Principles of Vascular Access
Vascular catheters are ubiquitous to cardiothoracic critical care. They may be defined or described on the basis of numerous factors (Table 11.1). For the purposes of this chapter, vascular access relates to catheters within the arterial or central venous system.
Table 11.1 Factors defining vascular catheters
Type of vessel: arterial, venous; peripheral, central |
Site of insertion: jugular, femoral, central, etc. |
Duration of access: short term, medium term, long term |
Pathway from skin to vessel: tunnelled, non-tunnelled |
Length: short, mid, long |
Additional features: number of lumens, antibiotic, antiseptic or heparin impregnated |
Insertion
Vascular catheter insertion within the critical care unit is almost universally by the Seldinger technique: a needle is inserted into the target vessel (or a tributary of the target vessel); a guide wire is passed into the vessel; one or more dilators are passed over the guide wire to create a tract through the skin and soft tissues; and the catheter is advanced over the guide wire, into the vessel.
Traditionally, the target vessel was sought using a combination of anatomical landmarks and palpation. The increasing availability of point-of-care ultrasound technology within the critical care environment has led to a gradual move towards real-time ultrasound guided vascular access. The use of ultrasound for vascular access has been demonstrated to increase success and reduce complications and is now considered a standard of care by the National Institute of Clinical Excellence.
Position may be confirmed by ensuring free aspiration of blood, by transduction of pressure (thereby confirming arterial or venous placement), by measurement of the oxygen content of aspirated blood (suggestive of arterial or venous placement) or by imaging (either X-ray or ultrasound).
Complications
The complications associated with line placement, and potential strategies to minimise risk are listed in Table 11.2.
Table 11.2 Complications related to central venous catheter placement and strategies to reduce risk
Immediate | Damage to adjacent structure (e.g. arterial puncture, pneumothorax) | Ultrasound guidance, experienced operator (particularly in the presence of anatomical abnormalities) |
Air embolism | Head-down position in jugular and subclavian approaches | |
Bleeding | Correction of coagulopathy prior to insertion | |
Late | Infection | Introduction of CVC insertion ‘bundles’, full barrier precautions during insertion, use of antibiotic impregnated catheters, use of antimicrobial impregnated patches over the insertion site, avoidance of the femoral vein as an insertion site, removal of catheters when no longer required |
Thrombosis | Avoidance of femoral insertion, use of heparin impregnated catheters, early removal |
Pressure Monitoring
Transducers
A transducer converts mechanical energy (hydrostatic pressure within the vascular system) into an electrical signal; the hydrostatic pressure within the catheter is quantified and displayed as both a value and a waveform.
A continuous column of fluid maintains contact between the transducer and the vascular system. Changes in pressure are transmitted down the column of fluid, leading to distortion of the transducer membrane. The membrane contains a strain gauge: distortion of the membrane stretches a wire, altering its resistance. The wire is integrated into a Wheatstone bridge, which allows accurate determination of changes in resistance. The change in resistance equates to change in pressure and this is displayed on the monitor.
A valve within the transducer system permits flow of 3–4 ml of saline per hour through the arterial line, to maintain line patency. Some units heparinise the saline flush on the basis that this may reduce the risk of intracatheter thrombus formation. The use of heparin, however, exposes the patient to heparin (and associated reactions) and may interfere with the accuracy of coagulation samples drawn from the line if the flush fluid is incompletely removed prior to sampling. Systematic review of studies examining the efficacy of heparinised saline in this context found no convincing evidence of improved catheter patency.
Resonance and Damping
Every material has a natural frequency: the frequency at which it freely resonates. If a material is exposed to a frequency close to its natural frequency it will resonate, or oscillate, at its maximum amplitude. This physical phenomenon is of significance to pressure monitoring systems as, if the frequency of one of the components of the measured pressure wave is close to the natural frequency, the excessive oscillation generated will distort the measured pressure.
In order to avoid this phenomenon, the natural frequency of a pressure monitoring system must be several orders of magnitude greater than the component frequencies of the waveform being measured. Using longer tubing, of wider diameter and using a low density fluid within the tubing, will increase the natural frequency of a system.
Damping describes the dissipation of energy in a resonant, oscillating system. A pressure monitoring system may be over-damped by tubing, which is of excessive length, insufficient diameter, or made from highly compliant material. Additionally, kinks, clots or air bubbles within the system will contribute to damping.
The optimal system strikes a balance between resonance and damping. A system with excessive resonance will tend to over-measure peak (systolic) pressures and under-measure trough (diastolic) pressures; mean pressure should be unaffected. Such systems are described as under-damped. In contrast, a system with excessive damping and insufficient resonance (damped system) will under-measure peak and over-measure trough pressures; again mean pressure is unaffected. Whilst pressure measurement systems are produced with material and dimensions most suited to optimal damping, over-damping and under-damping may occur.
Zeroing
Accurate measurement of physiological pressure requires the transducer to be ‘zeroed’ to atmospheric pressure (a system pressure of zero equates to atmospheric pressure, as physiological pressures are described as relative to atmospheric pressure). Calibration involves opening the pressure system to air, and referencing this pressure to zero on the system. Transducers are prone to baseline drift and therefore this process should be undertaken several times per day.
The height of the transducer relative to the patient is also important. By convention, the transducer should be at the same height above the floor as the left atrium of the patient. A transducer placed above or below the height of the atrium will lead to under-reading and over-reading of pressure respectively.
Arterial Catheters
There are three major indications for placement of an arterial catheter.
1. Haemodynamic instability (either current or expected) in which beat to beat measurement of arterial pressure allows close monitoring of haemodynamic state and safe titration of inotropic and vasoactive drugs.
2. Need for frequent arterial blood sampling (e.g. respiratory failure or severe, persistent metabolic disturbance).
3. Non-pulsatile flow (for example in cardiac bypass or venoarterial ECMO) as there is no alternative method of measuring systemic pressures.
Arterial catheters may be inserted into:
Peripheral arteries (e.g. most commonly radial, also ulnar, brachial, dorsalis pedis);
Central arteries (e.g. most commonly femoral, also axillary).
The arterial pressure in peripheral arteries differs from that in central arteries.
A decrease in arterial compliance with increasing distance from the heart leads to a higher systolic and lower diastolic pressure measurement in peripheral compared with central arteries; the mean pressure should be comparable.
The tone of peripheral arteries will demonstrate a more significant response to temperature and vasoactive medications than central arteries; measurement of peripheral arterial pressure could therefore be a poor reflection of the central arterial pressure perfusing vital organs.
These differences between central and peripheral pressure are more pronounced in the elderly and in those with vascular disease.
Information Derived from the Arterial Waveform
Pulse rate
Systolic blood pressure
Diastolic blood pressure
Mean blood pressure
The shape of the arterial waveform is determined by numerous factors:
○ The stroke volume,
○ Left ventricular contractility,
○ Capacitance of the central arterial tree (the ability to distend and accommodate ejected blood),
○ Peripheral resistance (the rate at which blood dissipates from the central arteries into peripheral circulation).
The dichrotic notch is the positive deflection in the downstroke of the arterial waveform, which represents closure of the aortic valve:
○ Typically occurs at one third into the pressure wave descent when aortic pressure (AoP) exceeds left ventricular pressure (LVP);
○ If peripheral resistance is reduced (e.g. sepsis), AoP > LVP later in the cycle and the dicrotic notch shifts down the curve.
Pulse Pressure Variation
The intrathoracic pressure varies throughout the respiratory cycle; this alters the loading conditions of the heart and results in variation in stroke volume and pulse pressure between inspiration and expiration.
The magnitude of this variation is greater on the steep portion of the Frank–Starling curve (where a patient is likely to increase cardiac output in response to fluid) than on the flat portion (with less likelihood of fluid responsiveness).
Meta-analysis of studies relating to pulse pressure variation (PPV) (and the closely related stroke volume variation) suggests that variation of >13% is strongly predictive of fluid responsiveness.
The studies relating to PPV were however largely conducted in highly controlled environments, in deeply sedated patients, with no spontaneous ventilation; analysis of the intensive care population shows that few patients are comparable to the subjects of the initial studies; the practical application of PPV in the clinical setting therefore has significant limitations.
Central Venous Catheters
A central venous catheter (CVC) is a vascular catheter with a tip sitting in the central venous system. These may be short catheters (15–20 cm) inserted into a central vein (femoral, jugular or subclavian) or longer catheters inserted into a peripheral vein and advanced to the central circulation (peripherally inserted central catheter, PICC).
Indications for CVC placement include the following.
Administration of drugs which may only be safely delivered directly into a central vein (e.g. amiodarone, noradrenaline, concentrated potassium, cytotoxic agents, total parenteral nutrition).
Measurement of central venous pressure (CVP) or central venous oxygen saturation (ScvO2).
To facilitate a procedure which requires access to the central venous circulation (e.g. renal replacement therapy, pulmonary artery catheter flotation, temporary pacing wire insertion, extracorporeal membrane oxygenation).
If peripheral venous access proves impossible.
Central Venous Pressure Trace
The central venous pressure (CVP) trace is outlined in Figure 11.1.
Figure 11.1 CVP trace: a atrial contraction; c closure of tricuspid valve; x atrial relaxation; v ventricular contraction; y opening of the tricuspid valve.
Central Pressures
The CVP, when measured in the superior vena cava (SVC) equates to right atrial pressure (RAP). The significance of CVP in terms of the global haemodynamic state is complex and its role in management of the haemodynamic state is controversial.
At the end of the nineteenth century, Otto Frank and Ernest Starling described the relationship between myocardial stretch and stroke volume and demonstrated an increase in cardiac output in response to increasing preload. This so-called Frank–Starling mechanism became a cornerstone of cardiovascular physiology and remains an important concept in haemodynamic management.
Some 50 years after this original work, Arthur Guyton built upon the Frank–Starling concept. Guyton concluded, on the basis of a series of animal models, that venous return (Vr) was the key determinant of cardiac output. According to the Guyton model, Vr is determined by three factors: the mean circulatory filling pressure (MCFP) (generated by the elastic properties of the vasculature and the hydrostatic force exerted by volume within the vessels, the so-called stressed volume); RAP (against which the MCFP must drive); and the venous resistance (which may be altered by changes in regional blood flow to organ vascular beds). The accuracy of this complex model remains the subject of significant debate, not least because it is impossible to test in an intact human circulation. It remains therefore primarily a theoretical construct.
One key practical product of Guyton’s work has however arisen: the use of the RAP (in the form of CVP) in the clinical optimisation of cardiac output. Guyton considered RAP to be an independent determinant of SV (and therefore cardiac output). He proposed RAP and SV to have a similar relationship to that between end-diastolic volume and SV reported by Starling. Given its ease of measurement, CVP was thus widely integrated into clinical practice as a measure of left ventricular preload.
The intervening six decades have witnessed significant technological advances in terms of haemodynamic monitoring. Yet the use of CVP as a marker of left ventricular preload persists. The Surviving Sepsis Campaign, for example, recommends a CVP of 8–12 mmHg as a target for volume resuscitation in the shocked septic patient.
The clinical utility of CVP in the assessment of preload is however questionable. Manipulation of preload in the shocked patient is undertaken with a view to optimising cardiac output; any assessment of preload is therefore beneficial only if it reliably identifies those patients in whom increase in preload (by fluid administration) is likely to increase cardiac output. In clinical trials, the CVP has consistently been shown to be a poor determinant of fluid responsiveness. As such, there are calls from some quarters for the CVP as a marker of preload to be abandoned.
Pulmonary Artery Catheter
History
The pulmonary artery catheter (PAC) evolved during the twentieth century as a means of measuring pressure and flow within the pulmonary vasculature. Ronald Bradley of St Thomas’ Hospital, London, first described the placement of a catheter in the pulmonary circulation of humans in the 1950s. This original catheter was, however, difficult to site. Harold Swan (an alumnus of St Thomas’ Medical School) and his colleague William Ganz (Cedars-Sinai Medical Center, Los Angeles) adapted Bradley’s design by adding a flotation balloon, thereby enhancing ease of insertion. The names of Swan and Ganz have since become eponymous with the PAC.
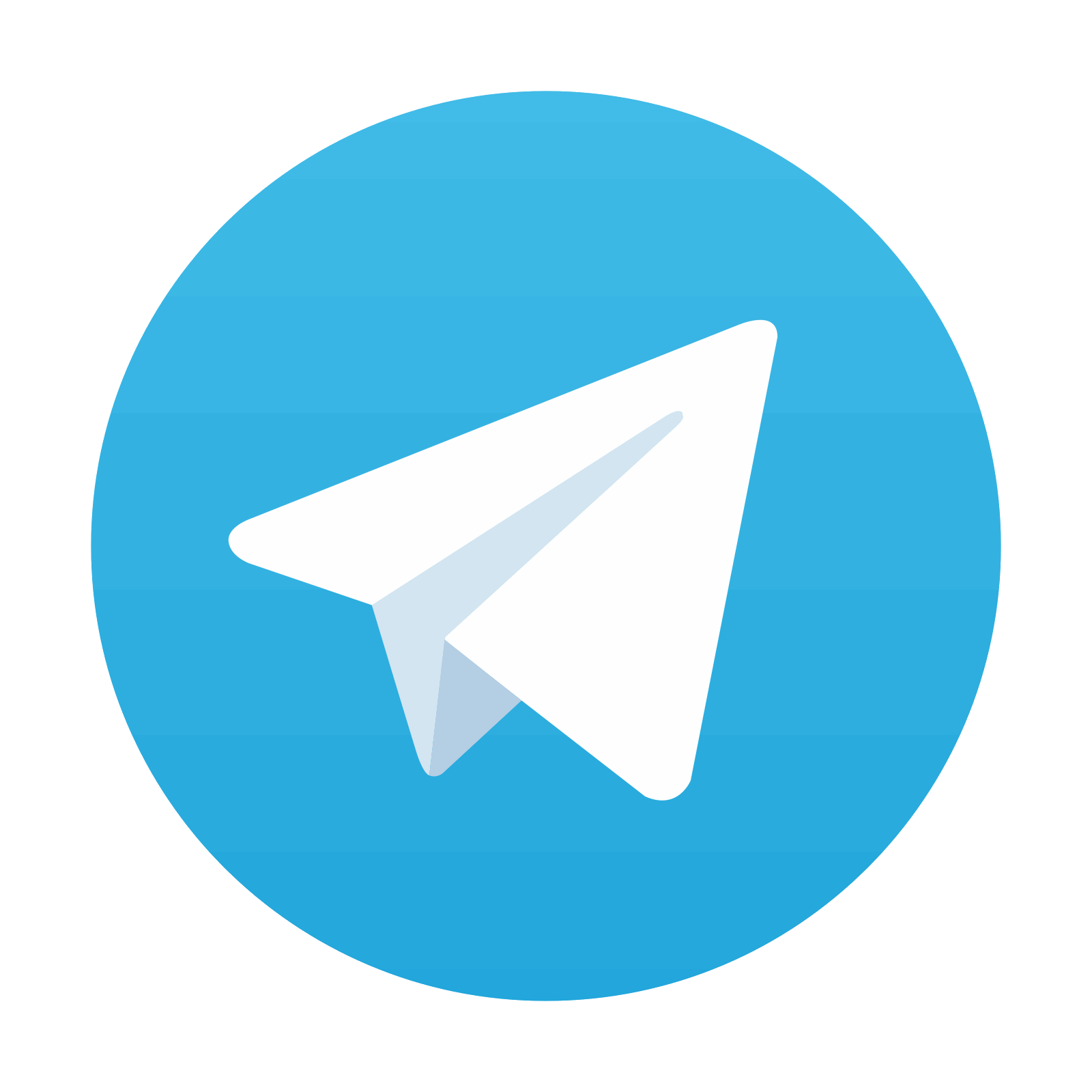
Stay updated, free articles. Join our Telegram channel

Full access? Get Clinical Tree
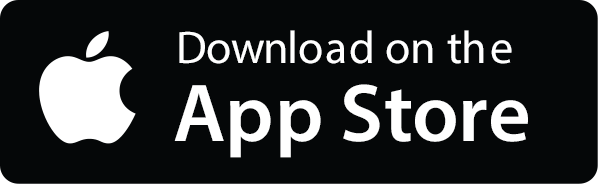
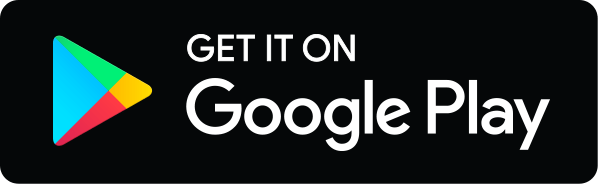
