnetic materials, which produce localized artifact in the region of the stent during cardiac magnetic resonance (CMR) examinations. These stents are generally considered magnetic resonance (MR) safe, but signal distortion may preclude direct anatomic and phase CMR (PCMR) measurements within the stent. Radionuclide pulmonary scintigraphy has traditionally been used for measuring the net pulmonary blood flow (PBF) distribution in patients with BPA stenosis. However, in more recent years, clinicians have turned to PCMR which has been shown to accurately measure PBF without the use of ionizing radiation, of particular importance to pediatric age patients. The accuracy of PCMR in the context of an implanted ferromagnetic BPA stent is unknown. We hypothesized that measurement of the stented BPA flow could be accomplished in the presence of ferromagnetic stents (FSs).
Methods
We retrospectively reviewed 49 consecutive repaired conotruncal anomalies PCMR and cine MR studies performed from January 2005 to December 2012. All patients underwent either left (n = 29) pulmonary artery (LPA) or right (n = 20) PA (RPA) stainless steel stent placement. Patients with bilateral BPA stents and patients with functional single ventricle were excluded from the study. The investigators had full access to the data and take responsibility for its integrity. All investigators have read and agree to the manuscript as written. The study was approved by the institutional review board.
Our CMR protocol includes an initial steady-state free precession bright blood 3-dimensional static volume set acquired in the axial plane during diastole. Multiplanar reformatting was used for prescribing the optimal imaging plane to acquire segmented PCMR and cine data. Retrospective electrocardiogram-gated through-plane PCMR images were acquired for the main PA (MPA; or conduit in patients with pulmonary atresia) and nonstented BPA in all patients. Where technically feasible, PCMR was acquired immediately proximal or distal to the stent. PCMR measurements were acquired proximal to the stent only if there was adequate length between the MPA and the proximal edge of the stent to allow for a thin slice plane (3.0 to 5.0 mm) as suggested on cine imaging. PCMR measurements distal to the stent were only acquired if there was adequate length between the distal edge of the stent and the upper lobe BPA so that all lung segments supplied by the stented artery could be reliably acquired in a single acquired slice plane. In the rare case of where a single discrete upper lobe BPA had bifurcated, that too was included in the PCMR flow measurement. Our protocol expanded over time to include pulmonary venous flow measurements as an additional means of assessing the PBF distribution ( Figure 1 ). When possible (based on whether all the pulmonary veins on one side formed a common vein of sufficient length), all the pulmonary veins on one side were measured in one acquisition. Otherwise, they were acquired as separate upper and lower lobe pulmonary venous acquisitions. The typical parameters we use for PCMR have been described. In younger children (with an RR interval of approximately 600 ms), the parameters include 220 × 165 mm field of view, 192 × 144 matrix, 3- to 4-mm slice thickness, echo time of 2.8 ms, bandwidth of 501 Hz/px, repetition time of 35 ms, 25° flip angle, 15 measured phases, 30 calculated phases, 3 segments, and 3 averages. Typical encoding velocities were 150 cm/s for the MPA, RPA, and LPA and 80 cm/s for the pulmonary veins.
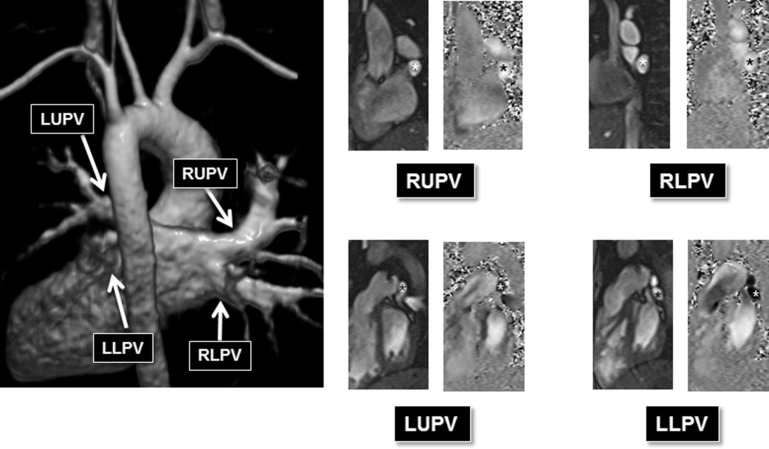
An entire retrospective electrocardiogram-gated steady-state free precession cine volume set from base to apex was acquired for the assessment of ventricular volumes and ejection. Typical parameters included slice thickness of 5 to 8 mm (depending on the size of the patient), temporal resolution 25 to 35 ms, echo time 1.2 ms, matrix 192 × 100%. Four cardiovascular magnetic resonance imaging (MRI) specialists (in alphabetical order MAF, MAH, MSK, and KKW) with 20, 10, 25, and 6 years, respectively, of cardiac MRI experience assisted in the acquisition and personally analyzed and interpreted the PCMR and cine volumetric data.
Three different methods for measuring flow through the stented artery were compared:
Method 1 was an indirect measure of the fractional net flow (Q) to the stented BPA by taking the difference between the MPA and the nonstented BPA:
Method 2 calculated the stented Q BPA by direct measurement acquired either proximal or distal to the stent:
Method 3 used measured pulmonary venous flows as a surrogate for the Q stented BPA .
In method 3a, ipsilateral pulmonary venous flows from the stented lung were used as a surrogate for the Q stented BPA .
Method 3a:
In method 3b, the PBF ratio was derived by comparing pulmonary venous flows from both lungs.
Method 3b:
The 3 methods for calculating the fractional net pulmonary flow were tested for internal consistency using 2-tailed paired t test, linear regression, Bland–Altman analysis, and intraclass correlation (ICC). The forward flow measured at the MPA with PCMR was compared to the cine derived right ventricular stroke volume using linear regression. A p-value of <0.05 was considered significant.
Results
The time between stent implantation and CMR study was 5.8 ± 4.2 years (range, 1 day to 14.4 years). Conotruncal anomalies included ToF, truncus arteriosus, transposition of the great arteries, and double outlet right ventricle ( Table 1 ). Additional diagnoses included pulmonary atresia with intact ventricular septum (n = 1), posterior malalignment ventricular septal defect with aortic stenosis and aortic arch hypoplasia (n = 1), and posterior malalignment ventricular septal defect with interrupted aortic arch (n = 1). Two patients with ToF and pulmonary atresia had undergone repair which included unifocalization and did not have any significant residual aortopulmonary collaterals ( Table 1 ).
Variable | Total (n=49) |
---|---|
Age (years) | 12±7 |
Diagnoses | |
Tetralogy of Fallot | 33 |
Tetralogy of Fallot/pulmonary stenosis | 22 of 33 |
Tetralogy of Fallot/pulmonary atresia | 11 of 33 |
Tetralogy of Fallot/pulmonary atresia with multiple aorto-pulmonary collaterals | 2 of 11 |
Transposition of the great arteries | 5 |
Double outlet right ventricle | 1 |
Truncus arteriosus | 7 |
Other | 3 |
Left pulmonary artery stents | 29 |
Right pulmonary artery stents | 20 |
Number of studies with pulmonary vein data | 21 |
Catheterization and surgical records were reviewed to obtain product information on the composition of the stents implanted into patients in the study cohort. Stent model information was available only for those patients whose stents were implanted at the Children’s Hospital of Philadelphia. Of the 49 study patients, 29 (59%) had specific device information. There were 26 Palmaz Genesis, 2 IntraStent LD, and 1 Formula 418 stents cataloged. All stents were made of stainless steel.
All patients had PCMR data acquisition at the MPA and the nonstented BPA. There were 29 patients who had PCMR data acquisition adjacent to the stent ( Figures 2 and 3 ). Two of these patients (both with RPA stents) had PCMR acquired proximal to the stent, and 27 patients had PCMR applied distal to the stent. There were 21 patients with pulmonary venous flow data acquired on the same side as the BPA stent. Nineteen of these patients had pulmonary venous flows acquired for both lungs. Based on all methods, 9 of the 49 patients had ≤33% flow to the stented BPA consistently across all applied methods.
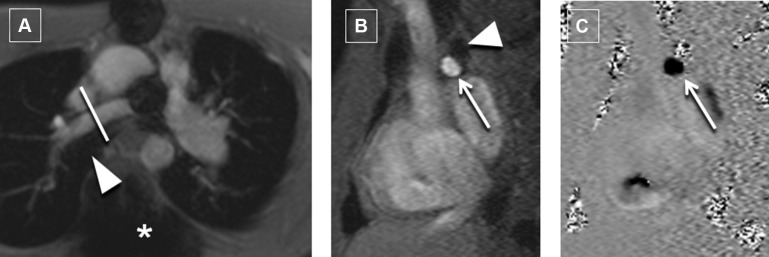
