In this chapter we will discuss the elements of chest CT scanning protocols that are optimized for lung CT AI applications. These lung CT AI applications can be divided into visual CT (VCT) applications, where the analysis of the lung CT images is done by an expert imaging physician, and quantitative CT (QCT) applications, where the image analysis is done by AI. The focus of this book is on QCT AI applications, so we will focus primarily on the elements of QCT scanning protocols and how the elements of these protocols were developed over time. The technology requirements for QCT of the lung go beyond those that are necessary for VCT of the lung, though there are many elements of a VCT scanning protocol that are also common in a QCT scanning protocol.
Visual and quantitative chest CT scanning protocols need to address ionizing radiation dose using the ALARA principle (see Chapter 2 ). The radiation dose needs to be kept as low as possible while providing the CT image quality necessary to achieve the goals of the CT study. The goals of a VCT study may not need as demanding a CT scanning protocol as a QCT study. It is important to not administer more ionizing radiation dose than is necessary to achieve the objectives of the study and to keep in mind that the effective dose of chest CT studies should be in the range of 1 to 10 mSv, depending on the application. Low-dose CT studies are defined as those that have an effective dose less than 1 mSv. Low-dose studies should be done where the objectives of the study can be obtained with a low-dose CT scanning protocol.
Additional elements of visual and quantitative chest CT scanning protocols include x-ray tube peak voltage (kVp) and tube current time product (mAs), as well as x-ray tube current and voltage modulation, x-ray beam collimation and additional filtration, pitch, positioning the patient at the isocenter of the CT gantry, total time for the examination, supine versus prone positioning of the patient on the CT scanner table, lung volume used for the scan, and making sure the patient is coached to the selected lung volume, SFOV, DFOV, reconstruction method (FBP vs IR), and reconstruction kernel ( Box 3.1 ). It is important to have a quality control (QC) program for both VCT and QCT that includes the use of CT phantoms, which are inanimate objects that simulate various material densities and structures contained in the human thorax to routinely determine if the CT scanner is working properly. There is also the need to decide how long the projection data is kept on the scanner so additional CT image reconstructions can be done if there is something that was done incorrectly in the image reconstruction process. The transfer of CT data to different sites via intranets and internets needs to be worked out, too.
- 1.
Radiation dose adheres to “As Low As Reasonably Achievable” (ALARA) Principle
- 2.
Routine quality control procedures
- a.
X-ray CT quality control phantoms
- b.
X-ray CT projection data retained for short period of time (e.g., 48 hours)
- a.
- 3.
X-ray tube peak voltage (kV)
- 4.
X-ray tube current time product (mAs)
- 5.
X-ray tube focal spot, filters, and collimation
- 6.
X-ray tube current modulation
- 7.
X-ray tube rotation time, CT table velocity and resultant pitch
- 8.
Patient positioning
- a.
Isocenter
- b.
Supine versus prone
- a.
- 9.
Scan field of view (SFOV), display field of view (DFOV)
- a.
Size of reconstruction matrix (e.g., 512 × 512, 1024 × 1024)
- a.
- 10.
Breathing the patient to the proper lung volume
- a.
Total lung capacity (TLC)–deep inspiration
- b.
Functional residual capacity (FRC)–normal passive expiration
- c.
Residual volume (RV)–deep expiration
- a.
- 11.
CT reconstruction method
- a.
Reconstruction kernel
- b.
Filtered back projection (FBP)
- c.
Iterative reconstruction (IR)
- d.
Deep machine learning reconstruction
- a.
- 12.
Axial CT image reconstruction width and interval
- 13.
Transmission, storage, and display of CT images
The quantitative CT scanning protocols that drive lung CT AI have been developed to try and address the issue of generating the most precise and accurate voxel size and CT numbers (HU) assigned to each voxel within the CT lung images, and to maintain the precision and accuracy of the CT voxels contained in the lung CT images over time and on different CT scanner make and models. We will review how the current quantitative CT scanning protocols were developed.
Early Work in the Development of QCT Scanning Protocols
Dirksen et al. reported in 1999 that quantitative CT using the 15th percentile point of the lung density histogram showed decreased progression of alpha-1 antitrypsin deficiency (A1AD) induced emphysema in A1AD patients who were receiving monthly intravenous augmentation therapy with human alpha-AT. The quantitative CT results were much more sensitive than monitoring decreases in airflow measured by using the forced expiratory volume in one second, FEV1. This was a turning point in the development of lung CT AI using quantitative CT metrics, such as the 15th percentile method, because now quantitative lung CT was doing something noninvasively that visual lung CT and pulmonary physiological measures of lung function could not do.
Workshop: Quantitative Computed Tomography Scanning in Longitudinal Studies of Emphysema
The excitement around Dirksen’s paper in 1999 led to the Alpha-1 Foundation organizing a workshop entitled “Quantitative Computed Tomography Scanning in Longitudinal Studies of Emphysema” that took place February 2 and 3, 2001. The workshop brought together experts in thoracic radiology, pulmonology, and lung pathology to explore current state-of-the-art CT imaging used to quantitate pulmonary emphysema, and to discuss the feasibility of using quantitative lung CT image data as a primary endpoint in drug trials designed to assess new treatments for A1AD. The primary goal of the workshop was to develop a consensus on the optimal parameters to be included in a quantitative CT scanning protocol that would be recommended for randomized, double-blind, multicenter drug trials for the treatment of A1AD.
Can X-ray CT Detect and Quantify Pulmonary Emphysema?
Pulmonary emphysema is defined as “abnormal permanent enlargement of air spaces distal to the terminal bronchioles, accompanied by destruction of their walls and without obvious fibrosis.” The workshop pointed out that the increase in the size of the airspace of the lung alveoli and the decrease in tissue mass due to destruction of the alveolar walls would cause a decrease in tissue density, g/mL. As was pointed out in Chapter 2 , the CT numbers assigned to the individual voxels of the lung CT images correspond closely to the density of lung tissue. Therefore, CT scanning should be able to assess the presence and extent of pulmonary emphysema both visually and quantitatively.
Single Versus Multiple Detector Row CT Scanners
At the time of the workshop, multiple-detector spiral CT scanners (MDCT), as described in Chapter 2 , were increasing in number and had advantages over the single-detector spiral CT (SDCT) scanners that preceded them. It was pointed out that the MDCT scanners were preferable to SDCT scanners in the QCT assessment of emphysema. This is because the greater the number of detector rows in the z-axis of the patient, the faster the entire lungs could be scanned. This is especially important in patients that are short of breath due to their underlying emphysema. Four-, 8-, and 16-row MDCT scanners with a detector width of 1.0 to 1.25 mm could obtain high-resolution images of the entire lungs in 25 to 30 seconds, 10 seconds, and 5 seconds, respectively. Sixteen-row MDCT scanners would be preferable, if available.
It was also recognized that for longitudinal studies, the same CT scanner model should be used at the different study time points for a given patient throughout the course of the study. If different CT scanner models were going to be used in a study for the same study patient, CT phantoms need to be used to verify that the different CT scanner models had equivalent spatial and density resolution.
Constant and Optimal X-ray Tube Peak Kilovoltage, mAs, and Radiation Dose
The x-ray tube peak voltage and x-ray tube current time product were discussed in the workshop and, given the MDCT technology at the time, it was agreed that the peak x-ray tube voltage should be between 120 and 140 kV and the same x-ray tube peak voltage should be used for all subjects in a given study. This is because the x-ray tube peak voltage determines the x-ray photon beam spectrum coming from the anode of the x-ray tube. Additional x-ray beam shaping and filtration placed in front of the x-ray tube, but before the patient, were not specifically addressed. The majority of the workshop participants favored setting the tube current time product between 80 and 100 mAs to provide acceptable image-to-noise ratio in the lung, while greater values would increase the ionizing radiation dose to the subject/patient without realizing any additional benefit. Twenty mAs was suggested as the minimum possible for a particular CT scanner model and CT scanning protocol. The recommended dose should follow the ALARA principle, achieving sufficient image quality to assess lung density accurately but no higher, with the effective dose range between 1 and 10 mSv. The 20 mAs study had an effective dose of 0.7 mSv. Phantom studies should be conducted to verify that the kV and mAs for the study would provide sufficient image quality for the chosen quantitative CT metric and keep the effective dose no higher than 10 mSv.
Scan Mode and Pitch
The optimal scan mode was spiral CT scanning, not axial scanning. The pitch settings were discussed and pitch values between 1.0 and 2.0 were acceptable. Pitch values greater than 2.0 were not acceptable because of adverse effects on image quality. Pitch values less than 1.0 increased radiation dose and were not recommended.
Detector Width and Recommended Axial Image Thickness and Spacing
The workshop recommended using an effective detector element z axis thickness of 1 to 1.25 mm, with the ability to reconstruct thin 1- to 1.25-mm axial images and thicker axial images with a slice thickness equal to or greater than 7 mm. The thinner slice thickness would be able to detect small foci of emphysema with greater image noise, and the thicker slices would have less noise but would also average out small foci of emphysema that were much smaller than 7 mm. The 16-row MDCT scanners would be able to accomplish this with scan times less than 12 seconds. The focus of the workshop was on determining abnormally low attenuating areas of lung density (see Chapter 5 ) as a measure of pulmonary emphysema. At the time of this workshop, the assessment of airway geometry, which requires slice thicknesses less than 1.5 mm, and submillimeter is better, was not a consideration. It was recognized that if lung texture was to be assessed, then the detector element thickness would need to be 1.25 mm or less.
Image Reconstruction
At the time of this workshop, filtered back projection (FBP) was the only reconstruction method available for image reconstruction. Iterative reconstruction (IR) was still in the future for commercial clinical x-ray CT scanners. However, it was recognized that the reconstruction kernel used in FBP image reconstruction was very important in determining both image noise and image spatial resolution. It was recommended that a neutral kernel be used, which at the time for the GE CT scanners was the “Standard” FBP reconstruction kernel. This neutral kernel would not edge enhance or smooth the image. It is interesting to note that this recommendation for using a neutral kernel in FBP is still favored in the early 2020 s for lung CT AI.
Optimal Lung Volume—Total Lung Capacity (TLC)
There are two main issues related to lung volume and the scanning of the patient for the assessment of lung disease: lung volume and coaching versus spirometric gating. It was generally agreed that for the assessment of emphysema the recommended lung volume is TLC. It was recommended that careful coaching of the patient to TLC by the x-ray CT technologist was the best approach. The use of spirometric gating was not recommended, as it did not add sufficient value over careful coaching of the patient to a given lung volume (e.g., TLC, RV). It should be noted that careful coaching is the approach in patients who undergo pulmonary physiology testing to assess airflow and lung volumes.
Administration of Intravenous Iodinated X-ray CT Contrast Media
The administration of intravenous iodinated contrast material will increase the x-ray CT measures of lung density due to the photoelectric absorption of x-ray photons by the iodine atoms in the contrast material. The presence of the iodinated contrast in both the intravascular and extravascular extracellular compartments of the lung tissue increases the x-ray CT measures of lung tissue density in HU due to the presence of the iodine atoms, and not due to any real change in the lung tissue density in the absence of the contrast material. So it was recommended that the use of iodinated contrast material should not be used in studies to assess pulmonary emphysema using lung density measurements from x-ray CT.
X-ray CT Phantoms for Image Quality Assessments
It was recommended that air calibration CT scans and CT scans of water phantoms should be used to calibrate the CT scanners daily to maintain the accuracy of the CT HU numbers. Anthropomorphic x-ray CT phantoms can also be used to assess the accuracy of the CT scanners assessments of the density of normal and emphysematous lung tissue. The use of dedicated x-ray lung CT phantoms is important in assessing CT scanner performance and maintaining CT image quality.
CT Image Analysis
The 15th percentile was the quantitative CT metric recommended by this workshop to assess changes in lung density due to pulmonary emphysema (see Chapter 5 ).
Image Data Transfer, Analysis, and Storage
It was recommended that both the reconstructed lung CT images and the unreconstructed projection data be transferred to a central image analysis facility using either DVD media or direct image transfer over the internet. The unreconstructed projection data are very large files, much larger than the reconstructed CT images; are difficult to obtain from many commercial CT scanner manufacturers; and present difficult challenges for transfer using DVDs or direct internet transfer. However, having the projection data to reconstruct additional CT images with different kernels would provide the opportunity to apply advances in CT image reconstruction in the future and could improve CT image quality throughout a particular study cohort.
Summary of the Recommended Quantitative Lung CT Scanning Protocol
In summary, the 2001 “Workshop: Quantitative Computed Tomography Scanning in Longitudinal Studies of Emphysema” recommended the following parameters for the quantitative lung CT scanning protocol to assess emphysema: 16-row MDCT scanner, same manufacturer and model for each patient over the course of the study; x-ray tube peak voltage of 120 kV, x-ray tube current time product of 20 to 100 mAs; effective radiation dose between 0.7 and 10 mSv, spiral scan mode, pitch between 1.0 to 2.0, maximum detector element z-axis width of 1.25 mm; coach patient to TLC; FBP method of image reconstruction using a neutral kernel, such as the GE Standard CT kernel; DFOV to include just the lungs, reconstruct axial images with 1- to 1.25-mm thickness and 7-mm or greater slice thickness; no intravenous contrast media; quality control should include regular scanning of appropriate CT phantoms, projection data and reconstructed images should be saved, and transfer of image data to central image processing facility for research studies via the internet or on DVDs ( Box 3.2 ).
- 1.
MDCT scanner, 16-row or greater (see Single versus Multiple Detector Row CT Scanners in this chapter)
- 2.
Use the same CT scanner manufacturer and model for the same patient over the course of a study
- 3.
Effective radiation dose = 0.7–10 mSv
- 4.
X-ray tube peak voltage = 120 kV
- 5.
X-ray tube current time product = 20–100 mAs
- 6.
Spiral scanning mode
- 7.
Pitch = 1.0–2.0
- 8.
Verbally coach patient to total lung capacity (TLC)
- 9.
Display field of view (DFOV) just large enough to include both lungs–—using a reconstruction matrix size of 512 × 512
- 10.
Reconstruction method
- a.
Filtered back projection (FBP)
- b.
Neutral reconstruction kernel (e.g., no smoothing or edge enhancement)
- a.
- 11.
Axial CT image slice width = 1.0–1.25 mm OR ≥7 mm
- 12.
No intravenous contrast material
- 13.
Regular quality control checks to include CT phantoms (see X-ray CT Phantoms for Image Quality Assessments in this chapter)
- 14.
CT projection data along with reconstructed CT images should be transmitted to a central image processing facility using the internet or on DVDs
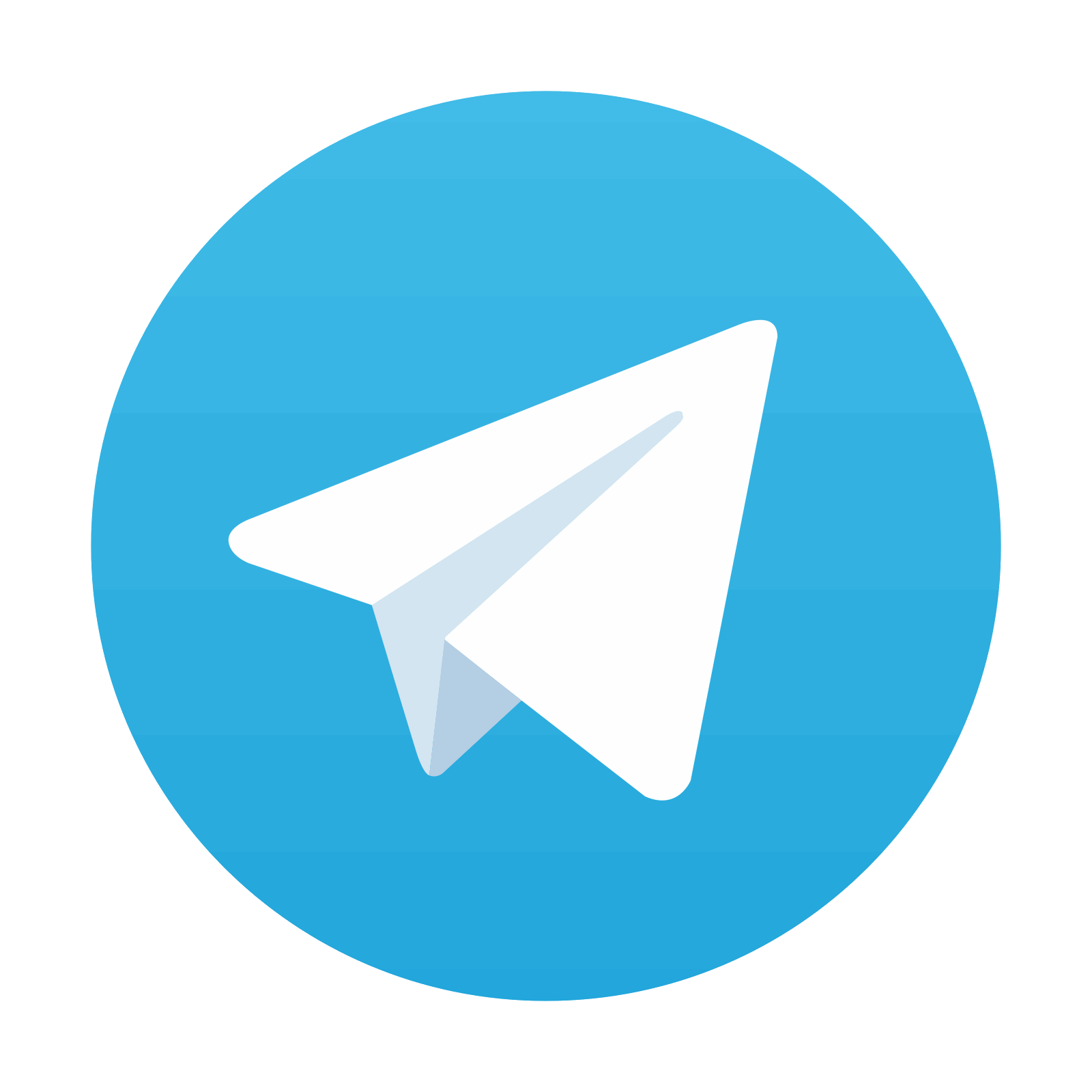
Stay updated, free articles. Join our Telegram channel

Full access? Get Clinical Tree
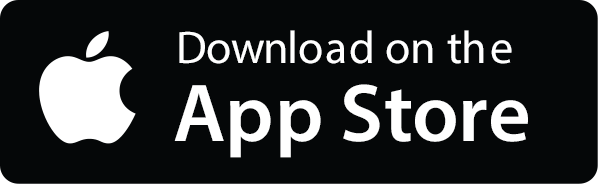
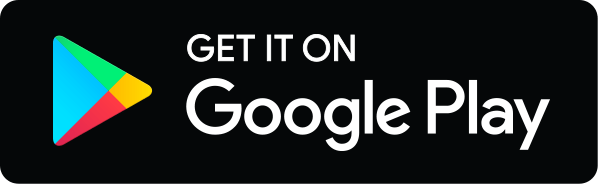
