Introduction
Respiration is a complex process during which gas exchange occurs between the respiratory system of the patient and the surrounding atmosphere. The two major functions of the respiratory system are to oxygenate the blood and to clear carbon dioxide (CO2) through ventilation of the lungs. Appropriate respiration requires several intact physiological pathways including the central and peripheral nervous systems, the respiratory muscles, the cardiopulmonary system, and an appropriate oxygen carrying capability with red blood cells. Pathological disruption of any of these systems can result in respiratory dysfunction.
Dyspnoea (breathlessness) is a symptom and sign. It is described by patients as difficulty with either inspiration, expiration or both. As a sign dyspnoea presents with involvement of accessory respiratory muscles and general distress. Dyspnoea is frequently but not exclusively associated with respiratory failure. Dyspnoea can be caused by both physical and psychological stimuli.
Dyspnoea as a sign is frequently obvious and most often associated with tachypnoea. Use of accessory respiratory muscles is a common sign. Acute dyspnoea leads to fatigue due to increased work of breathing.
Acute respiratory failure (ARF) is defined as the inability to meet the metabolic requirements of the patient through a failure of either oxygenation or ventilation. ARF can therefore be divided into two distinct subtypes including hypoxaemic respiratory failure (type 1) resulting in low arterial oxygen (PaO2) or hypercarbic respiratory failure (type 2) resulting in elevated arterial CO2 (PaCO2).
ARF is a common indication for admission to the intensive care unit (ICU), and can account for 17–56% of all admissions.
The relationship between dyspnoea and acute respiratory failure is variable. Some patients suffer and display dyspnoea without respiratory failure, and vice versa, some patients can be hypoxic or hypercarbic without the symptoms and signs of dyspnoea. The match of the two entities can give a clue to the duration of the problem, adaptive mechanisms and homeostatic control of the patients.
Hypoxaemic Respiratory Failure
There are several pathophysiological mechanisms through which hypoxaemic ARF can occur, including inadequate alveolar oxygen concentration (PAO2), ventilation-perfusion mismatch (V/Q), shunt, diffusion abnormalities and increased venous admixture (Figure 28.1). The most common cause of hypoxaemia in ICU patients is V/Q mismatch.
Figure 28.1 Common aetiologies of respiratory failure within the ICU.
Inadequate Alveolar Oxygen (PAO2)
Inadequate alveolar oxygen is most often caused by either hypoventilation or a low partial pressure of inspired oxygen.
Hypoventilation results in hypoxaemia through two mechanisms: a reduction in the PAO2–PaO2 gradient that causes a fall in PaO2 due to acidosis from inadequately cleared CO2, resulting in a rightward shift of the oxyhaemoglobin dissociation curve and lower affinity of haemoglobin for O2.
The second mechanism of low alveolar oxygen is uncommon in the ICU population. It more commonly occurs at high altitudes where the partial pressure of the atmosphere is much lower than at sea level. It may also happen in an airplane where the cabin pressure is not pressurised to sea level but to a higher elevation (6000–10,000 feet).
The alveolar–arterial (A–a) gradient calculation is a measure of the efficiency of alveolar gas exchange and can be used to determine whether hypoventilation is the cause of a low PaO2 (Figure 28.2). The normal A–a gradient is 10–20 mmHg; it is influenced by age and increases with each decade of life. Hypoxaemia with a normal A–a gradient is suggestive of hypoventilation. The patient’s history can be used to rule out low inspired partial pressure of O2 in most cases, and the A–a gradient in this situation should be normal as well.
Figure 28.2 Calculation of an alveolar–arterial (A–a) oxygen gradient. R respiratory quotient (VCO2/VO2) = 0.8, PaO2 arterial oxygen concentration, FiO2 fraction of inspired oxygen, PaCO2 arterial CO2 concentration, PB (barometric pressure) = 760 mmHg at sea level, water vapour pressure = 47 mmHg.
Ventilation and Perfusion Mismatch
Ventilation and perfusion (V/Q) mismatch is the most common mechanism for hypoxaemia within the ICU. In a normal upright lung there is a degree of regional V/Q mismatch as the apices of the lung are ventilated more than perfused (high V/Q), in contrast to the bases of the lungs, which are perfused much more than ventilated (low V/Q). In aggregate the lung has an overall matched V/Q ratio of approximately 1. Body position, posture and lung volumes, as well as age, can influence V/Q ratios in normal lungs.
Any disease process that affects the lung parenchyma can influence V/Q ratios (Figure 28.3). For example low V/Q ratios are associated with processes that impair ventilation such as bronchoconstriction, or secretions from pneumonia. High V/Q ratios are associated with processes that impair perfusion such as pulmonary embolism, or that lead to increased ventilation due to large tidal volumes such as emphysema. The lung vasculature has mechanisms to attempt to match V/Q and maintain an equal ratio such as hypoxaemic pulmonary vasoconstriction, which results in reduced perfusion to those areas of the lungs which are poorly ventilated.
Figure 28.3 Pathophysiological mechanisms of V/Q mismatch.
Shunt
There are two types of shunts that may cause significant hypoxaemia: intrapulmonary and intracardiac.
Intracardiac shunts include such aetiologies as a patent foramen ovale or an atrial septal defect. These shunts will cause hypoxaemia as venous blood bypasses the lungs and travels from the right side of the heart directly to the systemic side.
Intrapulmonary shunt is an extreme V/Q mismatch where there is no ventilation of the associated lung unit despite being fully perfused. The most common cause of intrapulmonary shunt is atelectasis, and fluid filled alveoli due to cardiogenic or non-cardiogenic pulmonary oedema. In non-diseased states there is a small physiological degree of intrapulmonary shunt (up to 3% or the systemic cardiac output), which is due to circulation from the Thebesian and bronchial circulation.
Severe shunts (greater than 30% of systemic cardiac output) due to the lack of ventilation do not respond to supplemental oxygen therapy. This can be used as a diagnostic feature when assessing a patient suspected to have a shunt.
Diffusion Abnormalities
Diffusion of oxygen and CO2 across the alveolar-capillary membrane is an extremely efficient process. The ability of gases to diffuse efficiently is dependent upon several key features of the capillary-alveolar membrane including its total surface area, thickness, diffusability and the solubility of the gases. Conditions that can cause diffusion abnormalities include end stage pulmonary fibrosis or fibroproliferative acute respiratory distress syndrome (ARDS).
Increased Venous Admixture
Low oxygen content returning to the pulmonary circulation can be a cause of hypoxaemia. This is often in the context of poor cardiac function or very high tissue consumption and extraction of oxygen. When this is suspected as a primary cause of hypoxaemia, detailed evaluation of cardiac function is required.
Hypercarbic Respiratory Failure
Hypercarbic respiratory failure, also known as type 2 respiratory failure or acute ventilatory failure, results in a rise in PaCO2 above the patient’s baseline. In most patients the normal CO2 is between 35 and 40 mmHg.
CO2 production is matched by CO2 elimination in steady state conditions. Hypercarbia can result from three major pathophysiological mechanisms: increased CO2 production, decreased tidal ventilation or increased dead space.
Increased CO2 Production
This can occur in both physiological and disease state conditions such as exercise, fever, major trauma, sepsis and hyperthyroid states. The compensatory response to an increase in CO2 production is to increase alveolar minute ventilation by increasing both tidal volumes and respiratory rate, with the objective both of maintaining a normal CO2 and of preventing acidosis. Patients who do not have the ability or reserve to increase their alveolar minute ventilation (i.e. pre-existing neuromuscular disease) can develop hypercarbic respiratory failure.
Decreased Tidal Ventilation
Decreased tidal volume ventilation resulting in reduced alveolar ventilation can be caused by pathology anywhere from central or peripheral nervous system to the respiratory muscles, chest wall, pleural space and parenchyma. Common conditions in the ICU which may cause this include CNS depressant medications such as narcotics and intravenous sedation, brainstem strokes, neuromuscular disorders like myasthenia gravis, critical illness neuropathy and myopathy, circumferential chest burns with significant eschar, rib fractures with a flail segment, airflow obstruction due to severe emphysema, and central sleep disordered breathing.
Increased Dead Space
Increased dead space results from disease processes that increase areas of the lung that do not participate in gas exchange, despite being ventilated. Dead space can be both anatomical and alveolar.
Anatomical dead space comprises the trachea, main bronchi and the conducting airways that do not participate in gas exchange. An average adult has approximately 150 ml of anatomical dead space. In mechanically ventilated patients this is also increased due to the ventilator circuitry, endotracheal tube and heat-moisture exchanger. Tracheostomy can significantly reduce the amount of anatomical dead space in comparison to an endotracheal tube.
Alveolar dead space results when non-perfused alveoli are ventilated. This is often the sequelae of disease processes such as chronic obstructive pulmonary disease, pulmonary emboli or fibroproliferative ARDS.
Illustrative Clinical Scenarios in the ICU
Acute Respiratory Distress Syndrome
Acute respiratory distress syndrome (ARDS) is characterised by the acute onset of severe hypoxaemic respiratory failure with associated bilateral infiltrates on chest X-ray in the absence of significant left ventricular dysfunction (see Table 28.1). Initially described in 1967 in a series of 12 patients, ARDS has been the subject of significant clinical and research investigation due to the significant morbidity and mortality associated with the diagnosis. By most recent estimates, mortality for patients diagnosed with severe ARDS is over 40%.
The details of ARDS diagnosis and treatment will be discussed in Chapter 44. However, an important part of the diagnosis of this condition is to exclude a cardiogenic cause of pulmonary oedema.
Pneumothorax
A pneumothorax is the accumulation of air within the pleural space. It is most often caused by an acquired defect in either the parietal or visceral pleura. Pneumothoraces can be classified as either spontaneous or non-spontaneous. Spontaneous pneumothoraces are less common within the ICU in comparison to non-spontaneous pneumothoraces.
Spontaneous pneumothoraces most often occur in healthy young adults. Certain risk factors can increase the risk of a spontaneous pneumothorax such as underlying lung disease (i.e. emphysema), familial history, drug abuse or more rare causes such as catamenial pneumothorax.
Most pneumothoraces that are encountered in the ICU are not spontaneous and are trauma induced or iatrogenic. Iatrogenic pneumothoraces can be due to barotrauma from mechanical ventilation, or they can be procedure related (such as central venous catheter insertion, thoracentesis, transbronchial lung biopsy, percutaneous tracheostomy).
Mechanical ventilation induced pneumothoraces are often the result of barotrauma. Tissue disruption allows the passage of air along the bronchovascular bundle back to the hilum and into the pleural and pericardial spaces, as well as into other interstitial tissues such as subcutaneous chest, neck and abdominal tissues. Insufficient decompression via one of these routes can lead to rupture of the pleural space and subsequent pneumothorax.
Clinically, a pneumothorax should be suspected if a mechanically ventilated patient has an acute increase in peak and plateau airway pressures. If the pneumothorax is significant or causes obstruction to cardiac inflow or outflow via tension, there may be associated hypotension. Hypoxaemia is common as the pneumothorax results in collapse of the underlying lung. The chest X-ray can reveal a pneumothorax with evidence of a visceral pleural line and no lung markings beyond this. Radiography in the upright position and at end expiration may improve the successful diagnosis of pneumothorax by chest X-ray. Patients with a pneumothorax may have chest X-rays supine and as a result pleural air rises to the anterior costophrenic sulcus resulting in a deep visible costophrenic angle and double diaphragm, known as a deep sulcus sign.
Treatment of pneumothorax varies depending on the severity and may include expectant management, supplemental high concentration oxygen, aspiration and tube thoracostomy. High concentration supplemental oxygen increases the rate of pneumothorax resolution. An increase in the partial pressure of oxygen in the pleural space results in a reduction in partial pressure of nitrogen in the pleural space. Oxygen is more readily absorbed and results in increased reabsorption of pleural gas. In large or unresolving pneumothoraces, tube thoracostomy can be performed either with a large bore chest tube, or via percutaneous small bore chest tube.
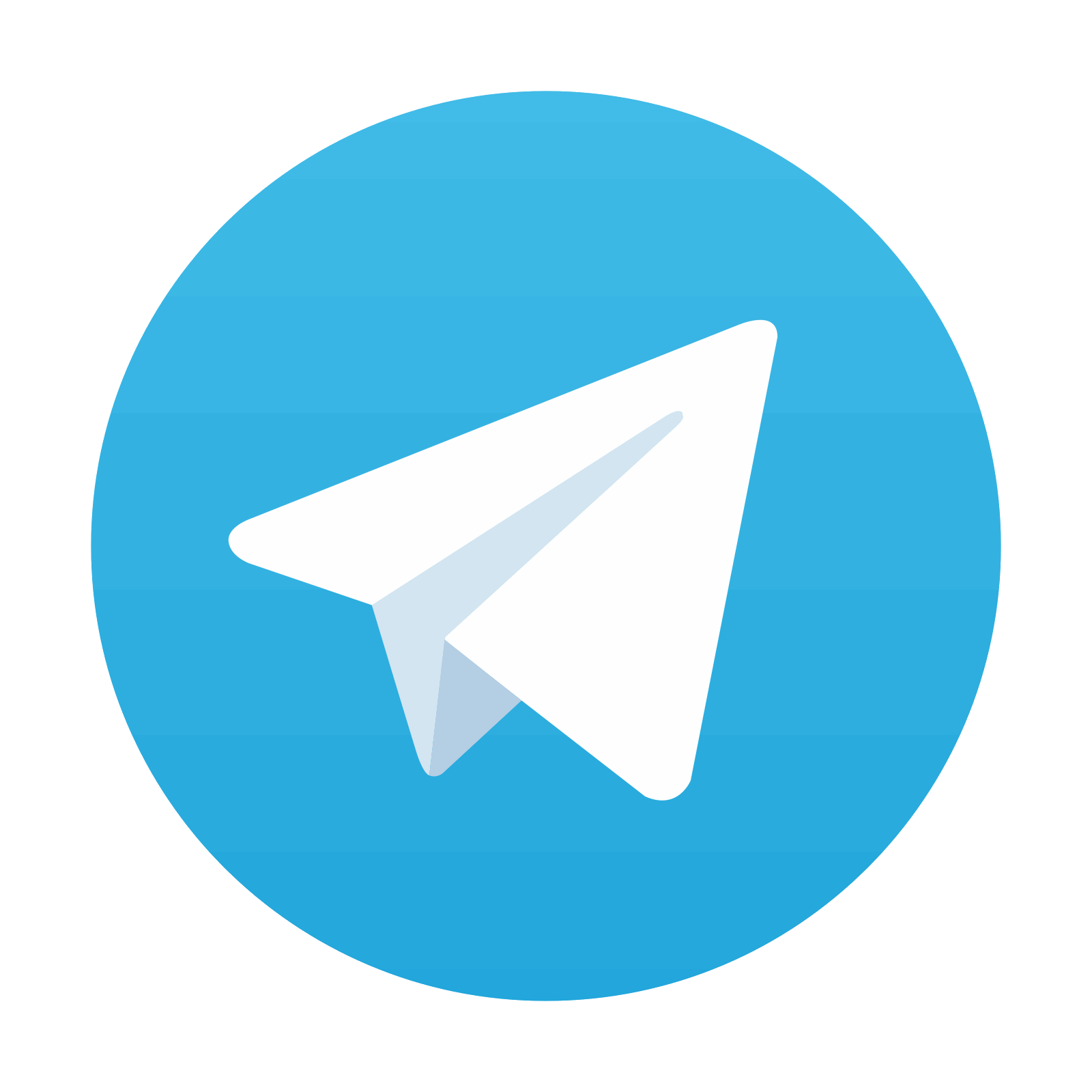
Stay updated, free articles. Join our Telegram channel

Full access? Get Clinical Tree
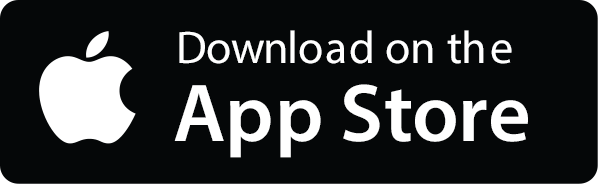
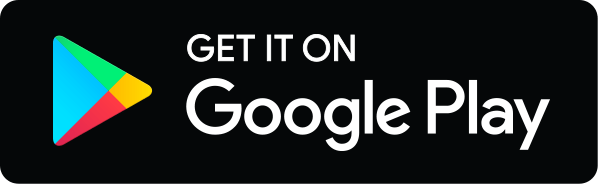
