Definition and incidence of renal and right ventricular complications in heart failure with preserved ejection fraction (HFpEF). Blue: chronic features; purple: acute features. CKD, chronic kidney disease defined as an estimated glomerular filtration rate < 60 mL/min/1.73 m2; CMR, cardiac magnetic resonance imaging; LV, left ventricular; WRF, worsening of renal function defined as an increase in serum creatinine or decrease in diuresis
It is important to distinguish RV dysfunction from right heart failure. Right heart failure involves hemodynamic decompensation. Right heart failure presents with various associated clinical signs such as hypoxemia, signs of systemic congestion (jugular venous distension, hepatojugular reflux, peripheral edema, pericardial effusion, congestive hepatosplenomegaly, ascites or anasarca) and more specific signs of RV dysfunction such as those associated with tricuspid regurgitation (third heart sound, systemic murmur of tricuspid regurgitation, hepatic pulse, etc.) [3]. Signs of low cardiac output state (hypotension, tachycardia, cool extremities or oliguria) could be present. No specific biomarker of right heart failure has been described yet. Nevertheless, non-specific biochemical markers such as lactate, natriuretic peptides [brain natriuretic peptide (BNP) or N-terminal of the prohormone of BNP (NT-proBNP)], cardiac troponin I or T, but also liver biochemistry or markers of renal function (especially creatinine) [3] could guide the diagnosis and help to evaluate prognosis [4] in primary right heart failure such as for instance in the case of pulmonary arterial hypertension [5].
A comprehensive definition of right ventricular dysfunction remains elusive. Right ventricular dysfunction is used to characterize RV alteration mainly by imaging. It is based on the monitoring of RV function and is therefore not based on a clinical syndrome. Diagnosis of RV dysfunction could involve echocardiographic or cardiac magnetic resonance imaging analyses with various cut-off values [6]. Finally, a gold standard method to quantify RV dysfunction considers the RV–pulmonary artery coupling and involves invasive pressure measurements and acquisition of volume loops ideally associated with the hemodynamic tracings. Heterogenic cohorts and multiplication of diagnosis methods including echocardiographic criteria such as reduced RV fractional area change (FAC), tricuspid annular plane systolic excursion (TAPSE) and tricuspid annular systolic velocity (RV S′) or cardiac magnetic resonance imaging analyses contribute to the complexity of the estimation of RV dysfunction prevalence [7]. A recent meta-analysis found a RV dysfunction prevalence of 18%, 21% or 28% using RV FAC, RV S′ and TAPSE, respectively. TAPSE or RV FAC values are associated with mortality [6].
Stage 1 | Serum creatinine: 1.5–1.9 fold increase from baseline within 1–7 days or ≥26.5 μmol/L increase within 48 h Urine output: <0.5 mL∙kg−1/h for 6–12 h |
Stage 2 | Serum creatinine: 2.0–2.9 fold increase from baseline Urine output: <0.5 mL∙kg−1/h for ≥12 h |
Stage 3 | Serum creatinine: ≥3.0 fold increase from baseline or increase >354 μmol/L or initiation of renal replacement therapy Urine output: <0.3 mL∙kg−1/h for ≥24 h or anuria ≥12 h |
Pathophysiology of Kidney Dysfunction in Right-Sided Heart Failure
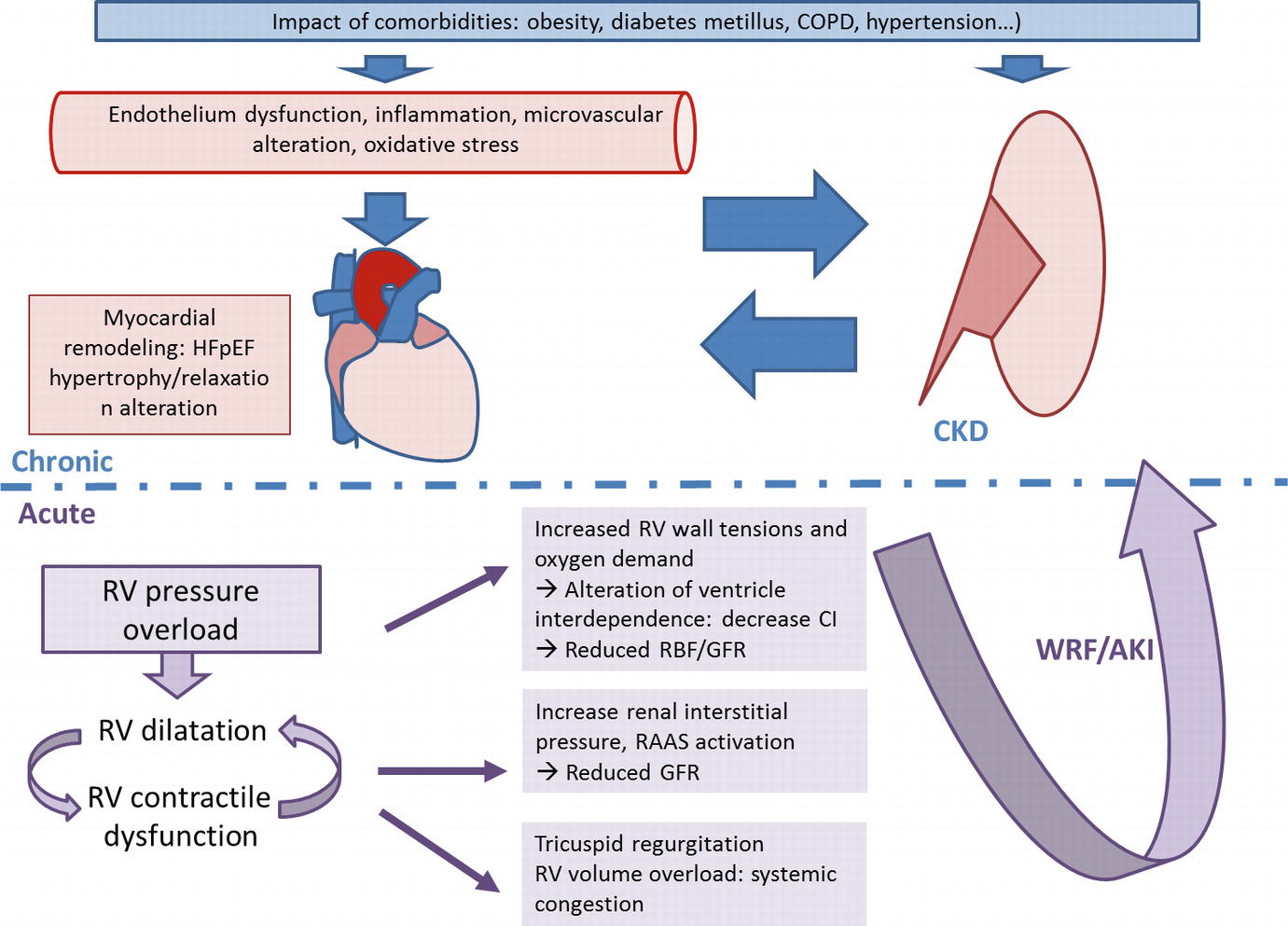
Chronic and acute physiologic mechanisms leading to renal dysfunction in heart failure with preserved ejection fraction (HFpEF). Blue: chronic features; purple: acute features. AKI, acute kidney injury; CI, cardiac index; CKD, chronic kidney disease; COPD, chronic obstructive pulmonary disease; GFR, glomerular filtration rate; RBF, renal blood flow; RAAS, renin-angiotensin-aldosterone system; RV, right ventricular; WRF, worsening renal function
Renal Dysfunction Secondary to HFpEF
Compared to HFrEF secondary to direct cardiomyocyte injury (ischemic, infectious or toxic mechanisms for example) with an alteration of calcium homeostasis and myocardial contraction, HFpEF is secondary to a set of conditions that cause systemic alterations, inflammation and endothelial dysfunction that eventually lead to pathological remodeling of the myocardium [8]. In this context, three pathways could be proposed to link chronic cardiac dysfunction and chronic renal dysfunction: (1) cardiac dysfunction leading to renal dysfunction (cardiorenal syndrome type 2), (2) renal dysfunction leading to cardiac dysfunction (cardiorenal syndrome type 4) and (3) common systemic mechanisms secondary to patients’ comorbidities leading to renal and cardiac changes (cardiorenal syndrome type 5).
HF induces CKD. Indeed, in 2013, Paulus et al. proposed a pathophysiological model to describe the relationship between HFpEF and CKD through systemic mechanisms such as inflammation, endothelial dysfunction, oxidative stress and microvascular disorders. Systemic disorders are mainly due to patient’s comorbidities [hypertension, diabetes, obesity, chronic obstructive pulmonary disease (COPD), etc.] by altering the protein kinase G pathway involved in cardiac remodeling (hypertrophy and relaxation mechanisms) [17]. The kidney proteome analyzed in an established model of HF in rats, shows an increase in various family proteins such as proteins involved in endothelial function [Von Willebrand factor (vWF), caveolin 1–3, T-kininogen 2], proinflammatory extracellular matrix activation [Microfibril-associated protein-4 (MFAP-4), collagen-VI, galectin-3, Four and a half LIM domains protein-1 (FHL-1), calponin] or glomerular filtration membrane integrity [Chloride Intracellular Channel-5 (CLIC-5), Zonula Occludens-1 (ZO-1)] [18]. Inversely, CKD is also associated with endothelial, microvascular and inflammatory dysfunctions. Nevertheless, specific mechanisms of CKD appear to be involved such as microalbuminuria, vitamin D deficiency or lack of erythropoietin. Those are all inducers of endothelial dysfunction, oxidative stress or inflammation [8]. Moreover, activation of the sympathetic nervous system in CKD has also been implicated in the development of cardiac failure [8]. In chronic state, distinction between causes and consequences of CKD in patients with HFpEF is not easy. In the context of combined renal and cardiac failure (cardiorenal syndrome type 5), Galectin-3 is a marker of interest that is currently emerging. Initially identified as a marker of cardiac fibrosis, it has been shown in animal models to be involved in the development of renal fibrosis [19] and to promote hypertensive nephropathy. Hypertensive nephropathy develops to a lesser extent after pharmacological inhibition of Galectin-3 [20]. More studies are necessary to confirm these observations.
Acute Kidney Injury Principally Related to Vascular Congestion
In acutely decompensated HFpEF, renal failure is related to decreased cardiac output, but also to venous congestion [21]. Damman et al. analyzed the relationship between venous congestion measured by right heart catheterization and renal dysfunction in a cohort of 51 patients with HF secondary to pulmonary hypertension. They compared the evolution of right atrial pressure (RAP), cardiac index (CI) and GFR as well as renal blood flow (RBF), measured by 125I-Iothalamate and 131IHippuran clearances. In multivariate analysis, RBF and RAP were independently associated with GFR suggesting that if RBF was the main factor determining GFR, venous congestion itself, characterized by increased RAP was also an independent determinant of GFR [22]. Other studies in larger cohorts of various types of HF have shown similar results with increased CVP consistently associated with impaired renal function and independently related to all-cause mortality [23, 24]. Association between an increased in BNP levels and renal dysfunction was observed in a large cohort of patients with chronic HF. The vascular congestion mechanism with elevated CVP has been shown to induce WRF both in HFrEF and HFpEF. The relationship between GFR and venous congestion is not simple. To understand this complex relationship, it must be remembered that the kidney is an encapsulated organ. Increase of central venous pressure will lead to increase of venous volume then interstitial edema that in turn increases hydrostatic pressure in Bowman’s capsule and decreases GFR [23]. Elevated central venous pressure potentially causes a reduction of RBF and activation of the renin-angiotensin-aldosterone system (RAAS) and the sympathetic nervous system that cause kidney damage and subsequently decreased GFR [16]. Knowing this cascade of events enables to introduce specific treatment such as active depletion but also inhibitors of RAAS, or sympathetic nervous system inhibitors such as beta blockers.
Management of Patients with Primary Right-Sided Heart Failure (with Severe Tricuspid Regurgitation) and Kidney Dysfunction
Determining the Volume and Cardiac Function Status
Different hemodynamic profiles are associated with WRF during decompensated HF: congestion and/or decreased RBF. One of the hurdles for choosing the adequate treatment is the evaluation of intravascular volume in these patients with right heart failure preload-dependency but also with important risk of fluid overload. Fluid overload causes cardiac contraction failure, worsening of tricuspid regurgitation, an increase in ventricular interdependency, a LV dysfunction, and finally an impairment of cardiac output. It seems appropriate to advise monitoring to guide fluid loading or depletion tests [3]. Hemodynamic monitoring such as measurement of CVP and/or cardiac output could be recommended in order to evaluate tolerance and efficiency of therapy. Decongestion strategies based on monitoring of total blood volume and plasma volume measurements at admission by using iodine-131-albumin dilution technique are associated with lower 30-day rates of readmission and 30-day and 365-day mortality but longer lengths of stay than control subjects [25]. The Evaluation Study of Congestive Heart Failure and Pulmonary Artery Catheterization Effectiveness (ESCAPE) [26] study compared a pulmonary artery catheter (PAC)-guide therapy to usual care to determine if PAC monitoring is safe and improves clinical outcomes. PAC use did not significantly affect prognosis during the first 6 months without increase in mortality related to PAC use. Monitoring and optimization of the intravascular volume status seems interesting but should not be done at the expense of patient safety. Less invasive methods such as CVP measurements, measurements of natriuretic peptides and troponins, as well as echocardiography seem interesting in the first steps of treatment.
Active Decongestion
As previously described, congestion causes both aggravation of HF and renal function. Intravenous diuretics are the first-line therapy to treat volume overload in patients with signs of venous congestion. Intravenous loop diuretics (through intermittent bolus or continuous infusion) are recommended for all patients admitted with signs or symptoms of fluid overload to improve symptoms [3]. The Registry Focused on Very Early Presentation and Treatment in Emergency Department of Acute Heart Failure (REALITY-AHF), a prospective, multicenter, observational cohort study, has suggested an association between early treatment with intravenous loop diuretics and lower in-hospital mortality in patients with acute HF [27]. A post-hoc analysis of the 4953 patients of the Acute Heart Failure Global Survey of Standard Treatment (ALARM-HF) cohort found no association between diuretic dosing and short-term mortality [28]. Nevertheless, loop diuretics may be associated with increased morbidity [29] and may lead to deterioration of renal function [30]. Chronic infusion of loop diuretics into animals induces increased activity of the thiazide-sensitive Na+/Cl−-cotransporter (NCC), demonstrated to be an aldosterone-induced protein [30]. Extraction of excess fluid through ultrafiltration has been proposed as an alternative method. Some studies tried to compare the safety and efficacy of ultrafiltration and conventional intravenous diuretic therapy for patients with acute HF and volume overload [31]. A meta-analysis has shown that the weight loss and fluid loss at 48 h was greater in patients who received ultrafiltration compared to diuretics, but the occurrence of WRF (defined as increase in serum creatinine >0.3 mg/dL at 48 h), was similar in the two groups. Actually, no clear recommendation exists regarding the use of ultrafiltration [31]. Moreover, other drugs are currently under investigation to design the optimal diuretic agent or combination of drugs to improve decongestion in acute HF with volume overload. For example, tolvaptan, an oral vasopressin-2 receptor antagonist, added to conventional therapy with loop diuretics achieved more diuresis and relieved dyspnea symptoms in acute HF patients with renal dysfunction [32]. Nevertheless no effect on long-term mortality or HF-related morbidity was observed [33]. Moreover, the Acetazolamide in Decompensated heart failure with Volume OveRload (ADVOR) trial investigates the carbonic anhydrase inhibitor acetazolamide combined with loop diuretic therapy in acute HF with volume overload [34].
Optimization of Arterial Pressure and Cardiac Output
WRF may be secondary to reduced cardiac output and low arterial pressure. Norepinephrine is primarily indicated to restore hemodynamic stability in RV failure [3]. Restoring blood pressure and cardiac blood flow is necessary to improve cerebral, coronary, kidney and other organ perfusion. In ventilated dog models, norepinephrine infusion decreases biventricular filling pressures and increases stroke volume without major increase of the pulmonary vascular resistance [35].
Monitoring cardiac output could justify the introduction of inotropes if low. Dobutamine improves cardiac contractility and output but may reduces blood pressure through its vasodilatory effect. Association of norepinephrine could therefore be necessary. Levosimendan was shown to have an inotropic effect without worsening of myocardial diastolic dysfunction, nor increase of myocardial oxygen consumption. Levosimendan has vasodilator effects in different organs such as the myocardium, lungs, liver and renal medulla and has anti-inflammatory and anti-apoptotic effects [36]. Compared to dobutamine in a specific group of patients with biventricular failure, levosimendan was shown to offer more beneficial effects on TAPSE, 24-h urine output and serum creatinine levels [37]. Levosimendan may also decrease pulmonary arterial pressure in acute conditions [38]. Lastly, phosphodiesterase III inhibitors have positive inotropic effects on the RV without increase of the pulmonary vascular resistance.
Mechanic circulatory support like extracorporeal membrane oxygenation (ECMO) could be proposed to support the RV in certain specific clinical situations such as refractory acute pulmonary embolism. Cardiac pump assistance devices need to be evaluated further for this indication.
Manage Causes of Acute Decompensation of HF
Identification and treatment of triggers of decompensation is necessary to resolve decompensated HF. Sepsis, arrhythmias, RV infarction, valvular disorders, drugs or pulmonary embolism should be sought after and treated. Pulmonary hypertension secondary to cardiac or pulmonary disease may also increase RV failure and congestive signs. Alveolar hypoxia, hypercapnia, hypothermia and acidosis promote pulmonary artery vasoconstriction and increase RV overload. Oxygen therapy with or without non-invasive ventilation to decrease hypercapnia and optimize arterial oxygen saturation > 90% could be proposed [3]. Positive pressure ventilation may increase RV overload but decreases LV preload. However, in the case of decompensated HF, it is associated with increased myocardial performance and reduction of pulmonary hypertension.
Increase of tricuspid regurgitation is a common cause of decompensation in chronic HF with right ventricular failure. In a retrospectively study of 5223 patients, it was associated with poor prognosis, independently from age, biventricular systolic function, RV size, and dilation of the inferior vena cava [39]. These data were confirmed by another study suggesting a beneficial effect of more aggressive approaches toward tricuspid repair or replacement [40].
Prevention of Renal Dysfunction in Primary Right Sided Heart Failure
Prevention of renal dysfunction in primary right-sided HF overlaps with the treatment of chronic HF. Current guidelines for patients with chronic HF recommend the use of beta blockers, angiotensin-converting enzyme (ACE) inhibitors, mineralocorticoid receptor antagonists (MRA) and diuretics [4]. Most of these treatments, such as ACE inhibitors and MRA, may induce a decline in GFR. However, this reduction in GFR is usually small and should not lead to treatment discontinuation [4]. In a recent meta-analysis, RAAS inhibitors were shown to induce renal dysfunction in both HFrEF and HFpEF during the acute phase [41]. However, in contrast to patients with HFrEF where the mortality increase with WRF is small, HFpEF patients with RAAS inhibitor–induced WRF have an increased mortality risk, without experiencing improved outcomes with RAAS inhibition. More studies are necessary but the most important conclusion should be that careful assessment of eGFR during RAAS inhibitor treatment is essential [42]. This also stays true when RAAS inhibitors are prescribed to patients with HFpEF [41].
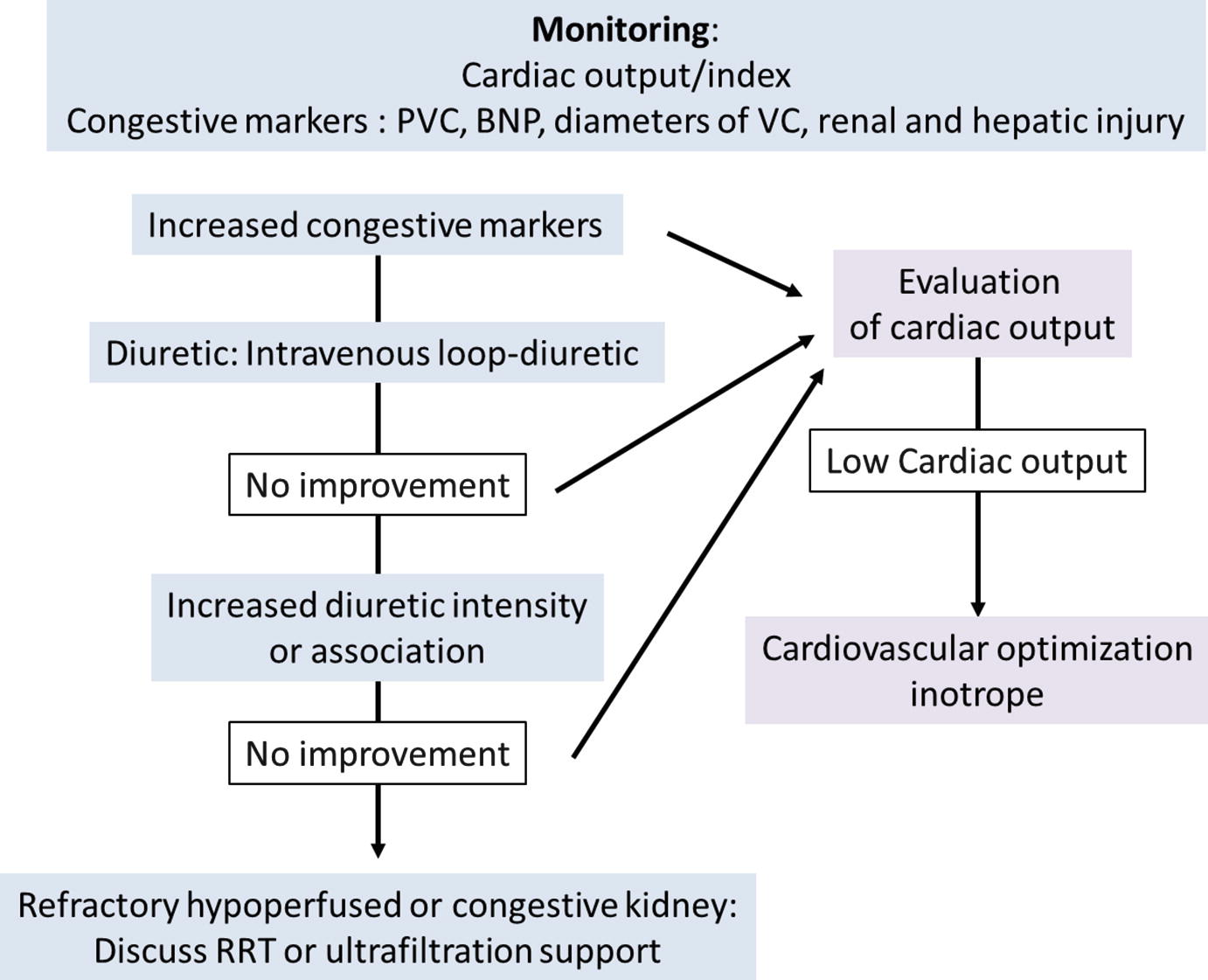
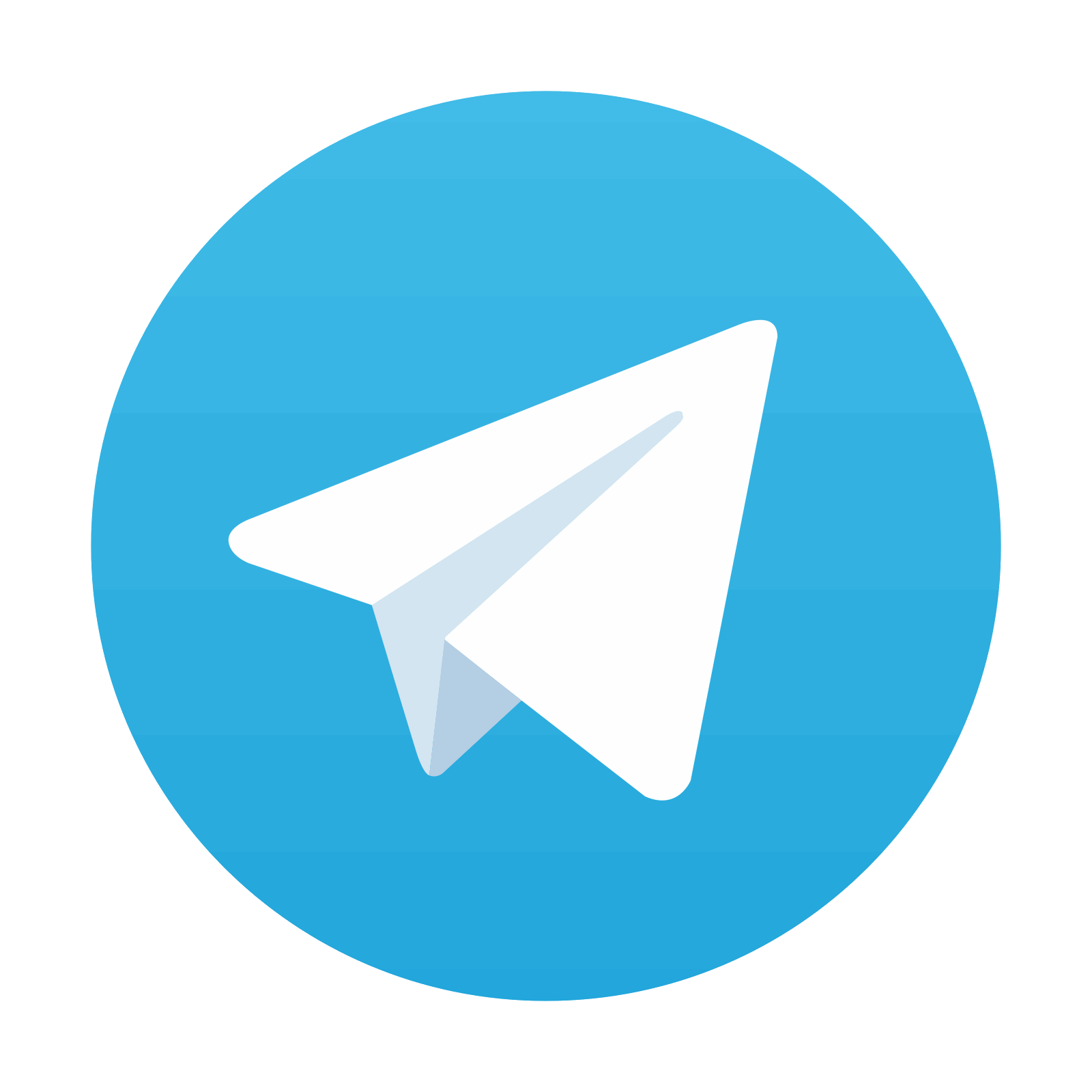
Stay updated, free articles. Join our Telegram channel

Full access? Get Clinical Tree
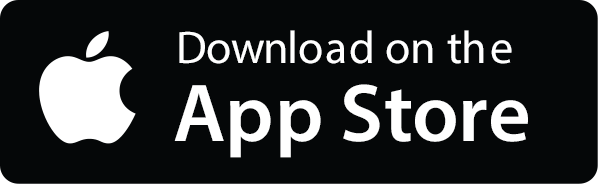
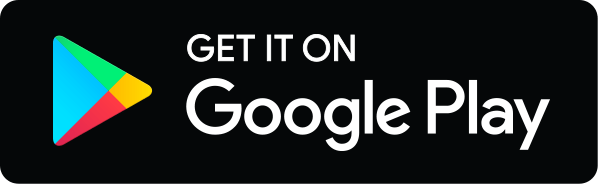