8 Cardiac Catheterization
8.1 Basics
Today, only very few purely diagnostic cardiac catheterizations are performed, as the anatomical situation for most heart defects can be visualized very precisely using echocardiography or magnetic resonance tomography, which is used especially in larger patients and adults.
Nevertheless, diagnostic cardiac catheterizations are occasionally needed for specific clinical questions such as for exact blood pressure measurements and the calculation of blood flow resistance, to quantify shunts, or in those cases where findings from noninvasive diagnostics were unclear or contradictory. Pressure values, oxygen content in the individual cardiac chambers or segments of blood vessels, and additional indicator methods, if needed, are used to assess the hemodynamic situation (e.g., calculating cardiac output or identifying shunts).
Another indication not to be underestimated is the surgeon’s desire and need to obtain additional morphological information preoperatively so that the surgery can proceed more quickly and more safely. Furthermore, there are a number of patients in whom an accurate diagnosis is possible only by cardiac catheterization; for example, if the diagnostic window is insufficient for echocardiography and MRI cannot be performed due to a pacemaker.
The special features of diagnostic cardiac catheterizations for some typical cardiac defects are discussed below.
8.2 Vascular Access Routes
Normally, most cardiac catheterization examinations in children as well as adults are performed via vascular access routes in the groin (femoral artery and vein; Fig. 8.1). The vessel is punctured using the Seldinger technique and the sheath is inserted over a guidewire. These sheaths consist of a hemostatic valve (rubber plug) through which the actual catheters can be inserted, and a side port through which the sheath can be flushed with saline solution, for example, and blood samples can be drawn. Standard sheaths are available in various sizes with an inner lumen from 4 F to 16 F (1 F = ⅓ mm).

Other access routes may become necessary because of vascular occlusions (Fig. 8.1). Commonly used access routes in this event are the brachial or axillary vessels, the jugular vein, the carotid artery, and the subclavian vein. Access via the umbilical vessels is also possible in neonates—for a Rashkind procedure or treatment of a critical aortic stenosis, for example.
The transhepatic access is very rarely used, but is a safe access should the peripheral venous accesses be completely occluded. A percutaneous puncture of the hepatic vein is made under sonographic or fluoroscopic guidance and the sheath then advanced into the right atrium.
8.3 The Catheter Examination
The cardiac catheter examination procedure should be standardized to allow a precise hemodynamic examination with valid results. To ensure this, the following steps are helpful.
Documentation of the baseline condition
This includes documentation and monitoring of the vital signs, including pulse, ECG, transcutaneous O2 saturation, and noninvasive blood pressure.
Initial adequate stable hemodynamic situation
The patient should be calm and at rest and have no significant episodes of excitation. In most modern centers, the examination is performed even in small children under deep conscious sedation and with spontaneous breathing. General anesthesia is not routinely needed. A blood gas analysis should be performed to document adequate ventilation.
Measuring blood pressure and shunt fraction
These steps should best be taken initially, before the contrast medium is administered, as the contrast medium can lead to volume load that may result in false high blood pressure readings (e.g., high end-diastolic pressures). The pressure curves are recorded at defined positions of the heart. In addition, pressure curves are recorded along the course of blood vessels or anatomical structures (pull-back pressure curves). The first blood gas analysis should be made in room air or at FiO2 of less than 0.3. Depending on the results of these baseline recordings, a subsequent analysis can be made later with increased oxygen supply, for example, to test the responsiveness of the pulmonary vascular system.
Angiographies
After the hemodynamic situation has been determined, the vascular segments or cardiac chambers to be examined are visualized by the use of angiography. A sufficient amount of contrast medium is injected via the cardiac catheter using a high-pressure injection pump and the images are recorded continuously on film or in digital form.
Interventions
If an intervention is performed, the major and important steps of the procedure should also be documented (e.g., balloon inflation for valve stenosis or stent placement in a stenotic segment of a vessel). In addition, the result of the intervention and any complications that may have occurred (e.g., development of a new valvular insufficiency or contrast media extravasation) must be documented.
Final documentation
After the catheterization is completed, the patient’s condition must be assessed and documented before he or she is transferred to the ward for further monitoring and care. In addition to vital signs, this also includes checking the vascular status and the perfusion of the limb where the puncture was made.
8.4 Various Catheters
An important part of the careful planning of a cardiac catheterization involves the precise selection of the examination catheters to be used. A variety of different catheters are available for the different stages of the examination and for accessing the various cardiac chambers and vascular segments.
Flexible balloon catheter
These catheters have a small balloon at the tip that can be filled with air or carbon dioxide (Fig. 8.2). This balloon allows the tip of the catheter to float with or be carried by the bloodstream. Typical uses for these catheters include, for example, entry to the pulmonary artery from the right atrium and ventricle, or for an antegrade access to the aorta from the left ventricle (Fig. 8.3 and Fig. 8.4). Balloon catheters are very soft and flexible, but they can be temporarily stiffened and shaped with a guidewire and be moved along the wire into certain vascular segments or even against the bloodstream. Balloon catheters can have open or closed tips and are suitable for precise blood pressure readings (including pulmonary capillary occlusion pressure, wedge pressure), for angiography, or to determine the cardiac output by thermodilution (Swan-Ganz catheter).
Preshaped plastic catheters
These are predominantly catheters with an open tip and with a predefined typical shape that also gives them their individual names. Typical examples are the pigtail catheter, the right and left coronary catheter, or the multipurpose catheter (Fig. 8.2). Some of them have only one hole at the tip, but many catheters have several side holes at the end through which contrast medium can be injected using a high-pressure pump. Their predefined shape can be changed or temporarily modified by guidewires (Fig. 8.5 and Fig. 8.6). Since these catheters are more rigid than balloon catheters, they can be used to examine difficult-to-reach segments of the heart or blood vessels. However, the risk of injury is higher when using these catheters. Guidewires with soft, flexible ends are therefore often used for exact positioning.





8.5 Hemodynamic Monitoring
Hemodynamic monitoring is an essential component of cardiac catheterizations in childhood and for congenital heart defects. The blood pressure in the individual segments of the heart or blood vessel is transmitted through the hollow catheter via the continuous blood or fluid column to a pressure sensor and then on to the measuring system for analysis. The typical pull-back pressure curves are recorded when the catheter is moved through segments of the heart and blood vessels.
In addition, the catheters can be used to take a blood sample at individual segments of the heart or blood vessels for determining hemoglobin and oxygen concentrations. The cardiac output and shunt fraction can be determined from these values (see Chapter 8.5.2 and Chapter 8.5.3).
8.5.1 Pressure Curves
A standard examination includes measuring blood pressure in all segments of the heart and blood vessels that are probed and documenting pressure curves across valves when the catheter is withdrawn through valves, septa, or stenotic segments. Since there is usually a certain amount of variation from breathing, at least 10 pressure curves should be recorded, the mean values calculated, and artifacts eliminated.
Atrial pressure curves
These curves consist mainly of an A wave and V wave, corresponding to the atrial contraction (A wave) and atrial filling (V wave). The V wave is caused by an increase in pressure in the atrium from the venous backflow or from atrial filling after closure of the AV valve. In the right atrium, the A wave is usually larger than the V wave; vice versa in the left atrium. The pressure in the left atrium is normally higher than in the right atrium. However, if there is a large atrial septal defect, the pressure between the two atria is generally equalized. In addition to pressure curves, the mean pressure is also important (Fig. 8.7 and Fig. 8.8).
Wedge pressure (pulmonary capillary occlusion pressure)
This pressure is measured by advancing an open-tip balloon catheter into the pulmonary artery in the flow direction and inflating the balloon at the distal end of the artery. This prevents transmission of pressure from the pulmonary artery via the bloodstream. The pressure measured in this way corresponds with the pressure of the vascular segment located behind the catheter in the flow direction, namely the pulmonary tissue, the pulmonary vein, or the left atrium (Fig. 8.9). The E wave and A wave of the left atrium can also be seen here. If there is no stenosis in the pulmonary veins, the wedge pressure is equivalent to that in the left atrium (principle of communicating tubes). However, if there is a stenosis in the pulmonary vein, the wedge pressure is higher than the pressure in the left atrium.
Ventricular pressure curves
During ventricular systole, the ventricular pressure increases rapidly until it exceeds the diastolic pressure of the downstream vessel and the ventricle ejects blood. This is followed by a definite plateau, during which blood continues to flow into the vessel. Diastole then leads to a relaxation of the ventricle; the pressure approaches 0 mmHg, and now additional blood flows in from the atrium. After the end of diastole, there is an isovolumetric contraction of the ventricle before a new ejection phase starts (Fig. 8.10 and Fig. 8.11). This dip in the ascending segment of the ventricular pressure curve corresponds with the end-diastolic pressure. No mean pressure is calculated for the ventricle, as diastole is always 0 mmHg (exceptions are cardiomyopathy or tamponade, for example). The end-diastolic pressure of a ventricle is normally equivalent to the mean pressure of the upstream atrium. If this is not the case, there is a stenosis of the mitral or tricuspid valve.
Vascular pressure curves
The typical systolic–diastolic blood pressure curves that arise from vascular wall tone, systole, vascular resistance, and Windkessel function (Fig. 8.12) are documented in blood vessels. The normal arterial blood pressure varies depending on age and weight. The blood pressure in the pulmonary artery is identical for all age and weight groups with the exception of the period immediately after birth, when there is physiological pulmonary hypertension.






Systolic and diastolic pressures are elevated in a rigid vessel (e.g., due to atherosclerosis, scarring). If the volume of blood is large and the Windkessel function is absent (e.g., due to aortic insufficiency, patent ductus arteriosus [PDA]), the result is very high amplitude with low diastole (pulsus celer et altus). If the valve does not close, the diastolic pressure is usually considerably lower or is only 0 to 1 mmHg (e.g., pulmonary insufficiency) and may appear similar to a ventricular pressure curve.
Pull-back curves
For pull-back curves, blood pressure is measured continuously while the catheter is simultaneously pulled back through a segment of the heart or blood vessel. This allows the blood pressure curve in front of and behind an anatomical structure (e.g., valve) or stenosis to be determined (Fig. 8.13, Fig. 8.14, Fig. 8.15, Fig. 8.16). A pull-back pressure curve can be used to prove or rule out a pressure gradient.




The systolic and the middle pressure gradient can be measured in vessels with pressure differences. In valve stenosis the systolic pressure difference is usually measured between the ventricle systole and the systolic blood pressure of the downstream blood vessel.
Simultaneous measurements
In simultaneous measurements, pressures are recorded at the same time in different segments of the heart and vessels (Fig. 8.17 and Fig. 8.18). Simultaneous measurements are used in particular when a pull-back pressure curve is technically impossible or extremely difficult (e.g., mitral stenosis—wedge pressure or pressure in the left atrium and end-diastolic pressure in the left ventricle), or when a direct comparison between different vessels and heart segments is important (e.g., pulmonary pressure and aortic pressure for testing pulmonary vascular response).(Fig. 8.18. Normal reference values for pressures and saturation in an adult with a healthy heart are shown in Fig. 8.19)



8.5.2 Determining Blood Flow and Cardiac Output
Determining cardiac output is an integral part of cardiac catheterization and reliable, reproducible methods for measuring it are indispensable. Although cardiac output is affected to a certain extent by respiratory variability and also by the cardiac cycle, these minimal factors can be ignored and cardiac output is determined as the flow per time unit. There are two methods for determining cardiac output: the direct Fick principle, and the indicator dilution method. Both methods measure the pulmonary blood flow, which can be considered the equivalent of systemic blood flow if there are no intrapulmonary or intracardiac shunts. The small additional flow from the bronchial arteries is negligible.
The flow through a specific vascular bed can generally be measured using an indicator over a unit of time if the original and final concentrations are known.
The more of the added indicator that is removed from the blood or diluted per time unit, the higher the blood flow is. For accurate determination, the indicator must have been mixed thoroughly with the blood and there may no undetected inflow or outflow of the indicator. Usually the cardiac output in humans is approximately. 3.5 L/min per m2 body surface (± 0.7).
Direct Fick Principle
Pulmonary blood flow (QP)
The indicator for calculating cardiac output with this method is oxygen. Oxygen is consumed by the lungs during inspiration (this is the measurement profile, equivalent to pulmonary blood flow). A portion of the oxygen that was taken in through inspiration is supplied to the venous blood of the lungs. This process is called oxygen intake or oxygen consumption (V · O2). The oxygen content in the venous blood is called mixed venous oxygen content (MVO2), expressed in milliliters O2/100 mL blood. The higher oxygen content in the pulmonary veins after passing through the lungs is called PVO2. It is also expressed in milliliters O2/100 mL blood. This makes it clear that every 100 mL blood that flows through the lungs takes in the difference in the amount of oxygen between PVO2 and MVO2 while passing through the lungs. This amount is usually is around 25 to 50 mL per liter. If the amount of oxygen consumed by the lungs during inspiration is known (V · O2), the pulmonary blood flow (QP) can be calculated.
The 100 mL is multiplied by 10 to convert it to one liter so the cardiac output can be expressed in L/min.
Systemic blood flow (QS)
Since the two blood flows are connected in a series, the pulmonary blood flow, QP, is equal to the systemic blood flow, QS. If no systemic venous oxygen is added and no oxygen is removed, the pulmonary venous oxygen content (PVO2) is equal to the arterial oxygen content (SAO2). (This should not be confused with oxygen saturation, SaO2.) Hence the following formula is valid for calculating the systemic blood flow, QS:
Oxygen consumption (V · O2)
It is technically difficult, however, to calculate oxygen consumption (V · O2). Formerly, rebreathing bags were used for this purpose. Other methods use measurement instruments in ventilators but require intubation and anesthesia. In routine practice, standard tables are used that show oxygen consumption (V · O2) in relation to height and weight. In adults, oxygen consumption is usually around 200 to 250 mL/min. The formula below can also be used to estimate oxygen consumption:
where BSA is the body surface area.
Mixed venous oxygen content (MVO2)
Another problem is measuring the mixed venous oxygen content (MVO2). By definition, the oxygen content in the pulmonary artery is referred to as mixed venous oxygen content (MVO2). To measure it, the pulmonary artery must be probed with a catheter and blood taken there. Using the oxygen content in other large veins close to the heart, such as the superior vena cava (SVCO2) or the inferior vena cava (IVCO2) involves errors, because different blood streams from different tributary areas have different saturations. However, the oxygen content of the superior vena cava often corresponds to the mixed venous oxygen content. Therefore some centers use this oxygen content as MVO2. The following formula is commonly used to calculate the mixed venous oxygen content:
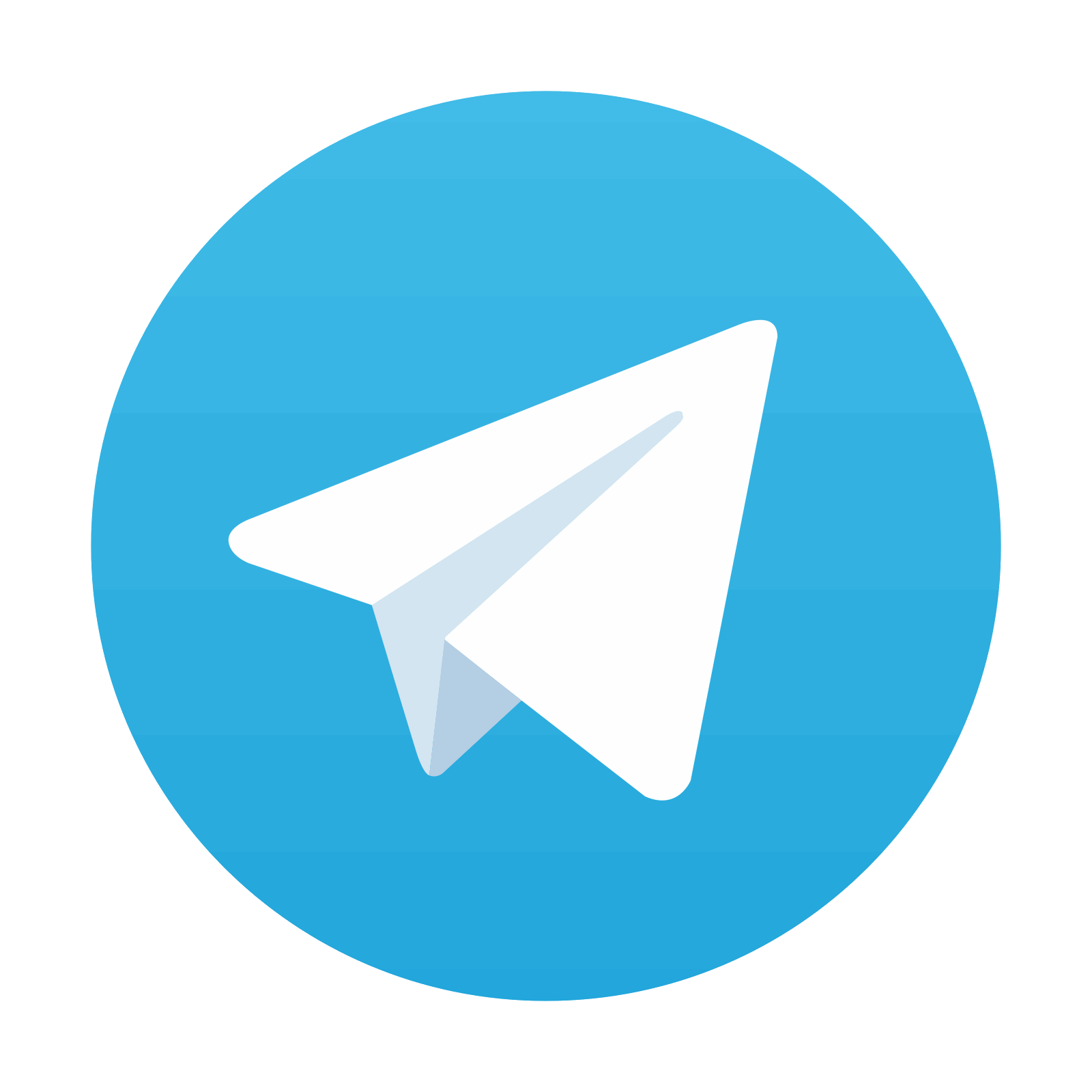
Stay updated, free articles. Join our Telegram channel

Full access? Get Clinical Tree
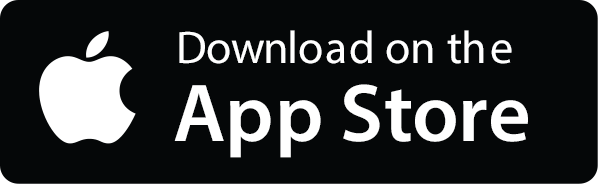
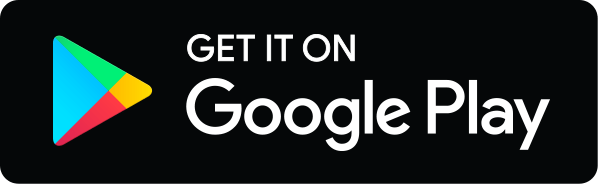
