, Germaine Cornelissen2 and Franz Halberg2
(1)
Department of Chronomics & Gerontology, Tokyo Women’s Medical University Medical Center East, Arakawa-ku, Tokyo, Japan
(2)
Halberg Chronobiology Center, University of Minnesota, Minneapolis, MN, USA
Abstract
Around and in living matter, a system of transdisciplinary cycles with common average periods is being quantified with point and interval estimates of parameters. The cycles’ characteristics constitute useful information once their uncertainties are assessed. The cycles in space climate are critical in discussing global warming. The cycles’ periods are described as congruent when their CIs (95 % confidence intervals) overlie or overlap and the amplitudes’ CIs’ lower limits are positive. Some congruent cycles in organisms, counterparts of the environmental day and the seasons, relate to electromagnetic radiation in the visible domain; these are the usually environmentally synchronized socio-photo-thermoperiodisms (photics). The biosphere also resonates with or is pulled or driven by nonstationary, environmental nonphotic cycles (nonphotics) – particle emissions from the sun and the wider cosmos, cosmoheliogeomagnetics, ultraviolet flux, gravitation, acoustics, and others.
Nonphotics, a set of in part transdisciplinarily novel spectral components, can be intermittent; when present, they coexist and compete with signatures of photic cycles, monitored, e.g., in blood pressure (BP) and heart rate (HR) for vascular disease detection and prevention. Nonphotics of, e.g., about (~) 1 week (circaseptans), ~17 months (transyears), characterize mood and performance, modulate, and sometimes override society’s (photic) schedules, even in dying suddenly unintentionally or by one’s own will or at the hand of others. Nonphotics persist but are damped in physiology or in terrorism when their environmental counterpart is not detected.
Biospheric monitoring complements the records from purely physical tools for surveilling the variable sun, validating in the biosphere the reality of intermittent, aeolian environmental spectral components that can be more consistent than their physical counterparts once they are coded in genes, indicating the relevant associations of space weather with human health and ecology. We invite readers, as a dividend from self-surveillance for preventive health care, to monitor, on themselves, solar variability, a transdisciplinary gauge of individuals’ and societies’ health and disease, including violence, notably terrorism.
Keywords
AeolianChronomicsCongruenceTransdisciplinary21.1 Introduction
These complementary materials extend the scope of a presentation on “What is life?” [1], prompted, in the wake of Erwin Schrödinger’s book of the same title [2] by Schrödinger’s colleague, the physicist Arthur March [3], to whose memory this chapter is dedicated. In 1946, we answered “What is life?” with “periodic waves,” and we do so again in 2007 [4] with observations from the senior author’s (FH) lifetime and that of many others. Further background to this summary, presented at the Hideki Yukawa 100-year Memorial Seminar “What is life?,” at the Yukawa Institute of Theoretical Physics in Kyoto, Japan, October 15–20, 2007, is found in cartography, clinical and basic, in an invited presentation, under the title “60 years of translational chronobiology,” to open a symposium on “circadian rhythms from animals to humans” at Experimental Biology 2007 in Washington DC on April 29, 2007 [4], earlier at the European Geosciences Union General Assembly in Vienna, April 15–20, 2007 [5] and later at the International Academy of Science symposium on “Natural cataclysms and global problems of modern civilization,” Baku, Azerbaijan, September 24–27, 2007 [4], and at the VII International Crimean Conference “Cosmos and biosphere,” Sudak, Ukraine, October 1–6, 2007 [6].
21.2 Preamble
Suitbert Ertel [7] summarized a schism between East and West that remains pertinent today:
At an Annual Meeting of the American Meteorological Society, 1926, an American participant delivered a paper written by Alexander L. Chizhevsky, a Russian scholar. Its bombastic title (“The influence of cosmic factors upon the behavior of organized human masses as well as upon the universal historical process”) appeared laughable. The author claimed that occurrences of social unrest, rebellions, upheavals, revolutions are significantly correlated with solar activity, i.e., with the ups and downs of magnetic turbulence of the sun….
Chizhevsky was imprisoned by the KGB from 1942–1958. His claim was oppressed; in Western countries, it was ridiculed or simply ignored. There are exceptions. At least one historian, William S. Langer, President of the American Historical Association and outstanding figure in his field, suggested that “We may … be on the threshold of a new era when the historian will have to think … even in cosmic terms” [7].
As of 2007, there are indeed many exceptions to Ertel’s [7] claim that Western science dismisses Chizhevsky’s theories (Astbury, personal communication; [8–42]). In post-Soviet Russia, Chizhevsky is revered, with a most recent meeting in Sudak, Crimea, Ukraine, dedicated to the 110th anniversary of his birth [43].
In conclusion, his review with meta-analyses in support of Chizhevsky’s theses concerning not only “infections” of the mind but also epidemics ([44–47]; cf. [7], [14]) notes that “researchers in chronobiology/chronomedicine and in biogeomagnetics … are not far from setting the stage.”
Negative findings, however, have been made in the West with impeccable methods on large databases (Table 21.1) albeit over hardly more than a single sunspot cycle [48, 49]. The chronobiologic stage was perhaps set:
Table 21.1
The problem of heliobiology in the “West”: negative results on millions of people in 2 North American papersa
1. From Feinleib et al. [48]: | ||
1964–1966 (3 years) daily; 1964–1971 (8 years) monthly | ||
Daily deaths | Estimated totals 1964–1971 (8 years) | |
All | 14,493,120 | |
Coronary heart disease | 4,383,000 | |
Stroke | 1,577,880 | |
2. From Lipa et al. [49]: | ||
1962–1966 (5 years) = 1826 days | ||
Coronary heart disease | Stroke | |
Total (1962–1966) | 2,750,880 | 1,002,246 |
When temporal and geographic differences could be documented in several mortality endpoints investigated (Table 21.2, Fig. 21.1a, b) [50, 51]
Table 21.2
Differences among three consecutive solar cycles found by fit of a 10.5-year cosine curve to daily mortality from myocardial infarction in Minnesotaa
Cycle N
P (A = 0)
Average ± SE (cases/day)
Amplitude (A) (95% CI) (cases/day)
Relative amplitude
Phase of maximum (95% CI) (degrees)b
1
0.006
12.0 ± 0.1
0.36 (0.18; 0.53)
0.030
−135 (−105; −164)
2
<0.001
12.4 ± 0.1
0.76 (0.55; 0.96)
0.061
−145 (−132; −158)
3
0.830
12.1 ± 0.1
0.07 ( )
0.006
−144 ( )
Test of equality of parameters
Parameter(s)
ndf
F
P
Average
(2, 20)
11.296
0.0005
Amplitude (A)
(2, 20)
12.820
0.0003
Phase of maximum (ϕ)
(2, 20)
0.231
0.7954
(A,ϕ)
(4, 20)
6.762
0.0013
(M,A,ϕ)
(6, 20)
9.586
<0.0001
Fig. 21.1
(a) Resolution of a controversy by the recognition of time- and site-specific changes that in the light of accumulating evidence can be anticipated insofar as such variability in results is repeatedly documented. The analogy of blood pressure measurement over a single circadian cycle comes to mind: such a spotcheck may lead to a lifetime of unneeded treatment when, e.g., in several repeated visits to a care provider’s office, a hypertensive patient may happen to be caught on several measurements (notably in the afternoon) with occasional normotensive values and hence will not be treated until a stroke occurs. By the same token, a mostly normotensive patient may be excited, the spotchecks may show “hypertension,” and the patient may be treated unnecessarily for a lifetime. Furthermore, an individual with blood pressure overswinging limited to the normal range may not be recognized as being at a very high risk of stroke by limitation to a single cycle or again he or she may be misdiagnosed and treated unnecessarily. Thus, studies that cover a single solar cycle may not be conclusive and may miss a relation that can be documented as being off and on aeolian elsewhere or over 2 of 3 solar cycles (in Minnesota, USA). Taking evidence over a single solar cycle is equivalent to taking the pulse for one second. The graph also shows geographic differences in the same solar cycle, as does Fig. 21.1b (© Halberg Chronobiology Center) (b) This figure shows initial limited data now further extended to additional geographic and temporal differences for the case of sudden cardiac death in the form of rhythmic patterns with different frequencies characterizing this condition at different times and in different geographic locations. A curtain of uncertainty, because of limited available data, hides any time- and geographic (geomagnetic or dip-magnetic) site specificity of various spectral aspects of sudden cardiac death. Thus, we find a transyear in Minnesota with a cis-half year (cY/2) and both a calendar year and a transyear in Arkansas and the Czech Republic: at the latter site a cis-half year, corresponding in length to an also-transient component of hard solar flares (Rieger et al., 1984; Ballester et al., 2002) is detected after but not before 1999 (Halberg et al., 2005). In 1998, Peter V. Minorsky reevaluated latitudinal differences in coconut palms’ foliar spiral direction (FSD). He hypothesized that latitude-dependent biases in FSD, a non-Mendelian trait, will be associated with a temporally varying component of the earth’s magnetic field. Earth currents, which are measurable in trees, bias the difference of auxin (or auxin transport proteins) in young embryos, such that left-handed palm trees (LHP) are produced preferentially in the Northern Hemisphere and right-handed ones (RHP) in the Southern Hemisphere. He built his observations on a previously collected database by Davis and Davis, revealing that the ratio of LHP and RHP/total was better correlated with magnetic (dip) latitude than with geographic or geomagnetic (centered dipole) latitude. Minorsky and Bronstein (2005) referred to this classical case of morphological antisymmetry in which dextral and sinistral forms are not inherited and are equally common within a species, proposing that Pc1-induced earth currents may bias the diffusion of morphogens in coconut palm embryos, thereby giving rise in asymmetries of FSD. Whether other geographic differences in sudden cardiac death may also relate to any magnetic latitude deserves scrutiny. It is noteworthy in any event that cardiac arrhythmias can also transiently reveal a transyear or a cis-half year, each in a different solar Schwabe cycle stage (Halberg et al. 2006a) (© Halberg Chronobiology Center)
When the presence vs. absence of an about-7-day (circaseptan) component in solar activity was accompanied by an amplification vs. damping of a persistent circaseptan rhythm of HR in RBS, a clinically healthy subject documented by about five daily self-measurements made for several decades, in keeping with other decade-long series from four other individuals investigated (Fig. 21.2a, b) [52, 53]
Fig. 21.2
(a) Average decrease in circaseptan-to-circadian amplitude ratio of heart rate for five subjects (right) is shown for one of the subjects (middle) during a span (V) when no solar circaseptan is detected in Walsh spectra vs. four other spans (I–IV) during which a component near 1 cycle in 6–8 days is detected in Walsh spectra (Vernova et al., 1983). The actual Walsh spectra of the rate of change in sunspot area during spans I (top) and V (bottom) are shown on the left. In data of subject RS (middle), amplitude ratios log-transformed to render the distribution closer to a normal one were computed during consecutive nonoverlapping adjacent 7-day intervals for comparison by Student’s t-test. All data collected during and for one week after a trip associated with a transmeridian flight involving at least four time zones were excluded, as were data collected during illness (usually no more than a cold or flu). Even though nonoverlapping adjacent weekly intervals served to compute the circaseptan-to-circadian amplitude ratios, it may be argued that some lingering serial correlation may remain. The smaller amplitude ratio persists (P < 0.001) when the Student’s t-test is repeated using weekly intervals separated by 2-day gaps to reduce any serial correlation (© Halberg Chronobiology Center). (b) Assigning the average circaseptan amplitude of heart rate in each span for each subject (expressed as a percentage of the mean computed across all spans for that subject) to the midpoint of each span, the fit of an 11.6-year cosine curve to the total of 15 values pooled over the five subjects is statistically significant (P = 0.018 for the circaseptan amplitude and P = 0.016 for the circaseptan-to-circadian amplitude ratio), with maxima occurring around May 1971 and May 1970, respectively. A statistically significant 11.6-year component is also found for the circadian (rhythm-adjusted) mean (P = 0.031) and for the circadian amplitude (P = 0.032) of heart rate, both expressed as a percentage of each individual’s mean (P = 0.031) (across all available spectra). Their respective peak times occur around March 1970 and December 1974 (© Halberg Chronobiology Center)
When the solar wind speed lost an about 17-month broad band transyear cycle and when, with a transyear lag, the same transyear was partly lost and the remainder damped in the systolic blood pressure of an elderly man (Fig. 21.3) [54].
Fig. 21.3
The resonance with solar activity is extended to a wobbly transyear with a period statistically significantly longer than a year in the systolic blood pressure (BP) of a 72-year-old man (FH) who automatically measured his BP and HR at about 30-min intervals, with gaps, for 16 years. Gliding spectral windows with trial periods (τs) between 2.0 and 0.4 y computed for 8y overlapping data intervals displaced in 1-month increments show no seasonal oscillations, as in some other elderly individuals. Far-transyears (FTY, with periods such as 1.2 years ≤ [τ – CI] < [τ + CI] < 1.9 years, where CI is the 95% confidence interval) characterize FH’s data. A spectral band is found for systolic (S) BP congruent with one in the speed of ionized particles that stream by satellites: the solar wind (SW). An abrupt disappearance of a FTY in SW speed (SWS) is followed (with an FTY lag due to one more resonating cycle?) by the disappearance of part of this band in SBP that was probably driven by SW. The persistence of part of the FTY band in FH’s SBP, in keeping with other evidence of a BP FTY free-running from that of the SW, demonstrates an evolutionary acquisition of a solar FTY oscillation that may reflect some of the sun’s dynamics for billions of years past. The circumstance that the remaining part of the transyear band is damped is in keeping with the assumption that it was also driven by SW. Only minor coincident changes are seen in diastolic BP (DBP) or heart rate (HR) (bottom 2 sections). Aeolian rhythms in gliding spectra of SWS and SBP change in frequency (smoothly [A] or abruptly [B,C,D], bifurcating [D,F], and rejoining [G], they also change in amplitude (B)) (up to disappearing [C,E] and reappearing). During a nearly 16-year span, there are no consistent components with a period averaging precisely 1 year in the three physiologic variables, probably an effect of advancing age. The finding of FTY patterns, along with a cycle of about 5 months, i.e., a cis-half year in sudden cardiac death, in some but not all geographic locations (Fig. 21.1b) shows the importance of the earth in modulating solar effects. Chance and post hoc ergo propter hoc reasoning cannot be ruled out, but the circadian story is extended to infradians, beyond the fact, underlining their importance, that both components can account for the difference between life and death (Halberg et al. 54) (© Halberg Chronobiology Center)
In this presentation and elsewhere, the likelihood that our measurable time structures – chronomes (Figs. 21.4 and 21.5a, b) – respond to space weather reopens an old field of investigations [7, 14, 43–45, 47–49], perhaps pertinent to setting the course of history, as Chizhevsky [44] put it insofar as the hard-to-define “good” and “bad,” such as religious motivation and terrorism, are involved. With inferential statistics implemented by computers on time series that were not available in the precomputer, pre-satellite, pre-molecular biology era, methods now allow us to follow a tree of life [55] with a figurative microscopy and telescopy in time. We can say that nothing in transdisciplinary science can be fully understood without a record of the broad spectrum of cycles in the cosmos that constitutes a quantifiable (variable but not random) spectrum (Fig. 21.5b) and thus is a hardly dispensable background to any experiment or investigation in time, replacing fictitious spotcheck-based “baselines.” Accordingly, we reconsider a statement attributed to Max Planck that the experiment carried out critically by a specialized researcher must yield the same result at each time and in each place. With respect to “experiments of nature,” we follow, in BIOCOS, the reasons underlying the need for studying “the influence of the sun’s emanations” on earth by an international effort given by Sir Edward Appleton, the discoverer of the ionosphere, in introducing a book by Harlan True Stetson [56]:




Fig. 21.4
Chronome: time structure consisting of multifrequency rhythms covering frequencies over 18 orders of magnitude, elements of deterministic and other chaos, trends in chaotic and rhythmic endpoints, and any other, as-yet unresolved variability (© Halberg Chronobiology Center)


Fig. 21.5
(a) From the mistaken assumption of a master clock serving regulation for constancy, we turn to recognition of an integrative internal-external collateral hierarchy for physiological coordination. Homeostasis postulates that physiological processes remain largely within certain ranges in health and seeks departures from such “normal values” as baselines to diagnose overt disease. Thereby, variability within the normal range, however, is often dealt with as if it were narrow, random, or trivial, the body striving for at least a relative “constancy.” The alternative, rather than complement, to this status quo, on the left of this figure, is learning about the rules of rhythmic and chaotic variations that take place within the “usual value” ranges that led to the postulation of a “biological clock” that would enable the body to keep track of time. By removal and replacement experiments, a “clock” was located first in the adrenal, shown to be responsible for some but not for other circadians that persisted after brain ablation. The fact that single cells and even bacteria are genetically coded for a spectrum of rhythmic variation indicates that the concept of “clock” needs extension. The concept of figurative calendars followed, aligned with clocks and further a biological week, a 2-week cycle, one of five and another of 6 months, and many more cycles, including some of ~1, ~2, and ~5 decades’ lengths, and other rules were found in variability, such as deterministic chaos and the long-known trends, some of which may turn out to be cycles (see Fig. 21.4). When the giant alga Acetabularia, a prominent model of a circadian “clock,” is placed into continuous light, its spectrum of electrical activity recorded by Hellmuth Broda (Cornélissen et al., 1986) reveals the largest amplitude for a component of about 1 week rather than of 1 day. When over a decade of studies on this alga are pooled, an about 10-year cycle emerges in the data set as a whole. The alignment of spectral components and chaos and trends in and around us has also begun. Long-term longitudinal, but not yet entire, lifetime monitoring of critical variables complements current linked cross-sectional (hybrid) reference values required for preventive health and environmental care. Changes occurring within the usual value range are resolvable by chronomics as longer and still longer cycles, with a (predictable multifrequency) rhythmic element that allows us to measure the dynamics of everyday life, in order to obtain, e.g., warnings before the fait accompli of disease, so that prophylactic measures can be instituted in a timely way, to detect heretofore largely undetected or unquantified environmental effects or internal changes, which are silent not only to the person involved at high risk but also to conventional health care and science. These vascular variability disorders are detected by cost-free analyses in the BIOCOS project (corne001@umn.edu). On the top right, an abstract sketch is an often-used snapshot reminiscent of the more complex sector structure of the interplanetary magnetic field, shown by three arrows in opposite directions. The fourth arrow is covered by another circle showing solar flares. The parameters of the solar wind and of solar flares are much more variable than visualized when first described. The three circles below represent a chronome (cf. Fig. 21.4); the right-hand section as a whole symbolizes associations of helio- and geomagnetic variability, not only with myocardial infarctions and strokes but also with suicide and terrorism that are accumulating and are just the tip of the iceberg, with a profound effect of magnetism (recognized by Gilbert in 1600), apparent in the human ECG, notably in the auroral region (Otsuka et al. 2001). In external-internal interactions, a broad spectrum of rhythms (in both the environment and in living matter) organizes deterministic and other chaos and trends. Trends pursued long enough may become low-frequency cycles, information that may serve, e.g., for the detection of any risk elevation and for the taking of timely action (© Halberg Chronobiology Center). (b) The chronomes of variables in us, which came about as a function of chronomes of variables around us and were eventually coded genetically, await further exploration in health care and broader science, including focus on mechanisms underlying adaptive, integrative, and cultural evolution toward a chrononoosphere, topics of chronobiology broadly (© Halberg Chronobiology Center)
First, the earth is round, not flat; hence, solar radiations impinge unequally in different regions.
Second, the earth rotates and interesting solar features may occur at any time; hence, observers are needed at different terrestrial longitudes so that no effects are missed.
Third, the earth is a large magnet in its own right, so that electrical particles from the sun are constrained to travel in curved tracks that may even have effects on the side of the earth’s atmosphere further from the sun (at night). Magnetic qualities are very different in different locations on earth, and hence they modify solar effects with unequal intensity at different terrestrial latitudes.
With the use of these three points, Sir Edward was a master in inviting international cooperation to find information critical to communication science as well as for the fields of bioheliogeomagnetics or what has also been dubbed chronoastrobiology [15]. In part for the same fundamental reasons, there are geographic and temporal differences among the signatures of photic vs. nonphotic phenomena, e.g., in incidence patterns of natality and mortality as well as of events in between.
21.3 General Background
Part of a vast, diverse, relatively recent literature on cycles in us and in the cosmos attempts to approach the open system called an organism, as a dynamo and a magnet, living on the earth, a larger magnet [57], in the atmosphere of the sun [58], within galaxies and universes, with magnetic storms causing blackouts in cities [59] and relating to as yet irreversible ones in human hearts. Organisms are open to their environment magnetically, electrically, mechanically, and in many other ways yet to be elucidated. We do not know the extent to which they resonate with or depend upon sets of processes near and far that are not consciously seen, heard, tasted, touched, or otherwise knowingly registered. We are, willy-nilly, an integral, continuously informed part of the environment, by which we also happen to be energized. What we can do to learn more about what Frank A. Brown Jr. [60] called subtle influences is to remove and preferably also replace parts of our body in the laboratory or, when this is not possible, let the cosmos do the subtracting and adding (i.e., of a spectral component) to observe what happens in ourselves. Herein, we succinctly summarize uses of circadian mapping (Fig. 21.6a) as a precedent for the merits of concern about photics in health care; we then turn to maps about photics (Fig. 21.6b) and nonphotics in and around us (Fig. 21.6c–g), to what we learned about methods for their study, about remaining problems, and about their relevance thus far, notably with respect to violence.














Fig. 21.6
(a) Illustrative circadian phase map suggesting a synchronization of several aspects of human physiological and psychological performance with differences in timing. Summary of authors’ and others’ published data (Halberg, 1969) (© Halberg Chronobiology Center). (b) Human circannual rhythms in urine and blood, yielding endpoints for the assessment of the risk of developing several diseases of our civilization (Halberg, 1983). Note, however, many indications of timing without horizontal lines that indicate uncertainties computed as 95% confidence intervals. When circannual phases are followed for decades in the same individual, they can reveal very great variability, possibly resulting from beats between photic and nonphotic, calendar year vs. cis- and transyear mechanisms (© Halberg Chronobiology Center). (c) Start of a partial circasemiannual acrophase map (© Halberg Chronobiology Center). (d) Near-transyears around [1–3] and in [4–9] organism(s). It is indispensable to specify the calendar span and thereby also the length of a time series upon which the resolution of a (set of) spectral component(s) (c) with period(s) (τ) rests. As to mechanisms, it seems pertinent that the only endocrine record covering 15 years of observation of an adult’s 17-ketosteroid excretion reveals globally no yearly component, only a near- and two far-transyears, the latter not shown. The finding of physical and biological near-transyears, defined by 1.00 year (y) < τ < 1.20 y, is mutually reinforcing. These near-transyears in us, acquired by evolution, reinforce the reality of those around us from which they likely derive. We all stacked data in the past over a year and other periods such as a day; yet whenever possible, we should get an estimate of the period involved before stacking! This was a sine qua non well over 50 years ago for circadians and has become one for circannuals and in particular for aeolians, such as the near-transyear, here depicted as calendar-time-location- and series-length-dependent near-transyears (Halberg et al. 2004a and b). It remains to be scrutinized whether near-transyears are separate components and probably not as sidelobes of circannual variation (not stemming from amplitude and/or phase modulation by components with a lower frequency) (© Halberg Chronobiology Center). (e) Phase map of ~20-year cycles. Note relative in-phaseness shown by overlapping 95% confidence intervals between criminality and religious motivation, among other variables: a transdisciplinary challenge (© Halberg Chronobiology Center). (f) A phase map of about 10-year cycles is illustrated here. Since studies are often carried out over relatively short spans, it is more important to map long-term cycles, so that the time horizon thus provided may serve to guide the design of shorter-term studies. This is one of the immediately useful chronomics that maps the broad time structures in biota and aligns them with environmental chronomes (© Halberg Chronobiology Center). (g) Population studies also point to the presence of ~50-year cycles in hardly trivial “diseases” of society, such as homicides and wars, almost certainly involving the human brain, as does the ~20-year cycle in religious motivation (© Halberg Chronobiology Center)
21.4 Remove and Replace = Subtraction and Addition in the Study of Circadian Rhythms
Removing a gland was a time-honored approach to finding out what the removed gland does, by looking for deficiencies or other alterations and seeing further what replacement does. This was how endocrinology was reportedly founded by Arnold Adolph Berthold [61, 62].1 Two cockerels with their testicles removed remained capons (subtraction); two other castrated cockerels that received testicular transplants (remove, subtraction; replace, addition) developed secondary sexual characteristics, and the concept of substitution therapy evolved.
In 1950, Franz Halberg found, as others did before him, very large variations along the 24-hour scale in counts of cells staining with a pink dye (eosin): the eosinophil cells [63]. The eosinophil counts were depressed by certain adrenocortical hormones. In the euphoria of the finding that the adrenal hormone made lame people walk again, adrenal glands were removed in human research looking for underlying mechanisms. The opportunity revealed that a cycle in eosinophil count was lost in patients without adrenals, as it was lost in adrenalectomized mice [64]. The finding held for the circadian rhythm of eosinophil cell counts; in the circadian rhythm of serum iron, there were only changes of the amplitude, A, and the acrophase, ϕ, after adrenalectomy, but no abolition (Fig. 21.7a) (bottom and top, respectively). Some decades later, the ablation of the suprachiasmatic nuclei abolished the circadian rhythm in water drinking, but not that in alcohol drinking or in DNA labeling in the digestive tract (Fig. 21.7b) or core temperature (Fig. 21.7c).














Fig. 21.7
(a) Chronobiologic self-experimentation extended to groups of medical students and staff served to show that the adrenal glands are an important source of circadian rhythmicity. For instance, a circadian component of variation in the circulating eosinophil count could not be demonstrated for patients with adrenocortical insufficiency (I bottom), whereas in healthy people with either restricted or unrestricted activity (I top), this rhythmicity was not only demonstrable but also amplified by enhanced motor activity. Whereas for patients with Addison’s disease a circadian rhythm in eosinophil counts could not be statistically validated (polar cosinor displays both), circadians persisted, however, for serum iron (polar plots on top, not covering the pole, i.e., center) (P < 0.001). Self-experimentation then leads to studies in the laboratory. Whereas in intact rats a circadian rhythm could invariably be detected for several steroidal hormones (pregnenolone, corticosterone, and dehydroepiandrosterone), in adrenalectomized and orchidectomized rats, a circadian rhythm remained demonstrable for plasma pregnenolone and for brain dehydroepiandrosterone (II). Circadians of adrenal corticosterone also persisted on the day after stepwise cerebral ablation (III) (© Halberg Chronobiology Center). (b) Circadian rhythm alteration rather than obliteration after lesioning of suprachiasmatic nuclei (SCN). By eyeballing alone of Section IIA, the circadian rhythm in telemetered core temperature, each measurement shown by a dot, is clearly seen in data from a sham-operated control on the left and seems to be lost in the rat with a SCN lesion on the right of this section, whether one examines squeezed values in the top row or stretched values in the second row (of dots). A circadian rhythm in temperature for individual animals is also displayed in Section I top, with a smaller within-day change of lesioned animals (IB) as compared to controls (IA). This finding is also seen after averaging in Section IIB (bottom). Time microscopy, in section IIC, apart from quantifying the rhythm by cosinor, reveals, by a shorter arrow, a great amplitude lowering after a bilateral SCN ablation and a phase advancement seen as an earlier vector. Section IIC also validates the persisting rhythm by the nonoverlap of the center (or pole) of the graph, by the error ellipse representing a 95% confidence region: the removal of the SCN is compatible with the persistence of a statistically highly significant circadian rhythm in core temperature quantified with its parameters and their uncertainties, after histologically validated bilateral SCN ablation. When the ablation unintentionally, as discovered at postmortem, was unilateral (U), the circadian amplitude was enhanced (Section I, bottom), a finding suggesting a subtractive coupling between the two SCN. Section III is in keeping with the speculation of an effect by lunar factors upon the “free-running” (or rather lunar?) period of about 24.8 hours found in controls or unilaterally ablated animals at that light intensity. If this should be in part a lunar effect, it is lost in animals subjected to bilateral (B) suprachiasmatic lesions and apparently tightened by unilateral (U) lesions, a possibility requiring further experimental scrutiny (© Halberg Chronobiology Center). (c) Persistent, albeit altered, circadian rhythmicity of 3H-TdR incorporation into DNA of different organs and of mitotic index of corneal epithelium of BD2F1 female mice after bilateral lesioning of suprachiasmatic nuclei (SCN) (rows 1–3); persistence of altered rhythm seen in ethanol (row 4; left) but not water (row 4; right) consumption after bilateral lesioning. Data from J.N. Pasley (Pasley et al., 1987). The SCN coordinates a collateral hierarchy that can be quantified in terms of amplitude and phase: the major effect of bilateral SCN ablation is thus far invariably, comparably to the behavior of core temperature, an advance in phase for the SCN-lesioned (L) animals in 8 cases out of 8, with a reduction in amplitude, except for DNA labeling in the stomach and colon, which may respond to food directly rather than by the SCN, Section III. Section VI shows a microscopic phase and amplitude chart summarizing the finding in the other sections (I–V, VII, and VIII). This chart extends the scope of the SCN lesson learned from studies of core temperature (Fig. 21.7b) to a number of other variables studied as marker rhythms: rather than being a master clock leading to the loss of all rhythms when ablated bilaterally, the SCN is compatible with the rhythm’s persistence in several of the variables investigated, except for a few important ones like locomotor activity, water drinking, and blood pressure. A subjective time-macroscopic interpretation-based impression that led to the master clock illusion is replaced by the objective quantification of a mechanism for period, phase, and amplitude in a network with a collateral rather than up-down hierarchy (© Halberg Chronobiology Center). (d) Macroscopic circadian desynchronization in mice after bilateral optic enucleation (dashed line connecting dots) visualizing the need for objective inferential statistical time-microscopic analyses added in sections B and C of Fig. 21.7e. While sham-operated controls (solid line connecting open circles) show a daily peak at or close to the vertical 20:30 lines, peak temperatures of blind animals already on day 6 seem to diverge, rising while those of the other group are falling. A graph of the original finding of this separation of the two groups and the decision based thereon to continue measurement every 4 hours around the clock was interpreted as “paranoia.” (At the time, in the precomputer era, the provision of a periodogram on desk calculators took a week, and its checking another week. Indeed, today the approach with a moving fit of a 24-hour cosine curve, rapidly implemented on a computer, is preferred to inspection that has to eliminate “misbehaving rogues” and remains subjective.) On the average, on top, peaks in temperature of the blinded group occur earlier and earlier, but there are uncertainties in such eyeballing. A transient antiphase at 22 to 23 days after blinding is readily seen. If, around that stage after blinding, 2-timepoint checks are carried out on the 2 groups, opposite results can be obtained on mice with and without eyes and later when they are again in phase, 2-timepoint checks show no difference, a puzzle readily resolved by an objective quantification of the rhythm characteristics. The need for this microscopy in time becomes obvious, notably if an inference is desired as soon as possible with an estimate of uncertainty. (Circadian desynchronization also characterizes congenitally blind ZRD mice) (© Halberg Chronobiology Center). (e) Top, section I: Desynchronization of circadian rhythm in core temperature of mice after blinding, seen time-macroscopically in IA (much better in Fig. 21.7d), here leads, in IB, to time microscopy with a chronobiologic serial section showing a different time course of the core temperature acrophases, ϕ, with early separation of the two groups by nonoverlapping 95% confidence intervals ϕ in IC, to a summary of individual periodograms that form two separate distributions, and in ID to time relations among three variables in a 24-hour synchronized (top) or free-running (bottom) system (of mice, left, and of a human, right). Section II shows a spontaneous (α) rhythm in circulating corticosterone of mice in antiphase with the slope of an in vitro response rhythm to ACTH, a reactive (β) rhythm. The components of the chronome (time structure) are internally coordinated through feedsidewards in a network of rhythms that are more or less spontaneous (α), others primarily reactive (β) or modulatory at a single mapped frequency, such as a circadian (γ), IIC and IID, or at multiply mapped (δ) frequencies, IIE (© Halberg Chronobiology Center). (f) Susceptibility rhythm to ouabain in mice of two stocks. Twenty (∓1) male mice (about 5 months of age)/timepoint/stock. Note daily change in mortality from 15% to 75% in D8 mice by comparison to change from 30% to 65% in C mice. Note also greater susceptibility of C mice (given smaller dose). Objective quantification is provided in the cosinor summary at the bottom (© Halberg Chronobiology Center). (g) As compared to patients with an acceptable variability of blood pressure and heart rate (top left) who have 4% cardiovascular morbidity after 6 years, the presence of a single vascular variability disorder (VVD) such as DHRV or EPP alone (top middle) greatly increases the risk, even more so when both conditions coexist (top right) to become a vascular variability syndrome, VVS; the added diagnosis of a circadian blood pressure overswing, CHAT (bottom), further increases risk. VVDs or VVSs can constitute prehypertension (Watanabe et al., 1997), in the absence of an elevation of the average of around-the-clock measures of blood pressure, while they can also complicate a high blood pressure, i.e., MESOR-hypertension; the latter, because of (always to be checked) variability, is also a VVD in its own right (© Halberg Chronobiology Center)
The team of Jerome W. Conn (of Conn syndrome fame) confirmed the finding in adrenocortical insufficiency and showed in addition that replacement with a single large dose at the right time but not with divided smaller doses of oral cortisone reinduced the rhythm [65]. The approach by “remove” (subtraction) was thus again complemented by “replace” (addition). The approach by subtraction was extended in the earliest 1950s beyond gland removal to study other mechanisms of the eosinophil rhythm. When the eyes, receptors of a synchronizing lighting cycle, were “subtracted” by surgery or genetics (in ZRD mice born blind), a previously 24-hour synchronized cycle, while it did not disappear, was free-running from the 24-hour routine, with a built-in period near 1-day length, but not of precisely 24 hours (Fig. 21.7d and e). A circadian desynchronization was already documented by periodograms (that took a week to compute on a desk calculator and another week to check) and were the topic of a discussion with Julius Bartels in 1953, to indicate that his harmonic dial could also be used flexibly for fitting anticipated desynchronized environmental cycles and not necessarily a precise 24-hour day [66].
“Circadian” was coined – in the early 1950s [67] and endorsed by international nomenclature committees [68] – both to indicate partial endogenicity and (another reason for “circa” that is too often forgotten) to refer to the also-encountered variability inherent in the cycle’s characteristics. “Circadian” was intended to replace “diurnal,” a term used in biomedicine to distinguish activity or morbidity patterns during the daily photofraction from those during the daily scotofraction, as diurnality vs. nocturnality, respectively. While they are obvious signatures of the photic day, circadians are also influenced nonphotically by natural and artificial electric fields [69] and by subtle environmental factors in caves [70]. Circadian timing accounts for the difference between ~75 % survival and ~75 % death from the same dose of a cardiac drug, among others, under controlled laboratory conditions as a function of timing (Fig. 21.7f). Alterations of circadian characteristics result, e.g., in vascular variability disorders (VVDs) that represent a risk of stroke which is greater than that of hypertension and can be treated (Fig. 21.7g).
21.5 About-7-Day Cycles: Circaseptans
Almost-ubiquitous circadians were soon complemented by other spectral components including about (not precisely)-7-day rhythms, dubbed circaseptans (Fig. 21.8a) [71]. The recognition that a free-running about-weekly schedule resides in part in living matter came first from the breakdown products (metabolites) of the very adrenocortical and gonadal hormones (17-ketosteroids, 17-KS), which are important for survival and reproduction (Fig. 21.8b), the circadian story repeated itself. An apparently free-running rhythm in 17-KS had a period close to yet different from precisely 7 days (Fig. 21.8a, b) [71]. On weekends, the subject (CH) drank an extra cup of tea and a bottle of beer, a schedule that synchronized the volume he excreted and measured, but not the steroid metabolites contained in his urine which he determined for 15 years by the same method, with the same reagents bought at the outset. In other words, the precise 7-day period found for CH in the urine volume in which the metabolites had been determined, coexisted with a period of about (but different from precisely) 7 days in the excreted steroidal metabolite and was also different (desynchronized) from another near-7-day period in the planetary geomagnetic index Kp during the urine’s collection span [71].


















Fig. 21.8
(a) Spectrum of urinary 17-ketosteroid excretion by a clinically healthy man measured over 15 calendar years. Estimates of periods, amplitudes, and standard deviations in six of the many more spectral regions are shown. Analyses in 4 spectral regions by least-squares spectra are on 4 separate sections. A number of cycles with long periods characterize this one-and-the-same biological variable (and other body functions). Reproducibility is seen when four sections are analyzed separately. For added components, see Fig. 21.8b (© Halberg Chronobiology Center). (b) Spectral windows with transyears are shown globally (left and right) and are resolved as a function of time (middle) (© Halberg Chronobiology Center). (c) Moving infradian-to-circasemidian spectral window of the heart rate of a boy born at term during the first 40 weeks of life shows prominent infradians postnatally. Circadian-over-circaseptan prominence starts toward the end of the first month of life: side view of relative prominence. Original data of Yoshihiko Watanabe (© Halberg Chronobiology Center). (d) Circaseptan-over-circadian prominence observed for the electrical potential of the unicell Acetabularia acetabulum following its release into continuous light, after prior synchronization to a regimen of 12 hours of light alternating with 12 hours of darkness. Original data of Hellmuth Broda (© Halberg Chronobiology Center). (e) Time course of tumor cell growth pooled across four kinds of tumor cells shown after normalization for weeks 2 and 3 of study to illustrate circaseptan-over-circadian prominence. Irradiation shortly after the circaseptan-circadian maximum of β-ATP is associated with larger tumor cell kill (lesser survival) than irradiation shortly after the circaseptan-circadian minimum of β-ATP (middle). Original data of Waldemar Ulmer (© Halberg Chronobiology Center). (f) Chronobiologic serial sections carried out at a trial period of 164.4 hours (left) and 168 hours (7 days) (right) for blood pressure and heart rate measured ambulatorily, mostly at 15-minute intervals for 16 months by a woman 67 years of age at the start of monitoring (top 3 panels) and for the north–south component (Bz) of the IMF (bottom panel). Shading (by bracketing dots) indicates statistical significance and its size corresponds to a 95% confidence interval of the acrophases. A mostly statistically significant (when shaded) circadian component is found for all variables, with both fits. The acrophase curve of Bz is horizontal (stable) at a trial period of 164.4 hours, whereas the acrophase curve of blood pressure is more or less horizontal at a trial period of 168 hours. The time course of the heart rate phases drifts with the 168-hour fit. The physiological variables are differently synchronized, blood pressure mainly by the societal week and heart rate pulled by the 164.4-hour period of Bz. In the case of heart rate, however, a small phase shift occurs more or less at the middle of the monitoring span. This shift in circadian acrophase of heart rate coincides with a similar shift in circaseptan acrophase of Bz from the interplanetary magnetic field. The left half of this figure shows that blood pressure clearly drifts away from 164.4 hours, further validating the 7-day-synchronized circaseptan component in blood pressure. In the case of heart rate, there is also a transient drift of the acrophase of the 164.4-hour component for several months, but it is not as pronounced or consistent as that observed for blood pressure. Moreover, there are spans when the 164.4-hour acrophase seems to lock and remain stationary and thus consistent with this period at the beginning of the series and again in the second half of the monitoring span. These are spans when a stable 164.4-hour acrophase is also observed for Bz. From these observations, it appears that Bz may pull heart rate and that there is occasional phase locking as well as frequency-locking between these two variables. It should be noted further that the phase difference between heart rate and Bz at the beginning of the series is about 90°, whereas in the second half of the series, the phase difference is much smaller; this means that when there is frequency-locking between the two variables, this may occur with a different phase difference. Whether or not these phase differences and phase locking follow a predictable pattern such as being dependent upon the stage of the solar activity cycle remains to be further investigated. These results are only observational at this stage, and a mechanism or model accounting for these observations has not yet been developed. One avenue that seems promising may be to consider the possibility that there is more than a single circaseptan component. This was the case for geomagnetic pulsations in Antarctica as well as in geomagnetic indices during some but not necessarily all spans investigated, since recording of aa or Kp started: one component was close to 7 days and the other was slightly shorter than 7 days. When two components with close periods are present jointly, they may pull during some spans, but not during others, depending upon the relative instantaneous phase difference between the two different components, e.g., whether they are in phase or out of phase (© Halberg Chronobiology Center). (g) Congruence can characterize characteristics such as the period or phase of a spectral component in 2 or more time series of the same variables or of different variables within tissues, organs, organ systems, and disciplines and, most interestingly, when they are transdisciplinary, as are transyears (© Halberg Chronobiology Center). (h) Transdisciplinary congruence in the circaseptan spectral region (© Halberg Chronobiology Center). (i) Combination of gliding spectral window on top with special focus on the behavior of two selected periods (middle and bottom), with the time course of the phase validating the 6.77 period by a more or less horizontal trajectory of phases in only part of the record, but invalidating a precise 7-day periodicity since no dots bracket any horizontal time course of phases, and the small initial section with dots shows a gradual advance (© Halberg Chronobiology Center)
Second, there is the observation that circaseptans appear after the administration of a single stimulus that carries no 7-day information, as in the case of a partial hepatectomy studied by Hübner [72] in rats and by us after human kidney [73, 74] or heart [75] transplantation. Single stimulus induction of circaseptans, or rather their manifestation after a single stimulus, traced back to antiquity, was also the topic of a classic book on circaseptans by Gunther Hildebrandt and Ingrid Bandt-Reges [76].
Third, as to ontogeny, a dominant weekly pattern, much more prominent than the also-present circadian component in the same data, was seen in a survey of more than 181 profiles of blood pressure, each profile on a separate baby, each measured every 30 minutes each for 48 hours on different 2 consecutive days of the first week of life, and in several hundred additional longitudinal monitorings for several weeks during the first months of life; by the fourth week of life, the circadian component usually dominated the human spectrum (Fig. 21.8c). In a crayfish, the circaseptan dominated the activity pattern at the age of 6 months. Aeolian circaseptans persisted into adolescence, albeit they came and went and were only quasi-persistent.
Fourth, again as to ontogeny, the circaseptans regain relative prominence with elderly age, concurrently with a circadian decrease in amplitude [77].
Fifth, as to phylogeny, the giant unicell Acetabularia acetabulum, standardized in light and darkness alternating every 12 hours, thereafter released into continuous light, after signal averaging of electrical activity, shows a circaseptan rhythm with a free-running near-one-week period, with a much larger amplitude than that of the also free-running circadian rhythm (Fig. 21.8d). Assuming that this alga has not changed in the interim, this eukaryote may tell us about the environmental cycles it adapted to when it developed, perhaps 500 million years ago [78] at a time that physical instruments cannot yet readily trace.
Sixth, like Acetabularia, tumor cell cultures, as spheroids or as monolayer, also show a major circaseptan peak in β-ATP concentration, with a superposed circadian in amplitude that is barely one-third of the circaseptan (Fig. 21.8e). While Acetabularia shows free-running circadians and circaseptans, the tumor cells are 1- and 7-day synchronized. While Acetabularia shows a dispersion of periods after a first cycle or so, the tumor cells remain 1- and 7-day synchronized for 6 consecutive cycles. Tumor cell kill by radiation was much better at the circadian and circaseptan peak of the β-ATP marker, as compared to radiation administered at the circaseptan and circadian minimum [79, 80].
21.6 Remove and Replace Revisited, with Circaseptans
About-7-day rhythms opened a new chapter in chronomics, the aligned recording of variables in and around us. Again a remove-and-replace approach proved to be helpful, except that this time the sun, not the surgeon (in blinding) or genetics (in the case of congenitally blind ZRD mice), did the removal and the cosmos the replacement: when solar activity had 7-day components in its (Walsh) spectra (computed in St. Petersburg) [81], human HR (in Minnesota and Connecticut) had these components amplified in its spectrum and vice versa when these components were lacking in the sun’s record, the HR’s corresponding components were damped, but not lost (Fig. 21.2a, 2b); they were also partly built in [53, 71]. This coding of the circaseptans in our genes is compatible with resonance; hence, it is hardly surprising to find an occasional pull of a biological circaseptan acrophase by a geomagnetic cycle with a proximal frequency, e.g., for HR but not for BP in Fig. 21.8f.
Before us, Fraser-Smith [82] found a precise 7-day component in the geomagnetic planetary index Ap. We confirmed him, of course [83], for the precise data accumulated by the time when he did the analyses, but in the longer series of the planetary geomagnetic index Kp, which we subsequently analyzed many years later [84], the major period of a component in the circaseptan spectral region was of ~6.75 days [83, 85, 86]. Others subsequently confirmed this component in Kp, noting its changes with solar cycle stage [87], and extended it to the antipodal geomagnetic index aa [88], as we did subsequently, in the context of extensive studies on biomedical circaseptans, notably by the school of Gunther Hildebrandt [76, 86, 89], among others [74, 90, 91].
At this point, it seemed worthwhile to look for congruence, defined for aeolians in view of overlying or overlapping CIs (95% confidence intervals) of two or more periods or phases, as visualized in the abstract Fig. 21.8g. By congruence, we test for the correspondence of periods and only in this restricted (rather than literal) sense for the reciprocity of two or more periods. Congruences were found among time series of the same variable, organ, organ system, or organism, also within a biological discipline such as physiology or within areas of physics, and furthermore transdisciplinarily among variables of many different disciplines such as cosmo-helio- or geophysics vs. physiology, pathology, and epidemiology, e.g., Fig. 21.8h.
21.7 Differential Bioenvironmental Circaseptan Congruences
The transdisciplinary Fig. 21.8h summarizes data from an elderly man, GSK (with MESOR-hypertension complicated by an intermittent circadian BP overswing), who performed rigorous half-hourly monitoring with few gaps for 10 years, aligned with changes during the same span in a few variables of his terrestrial and more remote environment. These results stem from global (again in the sense only of “based on the entire time series”) spectral windows computed for a narrow about-7-day region of the spectrum; multiple components are seen in physics and physiology, each with a CI (95% confidence interval) shown as the length of a horizontal line through the point estimate of a period, the latter shown as a diamond. The top row shows periods found in the speed of the solar wind (SWS). Four SWS components are congruent with one or another of the periods detected (in the next 3 rows) in three related geomagnetic indices, the equatorial Dst and the planetary Ap and Kp. As noted with the abstract Fig. 21.8g, congruence refers to no more than overlapping or overlying CIs of a characteristic such as the period and not necessarily to causal relations. The global summary in Fig. 21.8h cannot tell whether any particular component was present only during different sections of the series rather than during the entire span. In view of aeolian behavior, any intermittent presence of one or the other, if not of most components, is hardly surprising.
Chronobiologic serial sections, aligned with a gliding spectral window of the SWS in Fig. 21.8i, support caution by revealing that we are dealing with a component drifting in frequency that is 6.77-days long, present consistently (while phases are bracketed by dots representing CIs) only part-time (during the descending phase of solar cycle 23). In this approach, the fit of the wrong period of 7.00 days, also shown, reveals no consistent statistical significance during a relatively long section of the span investigated and hence serves to rule out a precise 7-day component. (When phases are bracketed by dots, they are advancing, indicating a period shorter than that fitted.) By contrast, the fit of two correct periods in the same span examined suggests the concomitant presence of two separate wobbly circaseptan components in human HR, one slightly longer, the other somewhat shorter than 7 days (not shown).
Congruence in Fig. 21.8h of a 7.03-day component among the three geomagnetic indices is not, however, shared by SWS. This is hardly surprising since no more than a plot (not shown) of Kp vs. Wolf numbers reveals differences as well as similarities between these variables that, if then available, may have prevented General Sir Edward Sabine from saying that the behavior of sunspots and geomagnetic activity is identical, an impression that may well be gained from viewing the much shorter time series of the two variables that were available during his time.2 Furthermore, some environmental signatures in HR and BP differ. There is congruence for the 6.78-day component found in SWS and all three geomagnetic variables with HR, but not with BP. There is no congruence of HR or SW for an about-7.03-day component found in the geomagnetic indices and in both systolic and diastolic BP. But spurious differences that can come about from many sources, of course, cannot be ruled out, just as congruence in itself does not rule in a relationship.
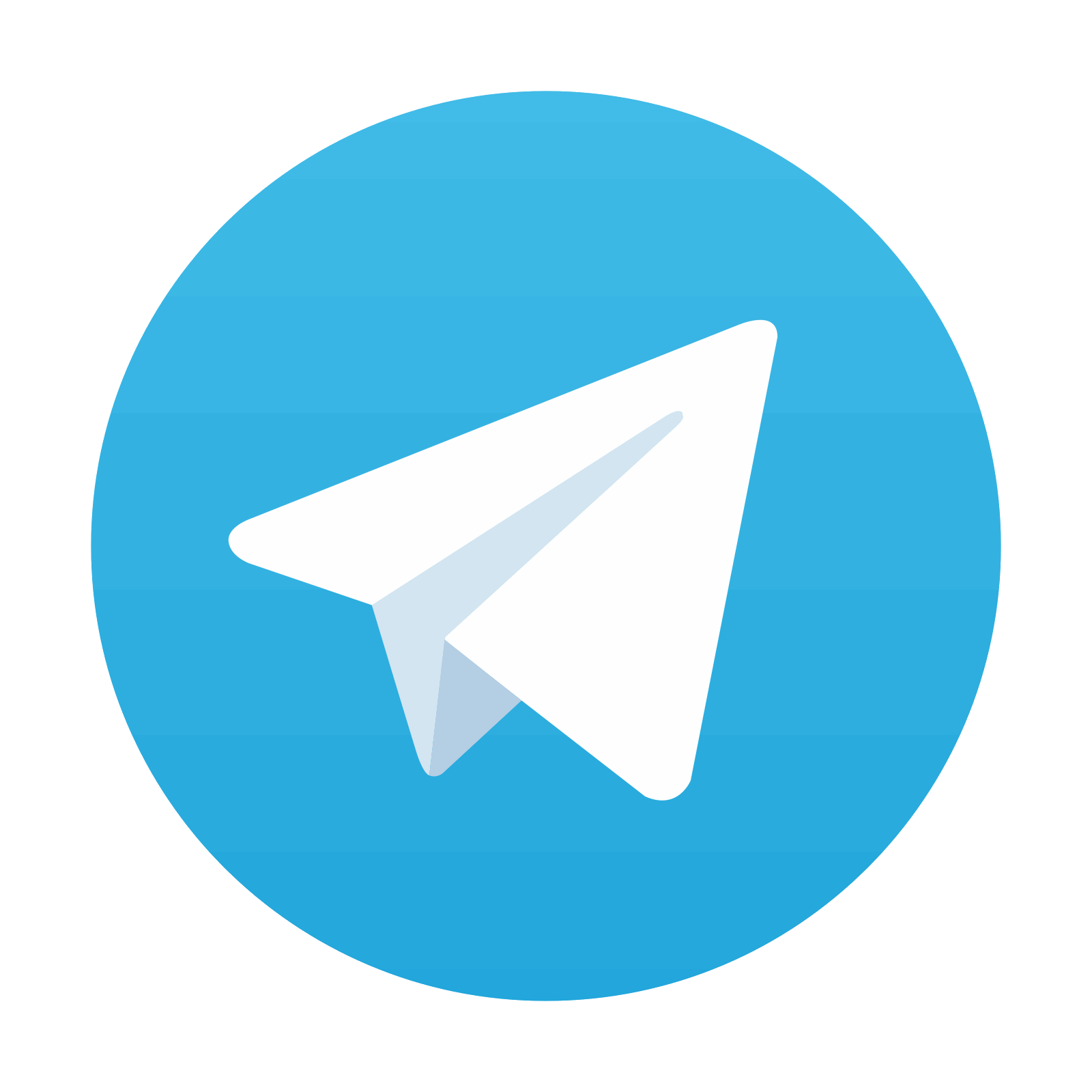
Stay updated, free articles. Join our Telegram channel

Full access? Get Clinical Tree
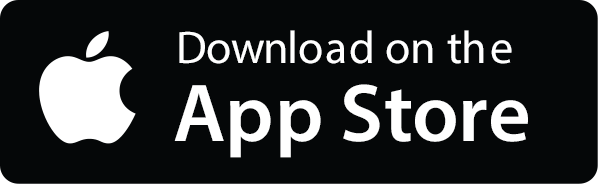
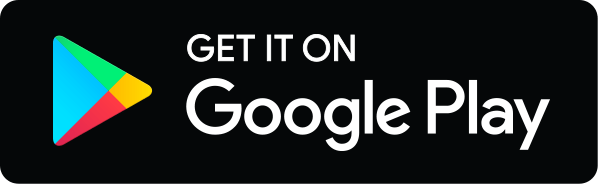