As most patients after routine cardiac surgery quickly resume baseline nutritional intake, specific dietetic assessment and support is rarely required. However, patients with pre-existing malnutrition, especially underweight, and those requiring prolonged intensive care due to perioperative complications are at risk of further nutritional derangement and early interventions to optimise nutritional requirements are recommended.
Nutritional Assessment
At hospital admission patients should be assessed for baseline nutritional status. In the simplest and most useful form this includes:
Body weight, height and body mass index (BMI, kg/m2); with normal weight defined as a BMI between 18.5–24.9 kg/m2;
Physical examination with an emphasis on muscle mass and mobility to assess strength and coordination;
The presence of oedema, body temperature and oral health.
For example, elderly patients with poor muscle strength and oral health will be at increased risk of not being able to independently feed themselves during the recovery period. These patients and the critically ill intensive care (ICU) patients are likely to require either supplementary or full nutritional support.
To further identify patients at risk of malnourishment, screening questionnaires such as the Subjective Global Assessment (SGA), Nutritional Risk Screening (NRS 2002), the Malnutrition Universal Screening Tool (MUST) and the NUTRIC Score should be used.
Unless patients have specific metabolic diseases, biochemical indicators of nutritional status (e.g. plasma transferrin and 3-methylhistidine concentration) rarely offer additional information to a thorough clinical examination and assessment as outlined above.
If additional assessment is required, measurements of body composition can be applied to measure body fat content, lean body mass, bone mass and water content. Although some of these techniques, for example skin fold thickness to measure body fat and bioimpedance to measure water, fat and lean tissue, are simple to perform at initial hospital admission, most methodologies have not been validated for consecutive measurements in ICU patients. Hence, wrongly applying such techniques may at best obtain misleading results. Table 19.1 gives normal values for BMI and percentage body fat.
Table 19.1 Body fat and body mass index
Body fat (%) | BMI (kg/m2) | |||
---|---|---|---|---|
Males | Females | Normal | 18.5–24.9 | |
Normal | 12–20 | 20–30 | Overweight | 25–29.9 |
Borderline | 21–25 | 31–33 | Obese | 30–39.9 |
Obese | >25 | >33 | Severely obese | >40 |
More accurate methods of estimating body composition increase the complexity and may not be useful in clinical practice. Table 19.2 briefly summarises the principles behind some methods of estimating body composition.
Table 19.2 Principles of body composition measurements
Method | Principle |
---|---|
Imaging techniques (CT, MRI, DEXA scanning) | Soft tissue distribution and bone mass can be measured |
Density (body plethysmography, under water weighing) | Water or air displacement to calculate total body volume and density. From the density, body composition can be determined. Water has high density, fat has low density |
Bioelectrical impedance (BIA) | Electrical resistance varies across the body compartments. Water is a good conductor, fat less so. Impedance varies with body composition |
Stable isotope dilution techniques | Tracer isotopes are distributed to specific body compartments. Body compartment sizes are calculated from volumes and concentrations |
Normal Nutritional Requirements
Energy Balance
To achieve energy balance (energy intake − energy expenditure = energy balance), in a normal healthy individual an average daily energy intake is required of approximately 2500 kcal for men and 2000 kcal for women. It follows that if an individual remains in energy balance, body weight is stable.
Energy Intake
The total chemical energy of food (determined by bomb direct calorimetry) is not available to the human body. The energy available to the human body is termed the metabolisable energy. The difference in availability of energy is due to not all food being absorbed from the gastrointestinal tract, the energy costs of metabolism per se, incomplete oxidation of protein and energy losses through faeces and urine. Total energy intake is mainly distributed between the three macronutrients – carbohydrate, fat and protein. Excess energy intake compared with energy expenditure is stored as deposits of glycogen, fat and protein. Fat is the preferential and most efficient way of storing excess energy, as fat tissue has a high energy density with low cell turnover. This compares favourably with carbohydrates, as intracellular glycogen is obligatory stored with water and protein stores have high energy costs due to active metabolism and cell turnover. The body has no alcohol storage capacity and thus alcohol at 7 kcal/g must be preferentially metabolised before the three main macronutrients.
Table 19.3 demonstrates the metabolisable energy of the three macronutrients and the recommended daily energy intake.
Table 19.3 Metabolisable energy and recommended daily intake of macronutrients
Metabolisable energy (kcal/g) | Recommended daily energy intake, and percentage of total energy intake | |
---|---|---|
Carbohydrate | 4 | No more than 50% (of which free sugars no more than 5%) |
Protein | 4 | 0.8 g/kg/day (approximately 15%) |
Fat | 9 | No more than 35% (of which saturated no more than 11%) |
Total energy intake is measured by recording the amount and composition of food consumed. Published tables provide the energy content of food items and, correctly reported and collated, total energy intake can easily be determined.
Energy Expenditure
Energy expenditure is more difficult to measure compared with energy intake. The gold standard for measuring energy expenditure, and hence to enable individually tailored energy intake, is indirect calorimetry.
Indirect calorimetry is most often applied to measure resting metabolic rate (RMR), which is the minimum energy required by the body at rest in order to fuel essential bodily processes and keep organs and tissues in working order. It is a standardised measurement performed on an awake, supine resting person in a temperature neutral environment after overnight fasting (8–10 hours).
From measurements of oxygen consumption (VO2), carbon dioxide production (VCO2) and additional nitrogen excretion it is possible to accurately calculate total energy expenditure as well as macronutrient oxidation (protein, fat, carbohydrates) in health and disease. The most widely used formulae to calculate energy expenditure from indirect calorimetry in humans are those of Elia and Weir:
RMR (kcal/min) = [15.818 VO2 (l/min) + 5.176 VCO2 (l/min)]/4.18.
If nitrogen excretion is measured, accuracy is improved by applying
RMR (kcal/min) = [16.489 VO2 (l/min) + 4.628 VCO2 (l/min) − 9.079 N (g)]/4.18.
An assessment of substrate being metabolised can be obtained from measurement of the respiratory quotient (RQ), which is the volumetric relationship between carbon dioxide production and oxygen consumption, VCO2/VO2. An RQ approximating 1 indicates carbohydrate oxidation and an RQ approximating 0.7 indicates fat oxidation.
Predictive equations, based on age and anthropometry, which negate the requirements for equipment to accurately measure RMR, have been developed.
In health, the Mifflin–St Jeor equation is accurate 75% of the time when compared with individually measured indirect calorimetry:
RMR (kcal/day) = 10 × weight (kg) + 6.25 × height (cm) – 5 × age (years) + 5 (males),
RMR (kcal/day) = 10 × weight (kg) + 6.25 × height (cm) – 5 × age (years) – 161 (females).
In disease, the modified Penn State equation can be used to account for the metabolic effects of body temperature and minute ventilation:
RMR (kcal/day) = Mifflin × 0.96 + Tmax × 167 + VE × 31 − 6212 (males),
RMR (kcal/day) = Mifflin × 0.74 + Tmax × 85 + VE × 64 − 3085 (females).
Here Mifflin is the RMR as estimated by the Mifflin–St Jeor equation, Tmax is maximum body temperature (oC) in the previous 24 hours and VE is the minute ventilation in litres/minute.
Nutritional Requirements in Disease
Apart from a few exceptions (e.g. cachexia in severe mitral stenosis, poor outcome at extremes of BMI for lung transplant patients), cardiac patients do not differ from other patients undergoing major surgery and admission to the ICU. Sepsis, stroke, renal failure, long-term ventilation and bowel ischaemia are feared perioperative complications across a range of surgical specialities as well as for cardiac surgery. These are all complications that have a direct impact on the management of nutritional rehabilitation.
Compared with healthy individuals, the critically ill exhibit an increased turnover and mobilisation of stored fuel reserves, especially protein. Due to hormonal changes and inflammatory response in the ill patient, this happens even when energy balance has been achieved and thus the resting metabolic rate in what appears to be sedentary ill patients can be increased by an average of approximately 25% compared with healthy individuals. In patients with severe burns, RMR can be double baseline value.
The increase in metabolism caused by the inflammatory response to surgery and trauma is independent of the injury type when controlled for the effect of fever. Fever compounds the increase in metabolism and protein catabolism observed after major surgery, trauma and sepsis. The resting metabolic rate in these patients can increase up to 1.5 times compared with healthy individuals. In febrile patients, the metabolic rate increases by approximately 10% per degree increase in body temperature and in the most severely ill patients energy requirements can reach 40–50 kcal/kg/day against a normal figure of approximately 25 kcal/kg/day. During the acute febrile phase of infection there is additional salt and water retention, exacerbating oedema, renal and ventilatory complications. Thus, although serial measurement of body weight is recommended to monitor energy balance and changes in body weight, in clinical practice it is very difficult to accurately weigh intensive care patients, and with the often concurrent large disturbances in fluid balance, this is of little added value for assessing nutritional status per se.
The increased concentrations of cortisol and catecholamines, and the reduced effect of insulin, compound the often significant protein catabolism observed during a sustained inflammatory response. Urinary nitrogen losses (gram protein oxidised = 6.25 × gram nitrogen excreted) can be measured to assess the protein wastage, which in the severely ill patient can reach more than 200 g/day. The amino acids released from skeletal muscle are used for gluconeogenesis and protein synthesis, i.e. in disease there is an energy increase requiring acceleration of protein turnover and enhanced lipolysis.
Indirect calorimetry remains the most accurate method for measuring patients’ energy requirements. In the absence of this, predictive equations (based on the patient’s hospital admission body weight) with additions for disease pathology will in most cases provide adequate nutritional support. In clinical practice, however, a value of 25–30 kcal/kg/day is often used as the initial energy requirement for most critically ill patients
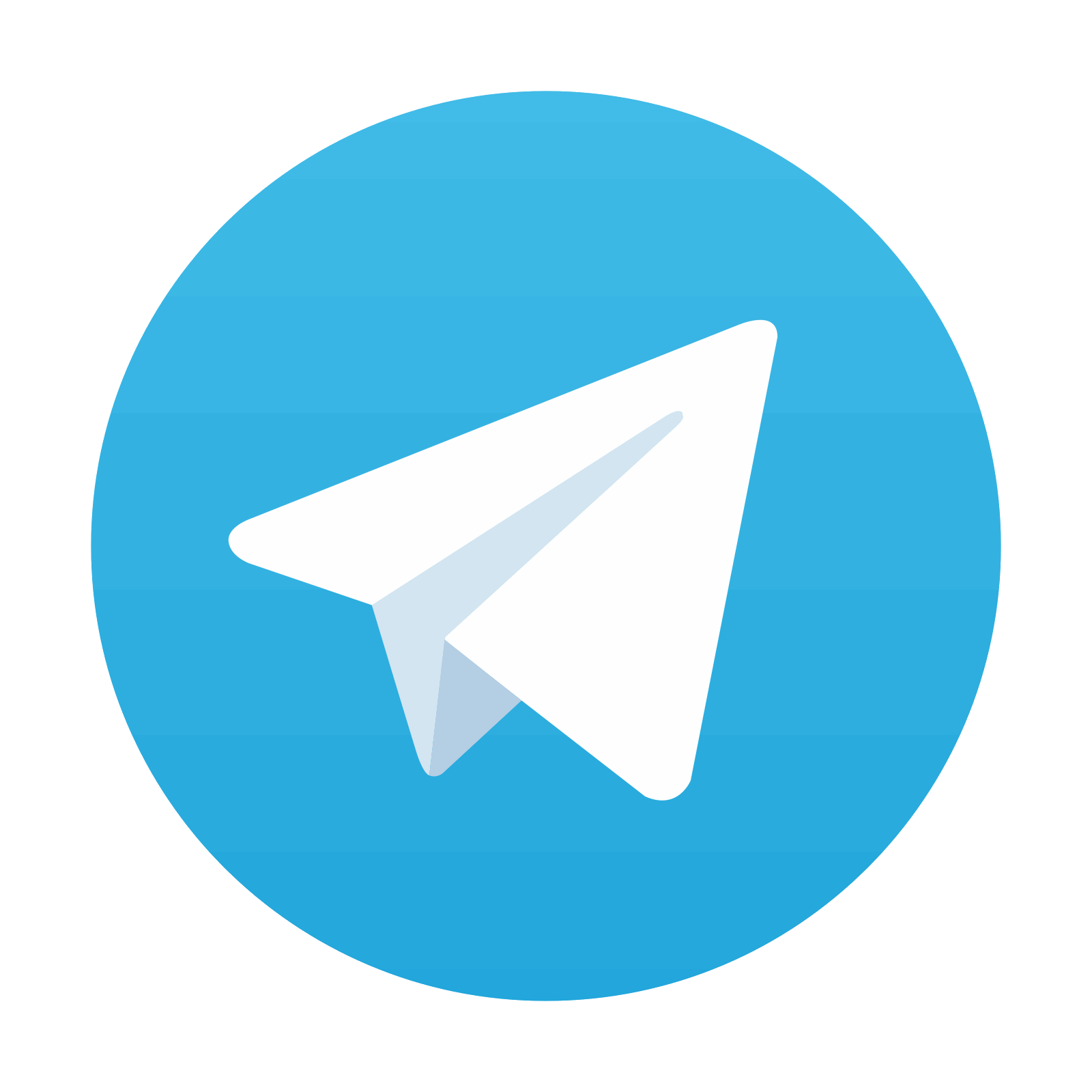
Stay updated, free articles. Join our Telegram channel

Full access? Get Clinical Tree
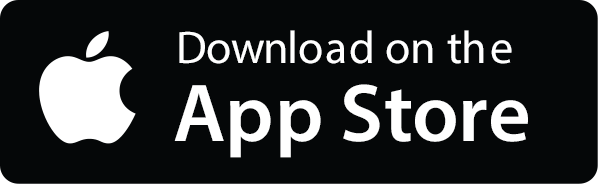
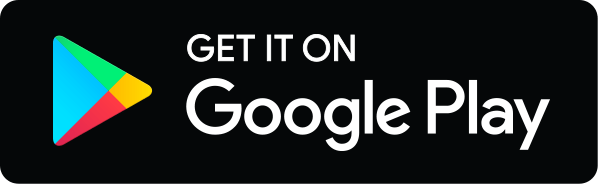