Introduction
Seizures are common in intensive care patients generally and cardiac surgery or post-cardiac arrest patients are at particular risk. Seizures are an important and potentially reversible cause of prolonged unconsciousness as well as being associated with poorer ICU outcome, although the latter may be multifactorial. Cerebral metabolic rate is greatly increased during seizure activity, which may lead to energetic crisis and neuronal injury. Non-convulsive seizures are particularly common in ICU patients and are under-recognised. Electroencephalography (EEG) is therefore an essential ICU investigation for the diagnosis of seizures or epileptiform activity and for distinguishing these from other disorders of consciousness, which may have characteristic EEG signatures. Continuous EEG (cEEG) is particularly sensitive for clinically occult seizures and is also helpful for managing seizures refractory to simple treatment. However, EEG requires specific expertise to perform and interpret which can be a barrier to its use in the cardiothoracic intensive care unit.
The Burden and Characteristics of Seizures in Intensive Care
Seizures are common but under-recognised in the ICU. Subclinical electrographic seizure activity is common in neurosciences patients but may also occur in critically ill patients without a history of seizures. Most studies show a prevalence of seizures of 15–40% and identify the presence of seizures and status epilepticus as independent predictors of poorer outcome. To what extent this represents a modifiable pathology or an epiphenomenon of worse underlying neurological injury is uncertain. However, prolonged seizure activity is associated with neuronal energetic failure and injury. Therefore recognition and treatment of seizures is important. Furthermore, seizures are also an important reversible cause of unconsciousness and should be considered in the differential diagnosis of a patient who does not wake appropriately from sedation or anaesthesia.
Clinically apparent seizures are due to focal or global disorganised brain electrical activity and may be manifest as changes in behaviour/level of consciousness or abnormal movements. However, in sedated or critically ill patients, none of these findings may be clinically obvious or apparent. The diagnosis of true seizures is complicated by other types of abnormal movements that may be seen in intensive care patients (such as shivering, myoclonus, tremor, emergence from neuromuscular blockade). A systematic approach to distinguishing seizures from non-seizure movements is helpful (Figure 33.1).
Figure 33.1 The diagnosis and initial management of seizures after cardiac surgery. After Hunter and Young (2011), with permission. VPA valproate; DPH phenytoin; SRU seizures after cardiac surgery; LOC NYD altered level of consciousness not yet diagnosed.
Most clinical seizures are self-limiting. Patients will regain consciousness although a postictal period of altered mental state is common due to neurotransmitter depletion, changes in receptor concentration/inhibition or altered cerebral blood flow. Sometimes clinical seizures may be prolonged or repeated. Status epilepticus (SE) is defined as ‘an acute epileptic condition characterised by continuous generalised convulsive seizures for at least five minutes, or by two seizures without full recovery of consciousness between them’. Electrographic seizure activity may also occur without movement, and this is particularly important in ICU patients. Such non-convulsive seizures (NCS) are a more elusive diagnosis that is frequently unrecognised. Its incidence after cardiac surgery is probably particularly high due to exposure of the patient to some degree of subclinical embolic phenomena, metabolic derangement, hypotension or proconvulsive drugs. Non-convulsive SE (NCSE) is further defined as an electrographic diagnosis of ‘continuous or intermittent ictal discharges without the patient regaining consciousness, and no overt clinical signs of convulsive activity’. NCSE is often suspected in patients who were in convulsive SE and subsequently do not fully regain consciousness. Hence, if coma persists after SE, EEG is necessary to identify the cause of unconsciousness (NCSE or a postictal state). However, it may occur de novo in ICU patients and this represents a particular diagnostic challenge.
NCS have been reported in up to 37% of critically ill patients undergoing cEEG monitoring. The highest proportion of NCS is seen in patients who underwent emergency EEGs for altered mental status and clinically suspected NCSE. Studies prospectively performing EEGs in unselected comatose patients without clinical signs of seizures found either NCS or NCSE in up to 8% of cases.
Electroencephalography
Given the diagnostic difficulties presented by critically ill patients, the unequivocal detection/exclusion of seizures often requires EEG. This is a technique that measures the spatial distribution of voltage fields on the scalp and their variation over time. The origin of this activity is thought to reflect the fluctuating sum of excitatory and inhibitory postsynaptic potentials that arise primarily from apical dendrites of pyramidal cells in the outer layer of the cerebral cortex under the input from subcortical structures.
The potentials recorded by the EEG are very small (of the order of microvolts) and are therefore easily contaminated by electrical noise and other artefacts such as the electromyogram when trying to record from areas with larger underlying muscles. Furthermore, EEG changes may be relatively localised, meaning that an array of electrodes is needed to cover the whole scalp adequately. Finally, seizures may occur intermittently and so continuous recordings (cEEG) over 12–24 hours or even longer are needed to realise the highest diagnostic sensitivity. Such considerations make recording a clinically useful EEG much more complex and involved than, say, an ECG. The American Clinical Neurophysiology Society has published recommendations for recording ICU cEEG. Equipment, length of recording and electrode montages should meet the technical standards defined in these consensus statements (Figure 33.2).
Figure 33.2 Left panel: typical EEG scalp electrodes for clinical use. Right panel: international 10–20 system for standard scalp electrode placement. The distances between adjacent electrodes are either 10% or 20% of the total anterior/posterior or left/right distance. Left and right are distinguished by odd and even numbers respectively. Each channel of the EEG is obtained by measuring the voltage between adjacent electrodes (bipolar montage, such as here) or of individual electrodes compared to a reference electrode or combination of electrodes (referential montage).
Even in the absence of seizures, the EEG shows stereotypical changes with consciousness, which can be diagnostically useful. EEG activity can be classified by frequency, amplitude, distribution or location, symmetry, synchrony, reactivity (to external stimulation), morphology, rhythmicity and regulation. The signal frequencies detected by a standard clinical EEG are divided into four standard frequency ranges or ‘bands’: alpha (8 to <13 Hz, usually occipital), beta (>13 to 25 Hz, usually frontal and central), theta (4 to <8 Hz, usually central or diffuse) and delta (<4 Hz, focal or diffuse).
The most important EEG pattern of wakefulness is a posterior dominant rhythm (PDR). The PDR is located predominantly at the occipital poles but becomes anterior as the patient becomes drowsy (normal sleep). This activity is normally in the alpha range and is symmetrical. Slower rhythms such as generalised continuous delta are always abnormal and are usually associated with diffuse or multifocal cortical injury or metabolic derangement. Intermittent slowing, such as intermittent rhythmic delta activity (IRDA) and frontal IRDA (FIRDA) or occipital IRDA (OIRDA) are thought to be caused by dysfunction in subcortical centres influencing cortical activation and may represent a manifestation of a more generalised process not limited to the frontal or occipital lobes.
Reactivity refers to a clinical (electromyographic activity, respiratory pattern change) and/or EEG response (increased continuity, amplitude reduction, frequency change) to external stimulation (pain, passive eyes opening, auditory stimuli). In cardiac arrest patients, a non-reactive EEG background after rewarming is associated with poor neurological outcome. However, the association is complex: this pattern is still compatible with good recovery when observed during therapeutic hypothermia and patients with myoclonus and no EEG reactivity within 72 hours from cardiac arrest have occasionally gone on to have good outcomes.
Electrographic seizures are characterised by repetitive or rhythmic focal or generalised epileptiform discharges at greater than 3Hz, and lasting more than 10 seconds or at less than 3Hz but with clear evolution in frequency, location, waveform or field or clinical manifestation. They usually resolve or improve after administration of rapid acting intravenous antiepileptic drugs such as benzodiazepines. The electrographic features of SE are highly variable, including rhythmic, generalised and symmetric spike-and-waves or polyspikes-and-waves at 2 to 3.5Hz, or atypical spike-and-wave with lower frequency and less symmetry, or multiple spike-and-wave, or high voltage, repetitive, rhythmic (focal or generalised) delta activity with interspersed spikes, sharp waves or sharp components (Figure 33.3).
Figure 33.3 Examples of EEGs from the ICU. Top left: normal wakeful EEG, high frequencies with alpha wave activity dominant in posterior/occipital channels. Top right: encephalopathic EEG illustrating prominent slow (delta wave) activity. Bottom left: generalised seizure activity with sharp spikes and waves in all channels. Bottom right: LPDs, periodic spike discharges over the left hemisphere (odd numbered channels).
If continuous EEG is not available, multiple intermittent serial recordings are desirable as seizures may otherwise be missed, especially as high dose anaesthetics are weaned. Whilst the EEG does not change significantly at body temperatures between 32 and 34°C, sedative drugs commonly used during therapeutic hypothermia can markedly affect the EEG background.
Regular electrode maintenance, scalp inspections, keeping the scalp dry and reducing pressure on the scalp from the electrodes is important to avoid scalp injuries. Sweating and scalp breakdown are more common in patients with fever, sepsis and prolonged systemic disease requiring frequent changes of electrodes. Hypothermia presents a theoretically increased risk of coagulopathy. However, scalp breakdown with bleeding or infection has not been reported among postarrest patients treated with therapeutic hypothermia (even up to several days of cEEG with either disposable or reusable versions of disc electrodes, Figure 33.2).
The EEG is typically recorded for later off-line analysis by a neurophysiologist. To this end the use of simultaneous video recording is recommended to identify sources of artefact and must be correctly repositioned when the staff moves either the patient or the camera of the EEG acquisition unit. Routine ICU care such as physiotherapy, suctioning and oral care can create rhythmic artefacts that mimic electrographic seizures but are easily identified with video analysis.
EEG electrode technology is sophisticated. The low voltages involved mean that very low electrical impedances are required. Poor contact renders the electrodes susceptible to electrical interference. Electrical noise from intravenous pumps, electrical beds, dialysis machines and other medical devices can obscure the EEG recording and requires proper identification and trouble-shooting.
Specialised hardware and software increase the utility of cEEG for monitoring at the bedside. Options include the ability to enter nursing notes, pushbuttons for seizures and other clinical events, software to integrate physiological data, and quantitative EEG software for graphical display of quantitative EEG trends.
Causes and Prognostic Significance of Seizures in Intensive Care
The dominant aetiology and prognostic significance of seizures in the cardiothoracic intensive care unit varies from patient to patient. Three distinct patient populations are of particular importance in the cardiac ICU: those who are post cardiac surgery (where embolic phenomena are likely to be important), patients successfully resuscitated from cardiac arrest (where hypoxic-ischaemic injury dominates) and those patients suffering from general critical illness.
Postcardiac Surgery
Postoperative seizures are known to complicate cardiac surgery with an incidence of up to 7% and recurrence rates of around 50%. This is almost certainly an underestimate, with NCS probably frequently missed and not necessarily benign. The manifestations and causes of seizures in the context of cardiac surgery are diverse, as are the expected outcomes. Both convulsive and non-convulsive seizures may contribute to prolonged reduced levels of consciousness, an increased length of stay in the ICU, and a possible increase in morbidity and mortality. Furthermore, convulsive seizures lead to cardiovascular strain and metabolic derangement in patients already in a fragile state.
Perioperative seizures may be caused by thromboembolic ischaemic stroke, cerebral air embolism, antibiotic toxicity or other perioperative drugs such astranexamic acid (TXA). Several risk factors have been directly associated with the presence of postoperative seizures. Open-chamber procedures, including valve and aortic repairs and cardiac transplants, have a higher incidence of complications than does coronary artery bypass graft surgery. The combination of TXA administration, especially high doses (>80 mg/kg) and open-chamber surgery has been confirmed in several studies as an important perioperative factor. Higher preoperative creatinine (>120 μmol/l), thoracic aortic surgery and early seizures onset (<4 hours) have also been found to increase risk of recurrent seizures (RS). Despite longer ICU stay and duration of mechanical ventilation, the presence of RS is not associated with significantly increased long-term morbidity or mortality.
Postcardiac Arrest
Clinical seizures occur in up to 40% of patients following cardiac arrest, mostly in the first 3 to 5 days. Seizures most commonly present as diffuse or multifocal myoclonus or focal and generalised tonic-clonic seizures, and contribute to decreased level of consciousness; they have important implications for prognosis.
Non-convulsive seizures may occur and often present as NCSE.
NCSE after cardiac arrest is very difficult to treat, and it is not clear if treatment improves neurological outcome or mortality. However, it is common: postarrest patients treated with therapeutic hypothermia and receiving routine EEG or cEEG monitoring have a prevalence of NCSE/SE of around 30%. Clinical seizures typically begin within the first 2 days following cardiac arrest but it is not uncommon to see earlier electrographic seizures on the EEG. Several studies have shown that initial electrographic seizures are typically not associated with any clinical correlate of myoclonus or other motor seizure types by day 2 to 3.
Myoclonus can also occur in patients with hypoxic brain injury and may be either acute or chronic. Acute post-hypoxic myoclonic status epilepticus (MSE) occurs soon after a hypoxic insult and is a clinical diagnosis consisting of unrelenting diffuse myoclonus involving the face, limbs and torso that is often precipitated by stimulation. It is strongly associated with poor outcome if onset is during the first 24 hours postarrest, and this association remains true with therapeutic hypothermia, although the onset may be delayed until 3 to 5 days after cardiac arrest. It is important to appreciate that although rare (less than 5%) there have been reports of survivors of MSE with good cognitive outcome. Most of these patients were treated with at least three to four antiepileptic drugs (including high dose anaesthetics), had preserved brainstem reflexes, intact cortical somatosensory evoked potential responses and reactive EEG backgrounds.
Subcortical structures are involved in MSE and therefore the EEG does not show the same electrographic features. Instead the EEG may show generalised periodic discharges (GPDs) and/or a burst suppression pattern. A brainstem origin of postanoxic myoclonus may be suspected when there is sequential activation of muscles innervated by cranial nerves (reticular reflex myoclonus) and characterised by non-specific EEG changes (non-epileptiform patterns such as diffuse alpha activity). By contrast, cortical action-reflex myoclonus instead presents with epileptiform discharges and/or polyspikes and typically (but not necessarily) affects a few muscles in a localised distribution.
MSE may be confused with chronic post-hypoxic myoclonus (Lance–Adams Syndrome, LAS). LAS is a distinct entity that may also appear after a period of cerebral hypoxia in patients with a more prolonged coma or period of sedation. In contrast to MSE, LAS develops later, days or weeks after the initial hypoxic event, and does not respond to anticonvulsant treatment. Crucially, however, such patients regain consciousness, but may exhibit muscle jerks affecting face, trunk or limbs often provoked by sensory stimuli and strikingly elicited by the willed voluntary action. LAS is rare, but its differentiation from MSE is important to avoid incorrect prognostication and for the institution of timely rehabilitation.
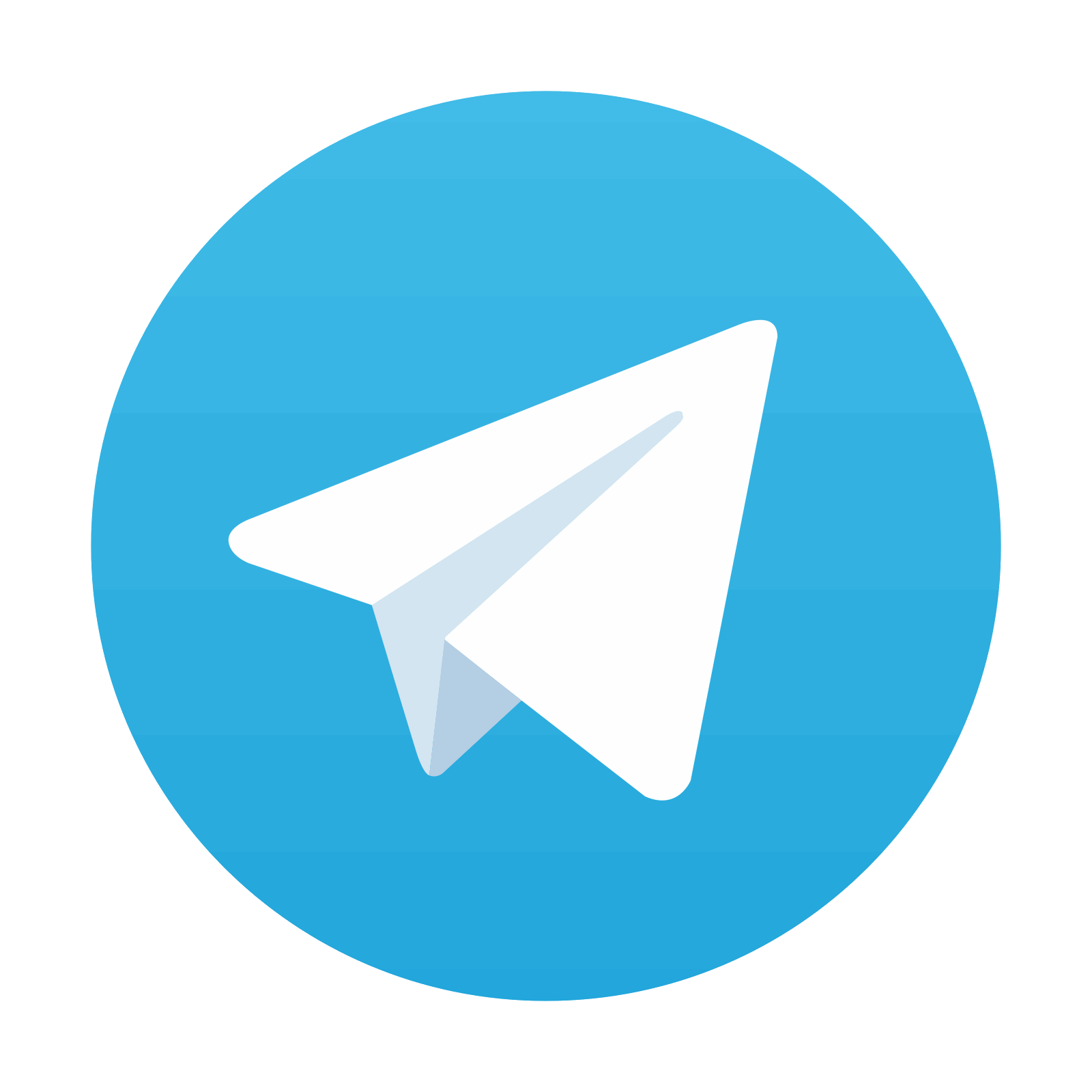
Stay updated, free articles. Join our Telegram channel

Full access? Get Clinical Tree
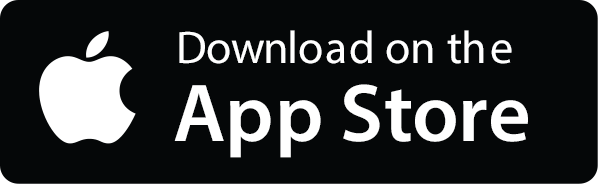
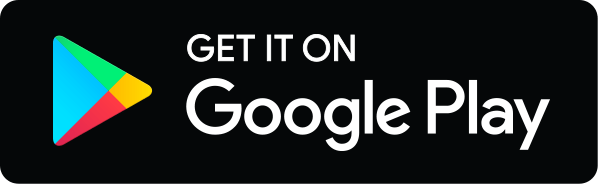
