Introduction
Analgesia and sedation are ubiquitous in the management of the intubated ICU patient, and attitudes towards them have undergone a paradigm shift in recent years. Where once the ICU was viewed as an extension of the operating room environment, the ‘anaesthetic triad’ of deep sedation, potent analgesia and neuromuscular blockade is now rarely indicated beyond the doors of theatre.
The problems of delirium, agitation and pain are intrinsically connected. It is perhaps best to conceptualise this ‘ICU triad’ as a three-legged stool – if any ‘leg’ is neglected, the stool cannot function as intended.
Infusion Pharmacokinetics
The administration of sedative and analgesic medications in the ICU is frequently via intravenous infusion.
When administering any drug via continuous intravenous infusion, a steady state concentration will eventually be achieved, as determined by the dose rate and the clearance of the drug.
The time taken for an infusion to reach this steady state is determined by the half-life of the drug (the time taken for the plasma concentration of the drug to decrease by 50%). It takes 3–5 half-lives for a drug to reach steady state.
For agents with a prolonged half-life, an increase in the infusion rate is an inefficient means of achieving desired depth of sedation within a short timeframe. Increasing the infusion rate to a level such that the target concentration is achieved quickly will result in a relative overdose if the infusion is allowed to continue at that rate (Figure 16.1).
Figure 16.1 The effects of increasing the rate of infusion to achieve a rapid clinical effect with a hypothetical drug with a half-life of approximately 4 hours. An increase in the infusion rate (from a steady state of 0.5 mg l−1) is performed. The desired clinical effect is achieved relatively rapidly, within 15 minutes (at a blood concentration of 1 mg l−1).
However, due to the prolonged half-life of the drug, the blood concentration steadily rises over the next 16 hours, a situation which, if left unchecked, will result in a steady state blood concentration nine-fold that of the original level, with potentially deleterious effects.
To achieve the target concentration of a drug whilst maintaining a continuous infusion at a safe dose rate, a loading dose is used.
If the loading dose is sufficient to achieve the target concentration for a given infusion rate, then the target concentration will be immediately achieved and maintained by the infusion without needing to wait the requisite 3–5 half-lives. If the loading dose yields a drug concentration more or less than the target concentration, it will still take 3–5 half-lives for steady state to be reached, but the starting concentration will be closer to the steady state concentration than if the loading dose had not been given (Figure 16.2).
Figure 16.2 This graph demonstrates the effects of a loading dose for a hypothetical drug with a half-life of approximately 4 hours. Curve A demonstrates the effect of no loading dose, and the target steady state of 4 mg l−1 is reached after 4 half-lives. Curve B shows the effect of a perfectly calculated loading dose – the target steady state is reached immediately, and maintained by the infusion. Curve C shows the effect of a partial loading dose. The starting concentration is higher, but it still takes 4 half-lives until steady state is reached. Finally, curve D shows the effect of an excessive load. The starting concentration is extremely high, and takes 4 half-lives to fall to the target steady state concentration. Drug concentration in mg l−1.
Sedation
Oversedation is an important cause of morbidity in the ICU. The initiation of a sedative or analgesic agent should not be based on anticipated distress but rather on that which is perceived by the clinical team at the bedside.
In the postcardiac surgery setting, sedation may be misused to mask a variety of problems without considering the underlying issue (particularly pain). With a few exceptions (status epilepticus, raised intracranial pressure and severe respiratory failure), it is recognised that maintaining light levels of sedation leads to improved clinical outcomes (i.e. shorter duration of mechanical ventilation, decreased length of ICU stay). Whilst lighter levels of sedation lead to a more pronounced physiological stress response, this has not been linked to an increased incidence of myocardial ischaemia.
Consequently, it is vital to identify the underlying cause of any distress, rather than resorting to sedation in the first instance. Anxiety, pain, delirium and residual neuromuscular paralysis are amongst the most common causes in the intensive care setting. A common underlying cause of anxiety amongst this population is patient-ventilator dys-synchrony, and the adjustment of ventilator settings (despite acceptable blood gas values) may negate the need for sedation.
Non-pharmacological strategies are equally important. Whilst attention must be paid to addressing the cause of agitation and distress, frequent reassurance and communication is equally important. Regular family visits, preservation of regular sleep-wake cycles and cognitive-behavioural therapies (music therapy, relaxation therapy) where available should also be incorporated into patient care.
Monitoring Sedation
A variety of different structured, subjective sedation assessment scores have been proposed. Of these, the Richmond Agitation-Sedation Scale (RASS) (Table 16.1) and the Sedation-Agitation Scale (SAS) (Table 16.2) are the most psychometrically reliable (particularly in terms of inter-rater variability) in measuring the quality and depth of sedation in adult ICU patients. In direct comparisons, neither of these measures is clearly superior.
Table 16.1 The Richmond Agitation-Sedation Scale (RASS)
Score | Classification | Description |
---|---|---|
4 | Combative | Overly combative or violent; immediate danger to staff |
3 | Very agitated | Pulls on or removes tube(s) or catheter(s) or has aggressive behaviour towards staff |
2 | Agitated | Frequent non-purposeful movement or patient-ventilator dys-synchrony |
1 | Restless | Anxious or apprehensive but movements not aggressive or vigorous |
0 | Alert and calm | |
−1 | Drowsy | Not fully alert but has sustained (more than 10 seconds) awakening with eye contact to voice |
−2 | Light sedation | Briefly (less than 10 seconds) awakens with eye contact to voice |
−3 | Moderate sedation | Any movement (but no eye contact) to voice |
−4 | Deep sedation | No response to voice, but movement to physical stimulation |
−5 | Unarousable | No response to voice or physical stimulation |
Table 16.2 The Sedation-Agitation Scale
Score | Classification | Description |
---|---|---|
7 | Dangerous agitation | Pulls at endotracheal tube, tries to remove catheter, climbs over bed rail, strikes at staff, thrashes side to side |
6 | Very agitated | Does not calm despite frequent verbal reminding of limits, requires physical restraints, bites endotracheal tube |
5 | Agitated | Frequent non-purposeful movement or patient-ventilator dys-synchrony |
4 | Calm and cooperative | Is calm, awakens easily, follows commands |
3 | Sedated | Is difficult to arouse, awakens to verbal stimuli or gentle shaking but drifts off again, follows simple commands |
2 | Very sedated | Arouses to physical stimuli but does not communicate or follow commands, may move spontaneously |
1 | Unarousable | Has minimal or no response to noxious stimuli, does not communicate or follow commands |
A variety of different devices have been evaluated for the objective measurement of cerebral function in the sedated ICU patient. These include:
Bispectral index (BIS);
State entropy (SE);
Auditory evoked potentials (AEP);
Narcotrend index (NI);
Patient state index (PSI).
Whilst these have some use in patients who cannot be clinically assessed (i.e. patients receiving neuromuscular blockade), or in whom monitoring of EEG is particularly important (i.e. non-convulsive status epilepticus), it is generally recognised that they are inferior to subjective scoring systems in the non-paralysed, non-comatose patient.
Planning Sedation
As previously mentioned, lighter levels of sedation lead to improved patient outcomes when combined with effective analgesia. This has led to the advent of protocolised sedative-analgesic strategies, whereby the bedside clinician titrates sedation to a set of predefined goals, guided by routine, structured, subjective assessments. The ability to undertake such intensive monitoring is dependent on bedside staffing and training, but it is effective in orientating practice towards sedation minimisation.
Another strategy is the use of daily sedation interruption (DSI). This involves ceasing sedation for a period to allow comprehensive, formal assessment of underlying neurological function. The use of this technique in isolation has met with controversy in the literature. If a unit is already practising sedation minimisation and/or protocolised sedation, the use of DSI offers little benefit.
Despite years of use, the common agents used for sedation (propofol and benzodiazepines) in the ICU have only recently been assessed by large, randomised controlled trials, many of which have significant limitations. This renewed interest came about as a result of the introduction of dexmedetomidine. Sodium thiopentone and the other barbiturates are rarely used outside of neurosurgical intensive care. They will not be discussed in this chapter.
In general, the choice of sedative agent is driven by:
1. The specific clinical circumstances and sedation goals for the individual patient including haemodynamic compromise and end-organ impairment;
2. The pharmacokinetic profile of the agent, including half-life, context sensitive half-time, metabolism and active metabolites, clearance and side-effects; and
Benzodiazepines
These agents act at the α/γ subunit of the GABAA receptor to produce sedative effects, mostly by enhancing neurotransmitter binding. They exhibit anxiolytic and skeletal muscle relaxant properties, although their sedative-hypnotic effects are not as pronounced as those of some other classes of agent. Cheap and readily available, there is some evidence that protracted use can lead to prolongation of mechanical ventilation and length of ICU stay, resulting in diminished popularity especially when sedation is required for more than 96 hours. They all demonstrate a similar side effect profile, with respiratory depression and systemic hypotension being the major manifestations. These are often seen when benzodiazepines are used in combination with other sedating agents. All benzodiazepines undergo extensive hepatic metabolism by cytochrome P450 enzymes, particularly CYP3A4 and CPY2C19. This leads to prolonged action in low cardiac output states due to diminished hepatic extraction.
Midazolam
Midazolam is possibly the most popular of the benzodiazepines, with a relatively short median half-life of 6.6 hours. It rapidly equilibrates across the blood-brain barrier, with duration of action of approximately 30 minutes after a bolus dose. Accumulation in the setting of prolonged infusion is a common problem, especially in the elderly or in renal and hepatic failure. Metabolised by CYP3A4 to the equipotent active metabolite α-hydroxy-midazolam, it competes with other drugs (particularly fentanyl) for elimination.
Diazepam
No longer used as an infusion in the ICU setting as it binds to PVC, diazepam has an elimination half-time roughly ~20-fold that of midazolam (20–50 hours), leading to gross prolongation of effect relative to other sedatives. This issue is further compounded by its multiple active metabolites, including desmethyldiazepam, n-methyloxazepam and oxazepam. It cannot be removed by dialysis and is extremely irritant when extravasated.
Lorazepam
More potent than midazolam or diazepam, lorazepam is less lipid soluble, with a slower onset of effect following a bolus dose. Uncommonly used in the ICU setting relative to midazolam, the major advantage of lorazepam is the lack of active metabolites, theoretically leading to more rapid offset when the drug is ceased, although the elimination half-life is still 8–15 hours. The clinical effects and duration of action are still prolonged by hepatic dysfunction. Parenteral formulations of lorazepam contain propylene glycol, which has toxic effects. Thought initially to only occur with very high doses, it has been seen with cumulative doses as low as 1 mg kg−1 day−1, manifesting as renal dysfunction and metabolic acidosis. Both of these occur frequently in the postcardiac surgical patient, and hence propylene glycol toxicity may be overlooked as a cause.
Propofol
Propofol is an intravenous sedative that has a number of useful properties for use in the ICU. It demonstrates sedative, hypnotic, anxiolytic, amnestic and anticonvulsant effects, but is not an analgesic. Whilst acting as a GABAA agonist, it also acts at a number of other receptors in the CNS, such as glycine, nicotinic and muscarinic cholinergic receptors.
As a sedative, propofol is characterised by a relatively rapid offset of action. This is due to its high lipid solubility and redistribution away from the site of action, but also its unusual hepatic and extra-hepatic clearance. This results in a short context sensitive halftime (despite a prolonged duration of infusion), allows for rapid changes in sedation depth and facilitates daily sedation interruptions.
Despite its many positive qualities, the use of propofol is not without risk, particularly in the postcardiac surgery patient. Similar to other sedative agents, it causes dose dependent respiratory depression, and hypotension due to systemic vasodilation. These changes are more pronounced in the patient with pre-existing circulatory instability.
The propofol infusion syndrome (PRIS) is caused by the inherent mitochondrial toxicity of the drug, and resembles the mitochondrial myopathies. The underlying pathophysiology is impaired entry of long-chain fatty acids, disruption of fatty acid oxidation and failure of the respiratory chain. Manifestation is uncommon, but presents with an unexplained metabolic acidosis, hypertriglyceridaemia, arrhythmia and escalating vasopressor and inotrope requirements. The risk of PRIS is increased by high infusion rates (>70 μg kg−1 min−1) over prolonged periods, but it has been reported with low dose infusion. The incidence of PRIS has been estimated to be as high as 1%. The syndrome is difficult to diagnose as these patients frequently have multiple aetiologies for clinical instability. Management involves early recognition and cessation of the infusion, followed by supportive care. This may not be sufficient to arrest its progress, and consequently, PRIS has a mortality of 33% in some series.
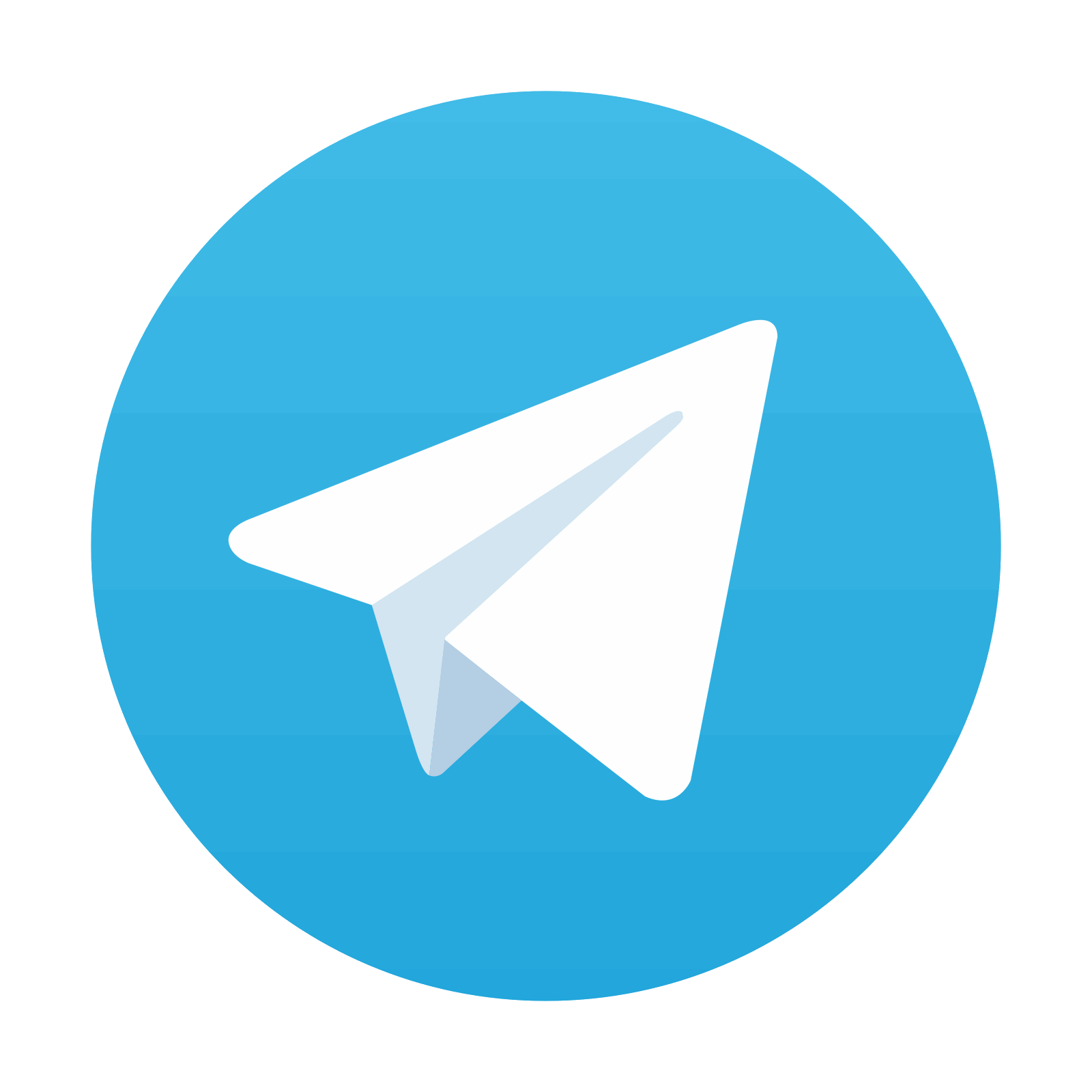
Stay updated, free articles. Join our Telegram channel

Full access? Get Clinical Tree
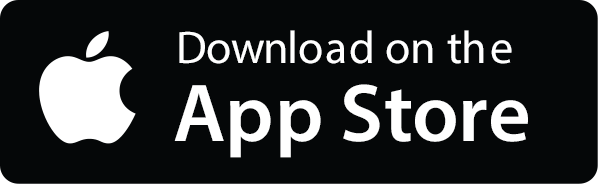
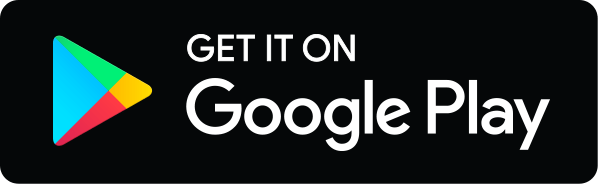
