Wounds, Bites, and Stings
INTRODUCTION
Wound healing and patients with altered or delayed healing cover the entire spectrum of surgical care. This chapter will review wounds, wound healing and closures, coverage options, and abnormalities in wound healing. It will focus on general principles and concepts rather than specific topics since the majority of these are dealt with in greater detail in other chapters. First, the biology and pathophysiology of wound healing will be explored in depth. Next, bites and stings, including emerging threats in the United States, will be detailed as our knowledge in these areas continues to advance.
Each year in the United States, millions of individuals sustain traumatic injuries but the overwhelming majority of these are simple and readily treated with minor interventions. In practical terms, satisfactory healing of wounds such as these would take place regardless of the treatments that they received. This chapter will focus on more severe wounds or those wounds that would not have a satisfactory outcome without special attention. The main principles in treating these wounds are preventing infection, retaining maximal function, and achieving acceptable cosmesis. The spectrum of health care providers who care for these wounds is extensive, including emergency medicine physicians, advanced practice nurses, family physicians, surgeons, and surgical subspecialists, but the treatment philosophy is the same. The nature and mechanism of the wound, its anatomic location, preexisting comorbidities, and the current clinical situation all dictate the approach to the wound but in general the simplest technique that fulfills the previously stated objections should be embraced. Fig. 47-1 provides a treatment algorithm for acute wounds that is applicable to a wide array of clinical scenarios and wounds.
FIGURE 47-1 Algorithm for the treatment of an acute wound. aAppropriate tetanus, rabies, and antibiotic prophylaxis. bIntermediate-risk wounds require more judgment. cSee Table 47-9.
Wound healing has always held an important status in medical care throughout recorded history. The Egyptians practiced advanced wound care as evidenced by the Edwin Smith and Ebers papyruses as did the ancient Greeks whose doctrine on the care of acute and chronic wounds in gladiators was described by Galen of Pergamum.1 The ancients observed that dead tissue and foreign bodies had to be removed in order for normal wound healing to progress and that cleanliness prevented infection. They recognized that organized collections of pus required drainage and that honey (a hypertonic, hygroscopic, and bactericidal fluid) could prevent infections while dirt and dung promoted them. However, the biology of this effect was a mystery to them.2 Later in the 1500s, the scholarly treatise on wounds by French surgeon Ambroise Pare established a truism that is still applicable today: “Do not put anything in a wound that you would not put in your own eye.” Despite the seminal contributions of Lister, Semmelweis, Ehrlich, Fleming, and Florey, it was not until the very end of the 20th century that our understanding of the biology of wound healing grew to the point that physicians are now able to manipulate wounds utilizing translational techniques taken from cellular and molecular biology.
Wounds are generally classified into one of the following two categories: acute and chronic. Acute wounds follow a systematic, organized series of stages that ultimately result in restoration of skin integrity. In contrast, chronic wounds follow many of the same steps but do not result in reestablishment of skin integrity. Generally, a wound that fails to fully heal by 3 months is said to be a chronic wound. However, such an arbitrary definition fails to consider the size or anatomic location of the wound or other impediments to healing. We will focus on the healing of acute, cutaneous wounds since they represent the vast majority of traumatic wounds.
NORMAL WOUND HEALING
Unlike humans, many lower life forms have the ability to regenerate after wounding. In humans only the liver and bone retain the ability to heal by regeneration. Excluding these two tissues, all other mammalian tissues heal through scar formation. The steps of scar formation or wound healing are classically divided into three distinct phases: inflammatory, proliferative, and remodeling (Table 47-1). These phases usually take place in a stepwise fashion, although, as we will discuss later, there are varying degrees of overlap and in certain wounds all phases may coexist simultaneously.
TABLE 47-1 Phases of Wound Healing
Inflammatory Phase
The inflammatory, or reactive, phase constitutes the first phase of wound healing and commences as soon as the injury occurs. All traumatic wounds lead to bleeding, disturbances in hemostasis, and an inflammatory reaction due to cellular destruction. The main goals of this stage are to stop bleeding, prevent infection, and remove devitalized tissue. Wounding invariably leads to disruption of blood vessels with ensuing bleeding that ranges from a slow ooze from capillaries to an exsanguinating hemorrhage if large arteries or veins are involved. In order to stop the bleeding, coagulation of blood must occur in a tightly regulated fashion so that clotting is confined to the site of injury but not at remote vascular locations. Blood vessel injury leads to the exposure of subendothelial, collagen, thrombin, and other procoagulant elements that bind and activate platelets. An important early response to vascular injury is contraction of the smooth muscle within the endothelium. Endothelin, a powerful vasoconstrictor, is released directly from the endothelium of the damaged vessel, an event occurring prior to the activation of platelets.3 Prostaglandins from injured cells, as well as circulating catecholamines following the trauma, also contribute to this early vasoconstrictive response. Additional stimuli for vasoconstriction occur via platelet activation with the resultant release of bradykinin, fibrinopeptides, serotonin, and thromboxane A2.
Platelet activation leads to a chain reaction culminating in the formation of an aggregate of platelets known as the “platelet plug.” Subendothelial matrix collagen interacts with circulating platelets leading to subsequent platelet adhesion. This is achieved via a complex of the platelet glycoproteins VI, Ib–V–IX, and collagen-bound von Willebrand factor on exposed collagen, as is mediated by platelet integrins. Since platelets are the first major cell to respond to wounded tissue, activated platelets serve as the platform on which clotting factors interact to form an insoluble fibrin meshwork that is generated from soluble, circulating fibrinogen. This fibrin mesh is cross-linked and traps circulating erythrocytes and activates additional platelets resulting in a tight, hemostatic plug.4 Shortly after coagulation is initiated, the complement cascade is set also in motion that leads to the release of potent neutrophil chemotactic factors, such as C5a.5 Large wounds or massive trauma may lead to an overly robust activation of this complement cascade and is thought to contribute to the subsequent development of the systemic inflammatory response syndrome (SIRS).6 C5a is believed to play a key role in the apoptotic response that occurs in immune cells shortly following a traumatic event.7 Complement is also involved in the destruction of bacteria through formation of the membrane attack complex, as well as opsonizing bacteria that facilitates their phagocytosis.
Effective hemostasis leads to a relative lack of blood flow to the wound microenvironment. This, coupled with anaerobic metabolism by leukocytes, leads to a locally hypoxic, acidotic wound environment. Such settings are known to be a stimulus for leukocyte activation so that coagulation is quickly followed by inflammation, but there is some overlap in these processes.8 Activated platelets and endothelial cells release cytokines that attract neutrophils and macrophages to the area. If thrombin is present, endothelial cells exposed to leukotrienes C4 and D4 will release the potent proinflammatory phospholipid platelet-activating factor (PAF) that enables neutrophil adhesion.9 Excessive PAF activation and involvement may lead to chronic and poorly healing wounds.10 Accordingly, by the end of the first hour and for the first 2–3 days after wounding, the dominant cell type in the wound is the neutrophil. The main purpose of the neutrophil is destruction of bacteria through phagocytosis and the generation of reactive oxygen species through a process called “respiratory burst.” Respiratory burst results in the formation of many reactive oxygen species such as superoxide anion (SO2−), hydrogen peroxide, hydroxyl radicals, and hypochlorous acid. Neutrophils also debride the wound by elaborating activated proteases and elastases that break down devitalized tissues and cells. Unfortunately, the release of activated lysosomal enzymes along with reactive oxygen species leads to the indiscriminant collateral damage of otherwise healthy host cells as well.11 Ultimately, the action of neutrophils leads to the generation of pus that is simply a collection of dead neutrophils and bacteria along with tissue breakdown products. Once neutrophils have completed their job, they undergo apoptosis, or programmed cell death, and are eliminated by macrophages in a process that is remarkably free of inflammation.
Of all the cells involved in wound healing, none is as critical as the macrophage.12 Activation of platelets, endothelial, and other cells in the wound leads to the generation of inflammatory substances such as histamine, serotonin, prostaglandins, kinins, platelet-derived growth factor (PDGF), transforming growth factor beta (TGF-β), insulin-like growth factor-1 (IGF-1), fibronectin, fibrinogen, von Willebrand factor, thrombospondin, and thromboxanes. These substances have many varied effects including increasing capillary permeability by disrupting the integrity of the endothelium, both vasoconstriction and vasodilatation, and the recruitment of several other inflammatory cell types. Monocytes respond to chemotactic signals such as fibrin and fibrinopeptides and leave the blood vessels via diapedesis. This migration is facilitated by capillary leak and interstitial edema that results from the action of inflammatory substances such that by the end of 1.5 days postinjury the numbers of monocytes in the wound reaches its peak. The inflammatory milieu of the wound then stimulates the monocyte to become a macrophage and it is these macrophages in conjunction with resident tissue macrophages that orchestrate the entire wound healing process.
Macrophages release over 20 different growth factors and cytokines and can perform a wide range of functions so that they are truly the central cell in wound healing.13 Just like the neutrophil, macrophages contribute to clearing the wound of bacteria, although neutrophils remain the prime antibacterial cell in the wound. The phagocytic function of macrophages is not limited to bacteria alone since they also clear away apoptotic neutrophils and other cells via phagocytosis. Recent evidence is emerging pointing to a key role of invariant natural killer T cells in the proper functioning of macrophages for acute processes such as wound healing and bacterial clearance.14 Unlike neutrophils, phagocytosis is not the main function of the macrophage and this activity is typically manifested only in the very early stages of wound healing. As the wound is cleared of bacteria, the macrophages release growth factors that reinforce endothelial cell activation and are chemotactic signals for various cells. Activated endothelial cells display adhesion molecules so that additional inflammatory cells can bind to the activated endothelium and be recruited into the wound.15
Initially, leukocytes tether and roll on the exposed vascular endothelial cells, before they are activated to adhere firmly with the ultimate goal of migration into the extravascular space. Leukocyte tethering and rolling is primarily mediated via selectins. The later firm adhesion is mediated via the immunoglobulin (Ig) superfamily members, including intercellular adhesion molecule-1 (ICAM-1) and vascular cell adhesion molecule-1 (VCAM-1), and their integrin ligands, including lymphocyte function-associated antigen-1 (LFA-1, CD11a/CD18) and very late antigen-4 (VLA-4, CD49d/CD29). The three cell-surface molecules expressed by leukocytes (L-selectin), vascular endothelium (E- and P-selectins), and platelets (P-selectin) comprise the selectin family. Each selectin exhibits a characteristic time frame. L-selectin is constitutively expressed on most leukocytes, whereas ICAM-1 is constitutively expressed at low levels by endothelial cells. ICAM-1 can be rapidly upregulated during inflammation. P-selectin is rapidly mobilized to the surface of activated endothelium or platelets. E-selectin expression is induced within several hours after activation with inflammatory cytokines. The combination of endothelial cell activation and the release of chemotactic and growth factors leads to the next stage of wound healing, the migratory phase.
Migratory Phase
The next phase of wound healing is the migratory phase. The initial “platelet plug and clot” need to be replaced with cells capable of achieving proper closure of the gap that was created from trauma, as well as to replenish the lost tissue. While the migratory phase is initiated early on in the wound healing process, its duration is highly variable and dependent on the size and nature of the wound that needs to be healed. The interaction of activated endothelial cells with circulating inflammatory cells leads to the margination of leukocytes. At this point, there is significant alteration in the cell–cell and cell–matrix adhesions to enable the progression from a laminin-V-and collagen-IV-rich basement membrane to provisional matrix substances.16 Migrating cells also assemble actin-rich lamellar protrusions that will enable crawling,17 and upregulate the expression of the proteolytic enzymes such as matrix metalloproteinases (MMPs). The migrating cells traverse the blood vessel wall through diapedesis and migrate along chemotactic gradients to the site of injury. Meanwhile, macrophages are progressing from bacterial phagocytosis to the coordination of the complex cell–cell interactions and are now the dominant cell type in the wound supplanting neutrophils and monocytes. The relatively hypoxic wound environment causes macrophages to release angiogenic growth factors, key among which is hypoxia-induced factor (HIF).18 HIFs are transcriptional complexes that are regulated by protein degradation, thereby allowing a swift response. Using an HIF-knockout mouse, investigators have shown that HIF-alpha deficiency leads to a pattern of multiorgan dysfunction with significant anaerobic metabolism.19 A further key player in angiogenesis is PDGF that is released by activated platelets.20,21 Vascular endothelial growth factor (VEGF) stimulates angiogenesis in a strict dose-dependent fashion.22 Many other factors, such as angiopoietin-2/angiopoietin-1,23 PDGF, basic fibroblast growth factor (bFGF), and monocyte chemoattractant protein-1 (MCP-1),24 have been shown to be indispensable in increasing vascular permeability, endothelial growth, remodeling, cell proliferation, and vascular lumen formation. PDGF also stimulates fibroplasia by stimulating the normally present but quiescent fibroblasts to begin replicating by releasing them from their G0 arrest. Cytokines such as IGF-1 and epidermal growth factor (EGF) released from activated macrophages and platelets cause the fibroblasts to begin replicating. Additional fibroblasts from outside the wound environment arrive by traveling over the scaffold of the fibrin clot by binding to fibronectin within it. While angiogenesis and fibroblast proliferation occur simultaneously, it is obvious that like all cellular synthetic functions, fibroblast synthetic function is dependent on oxygen. Thus, in the healing wound, angiogenesis and fibroplasia are interconnected processes.25
Angiogenesis is dependent on the action of endothelial cells derived from the nearby uninjured portion of blood vessels.26 These endothelial cells first generate plasminogen activator to degrade the clot at the site of vessel injury so that they can migrate out into the extracellular matrix.25 This action is reliant on the activity of zinc-dependent MMPs that are required to dissolve the basement membrane and collagenases.26 Specifically, MMP-2 and MMP-9 have been shown to be prominent in the angiogenic response to hypoxia. Neutrophil granules are potent sources of MMP-9. Further neutrophils can release tissue inhibitors of matrix metalloproteinases (TIMP-1) pro-MMP-9 that can activate latent pro-MMP-9 in the wound environment.27 The newly “liberated” endothelial cells then migrate through the surrounding extracellular matrix by extending pseudopodia, just like leukocytes do, in response to chemotactic signals released by platelets and macrophages. The relatively hypoxic local wound environment leads to the formation of lactic acid through anaerobic metabolism, which has been shown to be a potent stimulus for macrophages to release endothelial cell growth factors.13 When tissue oxygen tension improves and the macrophages are no longer hypoxic, they stop elaborating endothelial cell growth factors in an elegant feedback loop controlling angiogenesis. Further, with restoration of oxygen to the wound, HIF-1alpha proteins are downregulated by the oxygen-dependent inhibitor, factor inhibiting HIF-1 (FIH-1).28 Thus, wound revascularization removes the stimulus for endothelial cell growth and migration and similarly to the preceding neutrophils, the endothelial cells also undergo apoptosis. The typical beefy red color of granulation tissue is a result of dense collections of capillaries formed through the process of angiogenesis.
While angiogenesis is occurring within the wound, reepithelialization is occurring at the margin of the wound. This process begins approximately 24 hours postinjury and is driven primarily by transforming growth factor alpha (TGF-α) that is released by platelets and macrophages. If a wound is sufficiently deep enough to destroy the stratum basale of the skin, keratinocytes from the wound edges or from deep skin appendages such as hair follicles, sweat glands, or sebaceous glands spared by the wounding insult serve as the source for reepithelialization. Interestingly, reepithelialization of the wound is almost entirely dependent on migration of keratinocytes, which occurs as sheets of cells move in from the wound periphery, rather than mitosis of keratinocytes within the wound. In order for keratinocytes to migrate they must detach themselves from their basement membrane by dissolving their anchoring desmosomes. Following this, they display integrins and migrate across the early wound matrix using fibronectin cross-linked with fibrin as adhesion sites exactly like the fibroblasts before them. As the keratinocytes migrate they elaborate MMPs, collagenases, plasminogen activator, and other proteases to dissolve debris as well as phagocytosize dead cells blocking their path.
As the sheet of migrating epithelial cells advances, new cells are formed at the wound margin by greatly upregulated mitosis to replenish the advancing cells. The rate of mitosis at the wound margin may be 17 times higher than that seen in normal tissue and remains the only focus of epithelial cell mitosis in the wound.29 The migration continues until keratinocytes encounter other cells that stop their advancement through contact inhibition, and downregulation of their fibronectin receptors that are necessary for their movement.25 Reepithelialization proceeds more rapidly if the basement membrane is intact, otherwise new basement membrane substances must be secreted.30 Keratinocytes will then reanchor themselves to the basement membrane and each other using desmosomes and hemidesmosomes and normal skin stratification is established by cell division of the basal layer of cells. Critical to this stability is the interaction between the hemidesmosomes, integrin-alpha6-beta4, and plectin.31
Thus, by the conclusion of the migratory phase, new blood vessels are being formed and fibroblasts are appearing in the wound and begin replicating so that fibroplasia may take place, while at the wound margin an advancing sheet of epithelial cells moves inward. Table 47-2 reviews some of the more important cytokines and growth factors involved in the regulation of wound healing.
TABLE 47-2 Cytokines and Growth Factors
Proliferative Phase
The next phase of wound healing, the proliferative phase, is marked by the migration of multiple cell types including monocytes, lymphocytes, and endothelial cells into the wound along with the emergence of active fibroblasts. This phase usually commences around day 5 and is characterized by the dramatic increase in the number of cells and the production of collagen. The production of collagen may be detected as early as 10 hours after injury. However, collagen production reaches maximum velocity by day 7. Collagen synthesis continues at a vigorous pace until approximately day 21 when the wound approaches its peak of collagen content and collagen production plateaus. This is the physiologic basis for the practice of leaving retention sutures, used to reinforce tenuous laparotomy closures, in place until day 21.
Eventually, granulation tissue is formed as the fibrin–fibronectin network that comprises the blood clot along with the makeshift wound matrix of proteoglycans, glycosaminoglycans, and hyaluronic acid is replaced by a combination of collagen, new capillaries, and numerous inflammatory cells and fibroblasts. This process is largely accomplished by fibroblasts that interact with several adhesive ligands in the wound matrix such as laminin, tenascin, and fibronectin while they release hyaluronidases that degrade the provisional wound medium. This early wound continues to evolve as collagen is haphazardly laid down on the fibronectin and glycosaminoglycan scaffold. The amount of granulation tissue in the wound is dependent on the size and depth of wounds that are allowed to heal by secondary intention. Large wounds need to “fill in” with granulation tissue so that epithelial cells from the wound margin can migrate across and reepithelialize the wound. Alternatively, the development of a healthy bed of granulation tissue is the sign that a wound is ready to accept an autologous skin graft. It is the rich network of capillaries along with the relatively aqueous environment that supports the transplanted epithelium by bathing it in a flow of nutrient-containing fluid and oxygen. Full-thickness skin grafts are an important modality to mitigate the next step of wound healing that is contraction.
Wounds that are allowed to heal by secondary intention, and to a much lesser degree wounds closed primarily, undergo the phenomenon of wound contraction, which is a process whereby the wound edges and surrounding skin are pulled in toward the center of the wound. Contraction usually starts around 1 week after wounding, peaks in intensity around day 10, and may persist for weeks with a reported speed of nearly 0.75 mm per day. This process is a highly efficient way of reducing the surface size of the wound and decreases the amount of reepithelialization that needs to occur. Unfortunately, this process can be particularly debilitating if the wound overlies a joint, flexible part of the body or crucial orifice such as the eye or mouth. Significant loss of function due to the presence of a contracting wound is clinically called a “contracture.” Differences in the thickness of skin in various anatomic locations along with the laxity of the surrounding skin lead to variable degrees of contraction. Typically, the perineum and trunk experience the greatest degree of contraction while the extremities exhibit the least, while the head and neck exhibit an in-between amount of wound contraction. The exact mechanism of wound contraction is not well elucidated but appears to be dependent on modified fibroblasts that contain microfilaments and alpha smooth muscle actin and phenotypically resemble smooth muscle cells.32 The true phenotypic characteristics and capabilities of myofibroblasts are still poorly understood, but knowledge is growing as the role of the myofibroblast in pathological processes such as tumor dissemination is being better appreciated.33 Myofibroblasts are richly endowed with adhesion molecules in order to complex with a vast array of components making up the early wound environment. It is these attachments that allow them to bind and draw the wound edges together. As the wound contracts, other fibroblasts continue to synthesize collagen that effectively holds the wound together.
Nearly 20 different types of collagen have been identified and all share a common structural similarity known as the right-handed triple helix. Each type of collagen has unique structural properties that are attributed to specific breaks in the triple helix pattern. In general, collagen triple helices are composed of three alpha peptide chains that undergo extensive modification both in and outside the fibroblast. Collagen is the major component of all human tissues and is distinctive in that it contains large quantities of the unique amino acids hydroxyproline and hydroxylysine. The hydroxylation of specific prolines in the early peptide chain is extremely important because error at this stage of collagen synthesis leads to the creation of an unstable collagen that is quickly degraded in the extracellular environment. The hydroxylation of proline and lysine requires ascorbic acid (vitamin C), which is the mechanism whereby vitamin C deficiency leads to impaired wound healing, and even opening of previous healed wounds, as seen in the disease scurvy.
Regulation of collagen synthesis occurs at many levels, beginning with the transcription. Collagen transcription takes place on several different chromosomes from discontinuous genes resulting in a large precursor molecule that must be modified within the nucleus; thus, only limited amounts of messenger ribonucleic acid (RNA) are created. This highly modified mRNA then undergoes translation on ribosomes of the endoplasmic reticulum so that by the time the collagen molecule is ready to leave the Golgi apparatus, it has undergone significant glycosylation with glucose and galactose and has had “extension” peptides placed on its amino and carbon terminals to facilitate its orientation and handling. These procollagen monomers leave the cell where additional modification takes place extracellularly, resulting in highly cross-linked collagen fibers. The extracellular modification process is fairly unique to collagen, and it is during this juncture that the extension peptides are cleaved from the end of procollagen, which are then taken back into the cell and downregulate collagen expression. At the end of this stage of wound healing, sufficient collagen fibers have been synthesized and the wound is more appropriately called a scar.
Remodeling Phase
Although it seems contradictory, it is true that collagen must be degraded at the same time it is created in order for normal wound healing to occur. When the rate of collagen synthesis is balanced by an equal rate of collagen degradation, the wound is said to have reached the remodeling or maturational phase. This phase of wound healing may last for several months or even years for large wounds that heal by second intention. The tensile strength of the wound increases over time as the disorganized type III collagen that was laid down initially is degraded by MMPs and slowly replaced by type I collagen. The activity of the MMPs is itself regulated by TIMPs so that a balance between synthesis, deposition, and degradation of extracellular matrix is maintained.34 Progression from laying down collagen haphazardly to the organized structure seen at the end of the remodeling phase requires a careful balance within the wound environment. This balance may be quite delicate as evidenced by the role of arginine. Therapeutic supplementation with arginine has been proposed as a safe method of providing the necessary proline and polyamines to improve wound healing; however, excess arginine has been associated with poor collagen matrix formation, impaired collagen remodeling and cross-linking, and altered wound tensile strength. It is believed that this occurs via excess nitric oxide production rather than ornithine and an imbalance in the pace of collagen remodeling.35
As type I collagen is laid down and oriented parallel to lines of stress, the tensile strength of the wound increases. This increase is most rapid during the first 6 weeks, and then slows down but may persist for over a year or more. The tensile strength of the wound approaches 50% of normal skin by 3 months after injury and plateaus out about 80% by the end of remodeling, although this process continues on at an extremely slow rate for several more years. The increase in tensile strength is attributed to collagen cross-linking since the collagen content of the wound does not change beyond the third week. The scar that was originally red or purple in color due to the tremendous amount of capillaries it contains gradually turns white as these are readsorbed and replaced with type I collagen. The final result of the repair is an inelastic, avascular, brittle scar that is devoid of skin appendages such as hair follicles and sweat glands and never recovers more than 80% of the tensile strength of unwounded skin.
CLINICAL MANAGEMENT OF WOUNDS
Types of Wound Closure
Wound closure falls into one of the following four general categories: primary, secondary, delayed primary, and tissue transfer. In primary closure, the cutaneous defect is approximated through the use of sutures, staples, adhesive dressings, or topical sealants and the previously described phases of wound healing occur albeit on a limited scale. These wounds are typically closed in layers along tissue planes and derive their strength from collagen-rich layers such as dermis or fascia. The duration of healing and the final cosmetic result are closely linked to the amount of devitalized tissue, bacteria, and blood left in the wound at the time of closure. Meticulous debridement of the wound along with scrupulous hemostasis minimizes the inflammatory phase of wound healing and allows the wound to progress rapidly to the migratory and proliferative phases of the healing process and promotes earlier wound closure with improved cosmesis as well. The decision to close a wound primarily is based on the judgment by the clinician that the wound is at minimal risk for wound infection or that subsequent wound infection after primary closure is less detrimental than open management of the wound.
Wounds that are felt to be at excessive risk for infection are best left open to heal by secondary intention. Spontaneous or secondary intention closure occurs when the wound edges are not reapproximated and the wound must undergo contracture, granulation, and reepithelialization in order to close. Open wounds are much less likely to become infected, but they are not immune to wound infection. The presence of increased bacteria and tissue debris leads to a longer, more intense inflammatory phase that results in more scarring and decreased cosmesis. Failure of healing by secondary intention leads to a chronic wound and in large part is due to a fundamental shift in the type and quality of wound inflammation. Chronic wounds, which are practically defined as a wound that has failed to close within 3 months, exhibit much higher levels of collagenases and MMPs, yet have reached a point of stasis and do not ultimately heal.
In delayed primary closure, a wound is left open for a few days and is treated much the same as a wound that is healing by second intention. The difference is that after a certain time the wound is then closed primarily. The main reason that a wound is handled in this fashion is that there is too much bacterial contamination, tissue trauma, or foreign bodies to allow a primary closure and the wound is left open so that wound care and dressing changes can remove these impediments to infection-free healing.36 There tends to be more inflammation in wounds that are managed by delayed primary closure than in those managed by primary closure, but eventually these wounds exhibit the same tensile strength and cosmesis as wounds that were initially closed primarily and with lower infection rates.36 Some authors have advocated delayed primary closure, rather than closure by secondary intention, for gunshot wounds. However, this needs to be applied on a case-by-case basis.37
Open wounds and those with extensive soft tissue loss that are technically difficult to close are best treated by application of a skin graft or tissue transfer. In general, the simplest technique that will result in a closed wound should be employed so that a flap should not be done when a skin graft would suffice, and a free flap should be avoided if a rotational flap will be satisfactory. The timing of grafting or flapping is somewhat controversial and is best determined by the overall physiologic condition of the patient. Vascular anastomoses and exposed blood vessels are special cases and need to be covered as soon as possible to prevent catastrophic “blowouts.” In patients with an open abdomen it is highly desirable to get coverage of intestinal anastomoses with omentum, absorbable mesh, or the abdominal wall since uncovered they have a tendency to break down leading to devastating “enteroatmospheric fistulas.”38 Split-thickness skin grafts are usually applied 5 days after injury but this is based more on tradition than physiology.
Wound Management Decision Making
The way a wound is treated is dependent on several decisions and sound clinical judgment. The first determination in whether to close a wound or leave it open is related to the time since injury. In general, wounds that have been open for more than 6–8 hours should be left open, otherwise primary closure is advantageous. Obviously, there are many exceptions to this “rule” since open wounds on the face or scalp tend to do well even when closed more than 8 hours after wounding. This observation is due to the relatively low levels of commensal bacterial colonization coupled with an outstanding blood supply in these locations. On the other end of the spectrum, a 4-hour-old wound in an ischemic extremity in a diabetic is best left open since neither bacterial flora nor blood supply favors infection-free healing.
The next consideration in establishing a treatment plan for the wound is based on the mechanism of wounding. High-velocity gunshot wounds, crush injuries, close-range shotgun wounds, and high-energy open fractures are all characterized by extensive soft tissue destruction and are frequently complicated by wound infection.39 Heavily contaminated wounds such as human bites, farming-related accidents, and wounds with gross contamination, especially soil, are at exceedingly high risk for subsequent wound infection and are best managed open. These contaminated wounds are at risk for tetanus and the patient should receive tetanus prophylaxis. Tetanus prophylaxis is detailed in Table 47-3, while Table 47-4 reviews the characteristics of a wound that is tetanus prone. Guidelines have been published detailing the indications and considerations for tetanus and other vaccines following traumatic injuries.40
TABLE 47-3 Tetanus Prophylaxis
TABLE 47-4 Wound Characteristics Relating to Likelihood of Tetanus
In addition to local wound factors, systemic conditions can also increase the incidence of wound infection. Hemorrhagic shock has been identified as a strong risk factor for wound infection.41,42 This phenomenon is likely multifactorial since trauma and hemorrhage affects blood flow on both the global and tissue levels and causes immune,43 hemtapoeitic,44 and myelopoeitic45 bone marrow dysfunction. Diabetes,46 connective tissue disorders,47 advanced cancers,48 immunosuppressed states, atherosclerosis, hypothermia, malnutrition,49 COPD, corticosteroid therapy, and a multitude of other systemic disease processes all negatively impact wound healing and need to be considered in the wound treatment plan. Thus, the decision to close a wound is based on a series of assessments of the mechanism of injury, anatomic location, degree of contamination, and physiologic and medical condition of the patient.
In addition to the factors predisposing to wound infection discussed above, additional variables leading to wound infections have been identified. These threats to infection-free healing are listed in Table 47-5. Devitalized tissue is a common finding in high-velocity gunshot and high-energy wounds and represents a major risk factor for wound infection. Such tissue acts as a culture medium for bacteria, is poorly penetrated by immune cells and antibiotics, and incites an increased inflammatory response. Debridement is an effective way of removing this source of infection and inflammation and is an integral part of wound care.50
TABLE 47-5 Threats to Infection-Free Wound Healing
Meticulous hemostasis is often cited as a key component to normal wound healing; however, the rationale behind this statement is frequently omitted. Iron is an essential component for bacterial replication,51 so it is tightly regulated in vivo by sequestration in macrophages as well as by hepcidin, an iron-binding protein released by the liver in response to inflammation.52 Wound hematomas lead to increased infections by overwhelming the body’s ability to restrict iron and inhibit bacterial growth, and thus render a wound susceptible to infection by smaller bacterial numbers. In a similar fashion, foreign body contamination also leaves a wound more susceptible to smaller bacterial burdens by reducing granulocyte phagocytosis. If adequate hemostasis cannot be obtained due to coagulopathy or extensive injury or if debridement is inadequate, it is best to postpone primary closure until these conditions are corrected. Such wounds can be managed as open wounds and then treated by delayed primary closure at the bedside or taken to the operating room for further debridement and eventual closure.
Operative Versus Nonoperative Wound Management
Similar to the decision process regarding closure of a wound, the setting of wound care is equally important. In general, large complex contaminated wounds containing foreign bodies or devitalized tissue are best managed in the operating room. The anatomic location of the wound can be critical as well since perineal, facial, and severe hand injuries can have serious consequences if initially mismanaged. Noncompliant patients and those with hazardous communicable diseases are best treated in the operating room for safety reasons for the patient and clinician alike. Although a wound may be impressive, it is frequently the associated injury that is most threatening to the patient. A 2-cm neck wound is not impressive; however, it may lead to a disastrous sequence of events if it overlies the carotid artery. In general, attempting to manage a major wound without adequate light, exposure, anesthesia, equipment, or patient cooperation leads to missed injuries, retained foreign bodies, inadequate debridement, wound hematomas, and soft tissue infections or worse. Table 47-6 summarizes some conditions mandating operative management of a wound.
TABLE 47-6 Conditions Requiring Management in Operating Room
Operative Issues
Once the decision is made to proceed to the operating room, the next step is to consider patient positioning and skin preparation. In general, the patient should be positioned in such a way so that access to other body cavities and surfaces is maintained. The lateral decubitus position offers excellent access to a hemithorax but does so at the expense of access to the contralateral chest and abdomen. Extremities are best fully prepped and free draped to maximize exposure and operative options.
After positioning, the patient’s skin is prepared with an antiseptic. As part of this preparation, body hair may need to be removed to facilitate wound exploration and exposure and to prevent hair from becoming trapped in the wound and functioning as a foreign body leading to increased wound infections. Shaving hair should be avoided since it has been shown to lead to higher wound infection rates when compared with depilatories or clippers.53 While depilatories may be useful in elective surgery, they have little role in the management of urgent traumatic wounds; thus, surgical clippers with disposable heads should be readily available in the emergency department and operating room. Hair should be removed in an atraumatic fashion so as not to cause additional injury. Loose foreign bodies and debris should be removed as well. Eyebrows should not be removed since in a minority of patients their regrowth can be inadequate.54
The most common skin antiseptics are povidone–iodine solution (Betadine®) and chlorhexidine gluconate solution (Hibiclens®). Both agents are rapidly bactericidal; however, emerging evidence shows that chlorhexidine is a superior prep agent since it has more residual activity and is associated with lower rates of infection.55–57 A 2% chlorhexidine in 70% alcohol solution has been shown to be the most efficacious form of chlorhexidine and is now available commercially (ChloraPrep®).58 Both povidone–iodine and chlorhexidine are toxic to normal tissue, so they should only be used as preparatory agents rather than topical wound treatments.59 Ambroise Pare admonished against putting anything into a wound that would not be tolerated in one’s eye, but this is especially true for chlorhexidine since it causes direct corneal injury.60,61 Povidone–iodine or hexachlorophene solution (PHisoHex®) should be used for prepping the face to avoid corneal damage and possible blindness.62
One of the hallmarks of operative management of heavily contaminated wounds centers on the use of high-pressure pulse lavage systems. In the past, it was felt irrigation had to be performed at high pressure in order to effectively remove microparticulate contaminants and bacteria.63 Lavage of contaminated wounds with a bulb syringe or low-pressure systems was thought to be ineffective. While commercially available high-pressure irrigating systems are undoubtedly effective, their destructive effects on already injured tissues are frequently overlooked. In addition to the increased devitalization of the wound, high-pressure irrigation can also have detrimental effects on immune function and wound healing.64
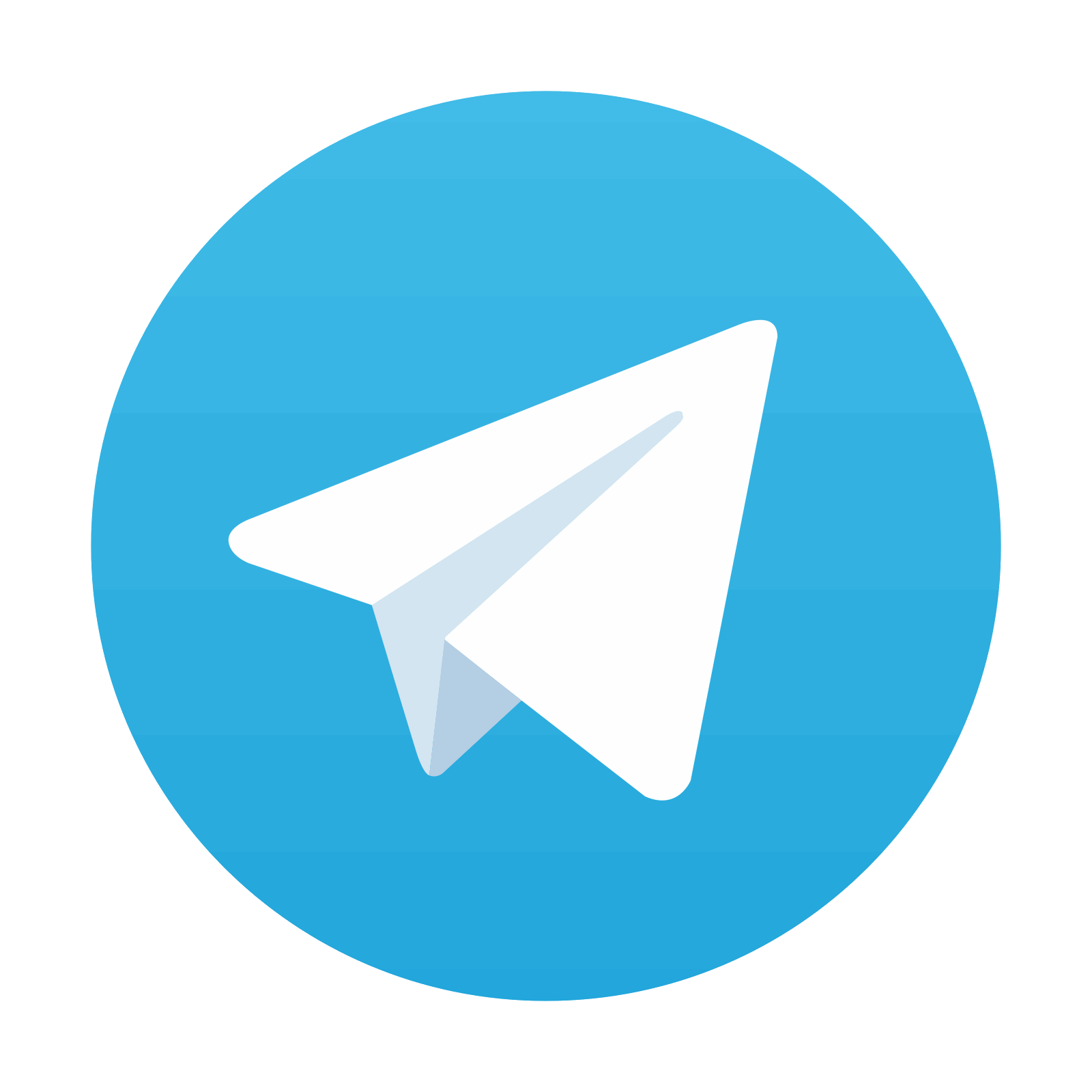
Stay updated, free articles. Join our Telegram channel

Full access? Get Clinical Tree
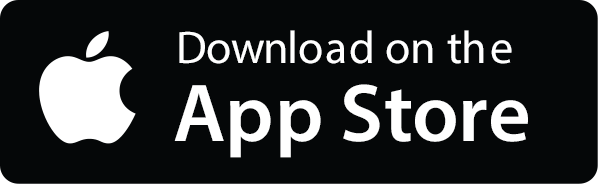
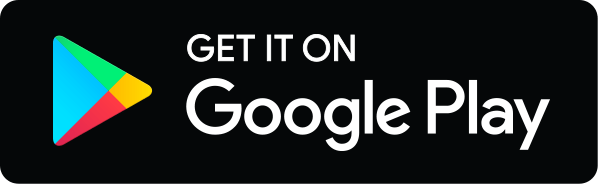