Chapter 58 Lung, Chest Wall, Pleura, and Mediastinum
Anatomy
The bony thorax is covered by three groups of muscles—the primary and secondary muscles for respiration and those attaching the upper extremity to the body (Fig. 58-1). The primary muscles include the diaphragm and intercostal muscles. The intercostal muscles of the intercostal spaces include the external, internal, and transverse or innermost muscles. Eleven intercostal spaces, each associated numerically with the rib superior to it, contain the intercostal bundles (vein, artery, and nerve) that travel along the lower edge of each rib. All intercostal spaces are wider anteriorly and each intercostal bundle falls away from the rib posteriorly to become more centrally located within each space. The intercostal muscle layers assist with respiration and protect the thoracic structures. The extrinsic muscles of the chest, latissimus dorsi muscle, serratus anterior muscle, pectoralis major and minor muscles, and cervical muscles (sternocleidomastoid, scalene muscles) attach to the bony thorax, protect the chest wall itself, and may assist with ventilatory efforts in those with chronic obstructive pulmonary disease (COPD).
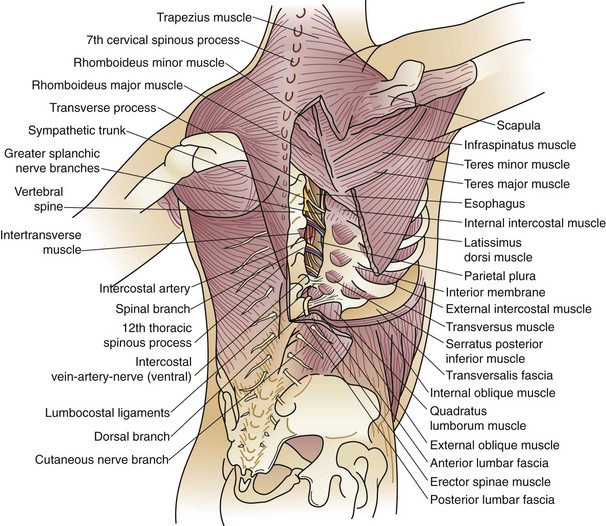
FIGURE 58-1 Musculature of the chest wall.
(From Ravitch MM, Steichen FM: Atlas of General Thoracic Surgery. Philadelphia, WB Saunders, 1988.)
The bony thorax consists of 12 ribs peripherally extending from the vertebrae posteromedially, to the sternum or costal arch anteriorly (Fig. 58-2). The 11th and 12th ribs are floating ribs and are not attached directly to the sternum. Ribs 1 to 5 are directly attached to the sternum by costal cartilages. The lower ribs (6 to 10) coalesce into the costal arch. The first rib is relatively flat, dense, and travels from the first thoracic vertebra to the manubrium to create the thoracic inlet (Fig. 58-3). Through this relatively small area pass the great vessels, trachea, esophagus, and innervation to the upper extremity, diaphragm, and larynx. Trauma to this area, manifested by a first rib fracture, is the consequence of a significant mechanical force with likelihood of injury to one or more of these structures. Other structures within the thoracic inlet include the phrenic nerve, recurrent laryngeal nerve in the tracheoesophageal groove, which recurs around the aorta at the ligamentum arteriosum on the left and around the innominate artery on the right, and insertion of the thoracic duct posteriorly at the junction of the left subclavian with the left internal jugular veins. The remaining ribs gradually slope downward. Each rib is composed of a head, neck, and shaft. Each head has an upper facet, which articulates with the vertebral body above it, and a lower facet, which articulates with the corresponding thoracic vertebra to that rib, establishing the costovertebral joint. The neck of the rib has a tubercle with an articular facet; this articulates with the transverse process, creating the costotransverse joint and imparting strength to the posterior rib cage.
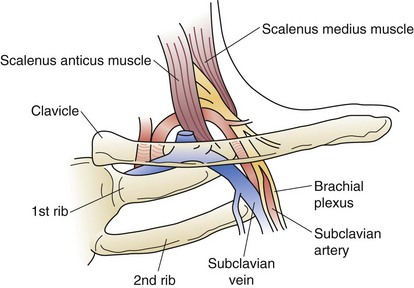
FIGURE 58-3 Relationship of the neurovascular bundle to the scalenus muscles, clavicle, and first rib.
(From Urschel HC: Thoracic outlet syndromes. In Baue AE, Geha AS, Hammond GL, et al [eds]: Glenn’s Thoracic and Cardiovascular Surgery, ed 6, Stamford, CT, Appleton & Lange, 1996, p 567.)
The lungs are broadly divided into five lobes, with multiple segments in each lobe (Fig. 58-4). The right lung is composed of three lobes, the upper, middle, and lower. Two fissures separate these lobes. The major, or oblique, fissure separates the lower lobe from the upper and middle lobes. The minor or horizontal fissure separates the upper lobe from the middle lobe. The left lung has two lobes—the upper lobe and lower lobe. The lingula corresponds embryologically to the right middle lobe. A single oblique fissure separates the lobes.
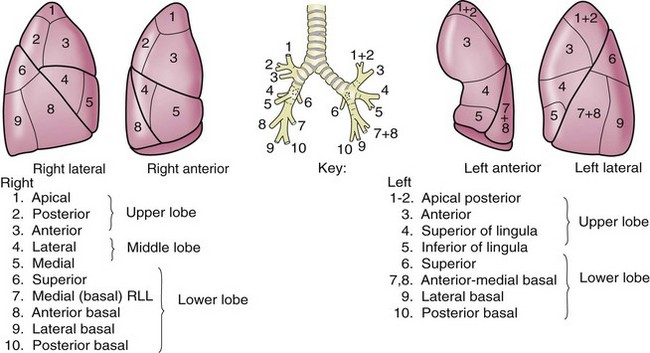
FIGURE 58-4 Segments of the pulmonary lobes.
(Adapted from Jackson CL, Huber JF: Correlated applied anatomy of the bronchial tree and lungs with a system of nomenclature. Dis Chest 9:319, 1943.)
Selection Of Patients For Thoracic Operations
The physiologic evaluation of the thoracic surgical patient must be individualized for each patient, but generally emphasizes pulmonary and cardiac function. The assessment of a patient’s ability to tolerate lung resection from a cardiopulmonary standpoint is fundamental to patient selection for surgery. Patients with advanced pulmonary disease and severe pulmonary dysfunction may have a prohibitive risk, which may exist in more than one third of patients with otherwise resectable lung disease.1
Cigarette smoking is associated with up to a sixfold increase in the incidence of postoperative pulmonary complications after surgery.2 If the patient is a smoker, he or she must stop smoking immediately. The physician must clearly communicate this message. Although there are few studies specific to pulmonary resection, there is evidence that preoperative smoking abstinence of 4 to 8 weeks’ duration is necessary to reduce the incidence of complications. Ideally, patients are smoke-free for a minimum of 2 weeks and preferably 4 to 8 weeks before surgery,3 although smoking cessation at any time is valuable.4 Smoking cessation programs may be helpful for these patients, and they may need pharmacologic assistance. This combination may have increased efficacy in smoking cessation efforts over counseling alone.
Physiologic Evaluation
Before thoracic operations, patients may be evaluated by a combination of physiologic studies.5 A plain chest roentgenogram is commonly obtained (Fig. 58-5). Spirometry measures the lung volumes (Fig. 58-6) and mechanical properties of lung elasticity, recoil, and compliance. Pulmonary function testing (Fig. 58-7) also evaluates gas exchange functions, such as DLCO (diffusion of carbon monoxide in the lung).
Quantitative radionucleotide perfusion scanning involves the injection of 99mTc-radiolabeled albumin particles followed by the visual inspection of planar images (Fig. 58-8). Quantitative perfusion provides a measurement of the relative function of each lobe and lung, allowing a prediction of pulmonary function after lung resection:
The carbon monoxide diffusing capacity (DLCO) can be measured by several methods, although the single-breath test is most commonly performed. The DLCO measures the rate at which test molecules such as carbon monoxide move from the alveolar space to combine with hemoglobin in the red blood cells. The DLCO is determined by calculating the difference between inspired and expired samples of gas. DLCO levels less than 40% to 50% are associated with increased perioperative risk.6
Cardiopulmonary Exercise Testing
A patient’s risk of perioperative morbidity and mortality may be stratified by . Those with
above 20 mL/kg/min are not at increased risk for complications or death after resection of non–small cell lung cancer (NSCLC). A level below 15 mL/kg/min is associated with an increased risk, and
less than 10 mL/kg/min indicates very high risk, generally precluding operation.7,8 Some have advocated stair climbing as a suitable measure of preoperative cardiopulmonary assessment.9 Given the wide availability of more objective and standardized noninvasive tests for cardiopulmonary function, stair climbing performance should not be used as the sole criterion to determine physiologic suitability for lung cancer resection. In patients undergoing evaluation for lung volume reduction surgery or for lung transplantation, a 6-minute walk test is used for a measure of the cardiac and pulmonary reserve. Patients are told to walk as far and as fast as they can during this time period. Distances of more than 1000 feet suggest an uncomplicated course.
Lung
Congenital Lesions
Various congenital lung abnormalities can occur as a consequence of disturbed embryogenesis.10 Bilateral agenesis of the lungs is fatal. Unilateral agenesis may occur more frequently on the left (≈70%) than on the right (≈30%), with more than a 2 : 1 male-to-female ratio.
Cystic Lesions
A bronchogenic cyst arises from a tracheal or bronchial diverticulum (see later, “Mediastinal Cysts and Tumors”). This diverticulum becomes completely separated from the trachea and is frequently found as an asymptomatic mass on routine chest x-rays. Computed tomography (CT) of the chest demonstrates this abnormality as a homogeneous-type mass, well circumscribed, and adjacent to the trachea (Fig. 58-9). The bronchogenic cyst accounts for 10% of mediastinal masses in children and is located in the midmediastinum. Treatment consists of excision, even if the patient is asymptomatic, to confirm the diagnosis.
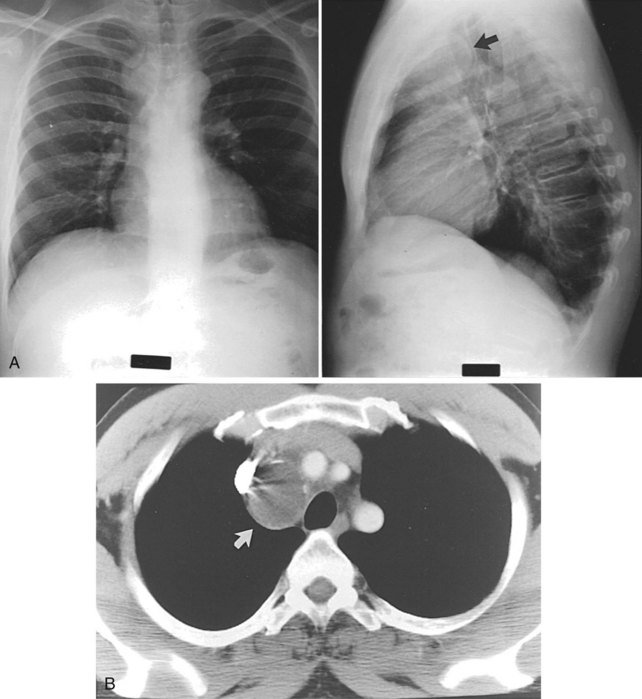
FIGURE 58-9 Two chest roentgenograms (A) and a CT scan (B) of the chest of a patient with a bronchogenic cyst (arrow).
Congenital Bronchopulmonary Malformations
Lobar emphysema10 is the most commonly resected congenital cystic lesion (50%). The onset of rapidly progressive respiratory distress usually occurs from 4 to 5 days to several weeks after birth. It rarely occurs after 6 months of age. It predominantly affects the upper lobe. Bronchiolitis is probably the most common cause overall. Treatment is lobectomy.
Congenital Abnormalities of the Trachea and Bronchi
Esophageal atresia with tracheoesophageal fistula is the most frequent abnormality of the trachea in infants (see later, “Trachea”) Bronchial atresia is the second most frequent congenital pulmonary lesion after tracheoesophageal fistula.11 The lung tissue distal to the atresia expands and becomes emphysematous as a result of air entry through the pores of Kohn. With no exit for air or mucus because of this blind bronchial stump, emphysema from air trapping or development of a mucocele may develop. Chest x-rays may demonstrate hyperinflation of a lobe or segment. The oval density may be identified between the hyperinflated lung and hilum. The left upper lobe is the most frequently involved of all lobes within the lung. Diagnosis may be confirmed with bronchography or CT. The surgeon must rule out a mucous plug, adenoma, vascular compression, and sequestration.
Congenital Vascular Disorders
Congenital vascular disorders of the lungs may occur.12 In Swyer-James-Macleod syndrome, there is an idiopathic hyperlucent lung. This problem develops from chronic pulmonary infections such as bronchiectasis. As the consolidation persists, decreased pulmonary artery blood supply may cause a so-called autopneumonectomy and hyperlucent lung.
Vascular rings13 represent 7% of all congenital heart problems.14 The most common vascular ring is a double aortic arch, which occurs in 60% of all cases. The right, or posterior, arch is the larger and gives rise to the right carotid and right subclavian arteries. The ring wraps around the trachea and esophagus. A posterior indentation is noted in the esophagus on barium swallow. Simple division corrects the anomaly. A right aortic arch with retroesophageal left subclavian artery and left ligamentum arteriosum occurs in approximately 25% to 30% of patients with vascular rings. Intracardiac defects occur with double aortic arch. Most of these infants require operation within the first weeks or months of life.
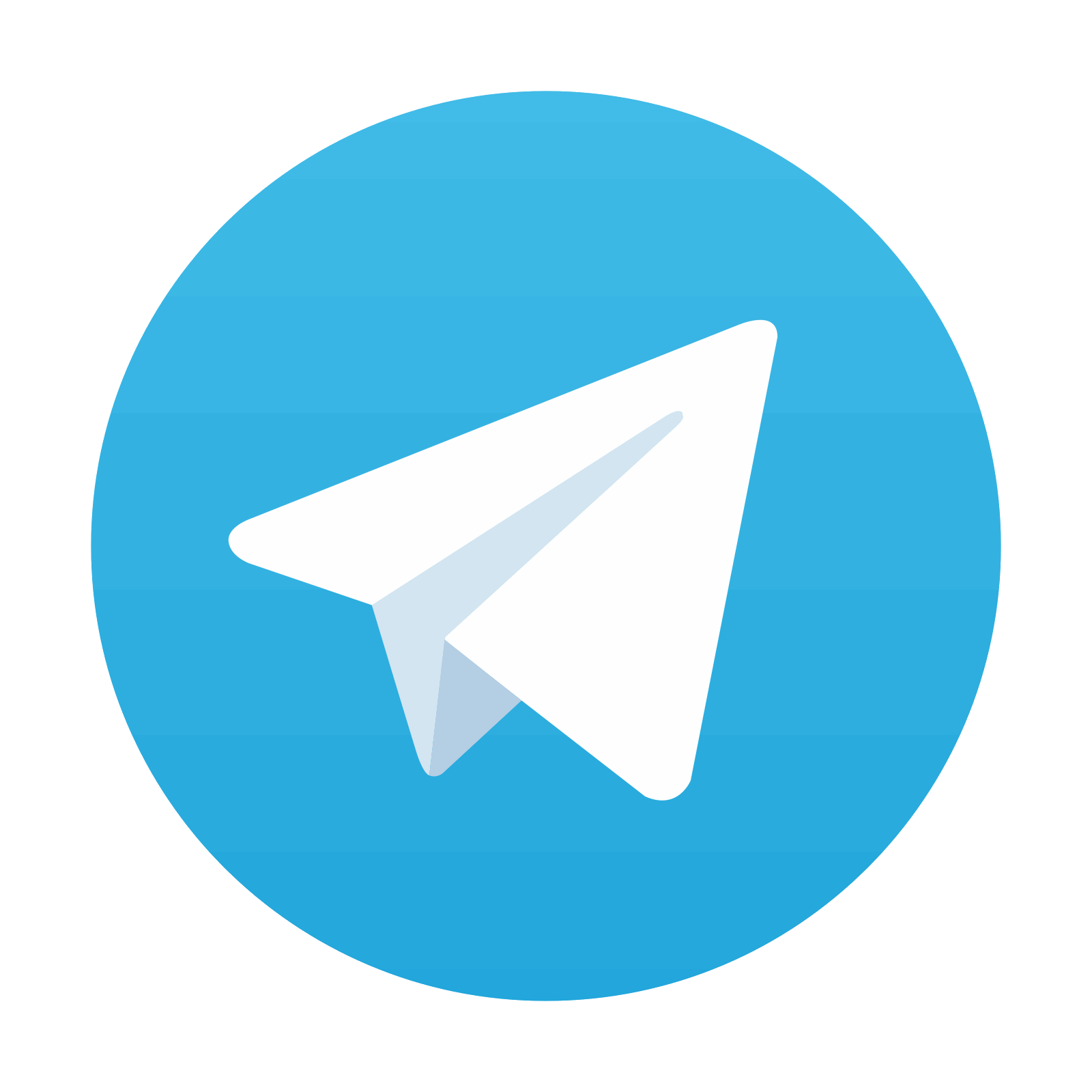
Stay updated, free articles. Join our Telegram channel

Full access? Get Clinical Tree
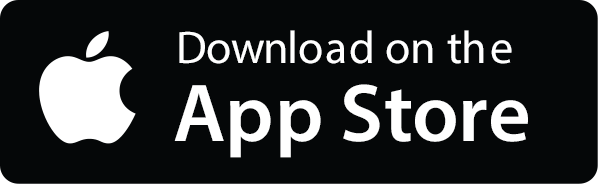
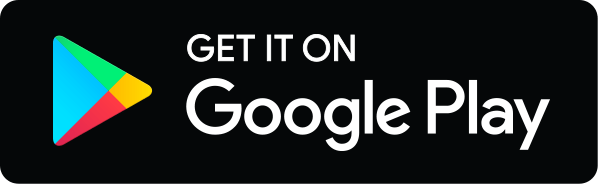