Fig. 1.1
CT scan (left panel) and vertical distributions of ventilation (open squares) and perfusion (closed circles) in an anesthetized subject (right lower panel). Ventilation and perfusion distributions in a waking subject are also shown (right upper panel). In the anesthetized subject, atelectasis can be seen in the bottom of both lungs. Note also that most of the ventilation is distributed to the upper half of the lung and is decreasing in the lower half until the bottom where the ventilation has ceased. This is different from the distribution in the waking subject. Perfusion on the other hand increases down the lung, similar to the waking situation, except for the lowermost part where a certain decrease can be seen. This causes a considerable ventilation/perfusion mismatch with high V/Q in the upper half of the lung, mimicking dead space ventilation, and low V/Q and shunt in the lowermost regions
1.3 Formation of Atelectasis
In their classic paper, Bendixen and coworkers proposed “a concept of atelectasis” as a cause of impaired oxygenation during anesthesia [9]. However, atelectasis could not be shown on conventional chest X-ray. With the introduction of radiological computed tomography (CT), densities were seen in dependent lung regions in anesthetized pediatric and adult patients [10, 11]. Morphological studies in various animals showed them to be atelectasis (see an example in Fig. 1.1, left panel).
Atelectasis appears in around 90 % of all patients who are anesthetized during spontaneous as well as mechanical ventilation and whether intravenous or inhalational anesthetics are used [11]. The atelectatic area on a CT near the diaphragm is on average 3–4 % of the total lung area but can easily exceed 15–20 %. The amount of tissue that is collapsed is even larger, the atelectatic area comprising mainly lung tissue, whereas the aerated lung consists of tissue and air. Thus, 10–20 % of the lung is regularly collapsed at the base of the lung during uneventful anesthesia before any surgery has been done. Abdominal surgery does not add much to the atelectasis, but the lung collapse can remain for several days in the postoperative period [12]. After thoracic surgery and cardiopulmonary bypass, more than 50 % of the lung can be collapsed still several hours after surgery [13]. The amount of atelectasis decreases toward the apex that is mostly spared (fully aerated). It is likely that the atelectasis is a locus of infection and that it can contribute to pulmonary complications [14, 15].
1.4 Prevention of Atelectasis
The major cause of atelectasis during anesthesia is closure of airways. This is important to remember when considering techniques to prevent atelectasis or reopen collapsed lung tissue. Compression of the lung might be suspected to be a major or additional cause of atelectasis, but this is not likely. Airways will close before alveoli collapse when the lung shrinks. This brings us to the second factor that is needed to cause atelectasis, resorption of the gas that is trapped behind closed airways. The higher the oxygen concentration, the faster is the resorption of gas and atelectasis formation [11] (one may even ask how much of lung collapse in the ARDS patient is caused by compression and how much by gas resorption). Thus, fall in FRC and high oxygen concentration are both needed to produce alveolar collapse, at least when considering the relatively short time of most anesthesias.
Positive end-expiratory pressure
PEEP is a simple technique to increase lung volume and airway dimensions. Depending on the magnitude of PEEP, airways may be reopened, but whether the same level of PEEP is high enough to recruit collapsed alveoli is less certain. Airways may close at an airway pressure of 6 cmH2O in a normal-weight anesthetized subject [16] and, most likely, at higher pressure in an obese subject. Perhaps a rule of thumb (not clearly tested) would be PEEP of 7 cmH2O when BMI is below 25 kg/m2, 9 cmH2O up to 32 kg/m2, and higher in more obese subjects to keep airways open. If this PEEP is applied before any atelectasis has been produced, it is likely that it can prevent formation of it. To confuse things, it should be mentioned that the application of 10 cmH2O PEEP consistently reopens collapsed lung tissue. It requires some time, still only minutes, and may rather be an effect of increased inspiratory airway pressure than of PEEP per se [11]. It may not reopen all previously collapsed lung tissue, even if applied during a prolonged period of time. Moreover, arterial oxygenation is not improved in proportion to the decrease in atelectasis because of shift of blood flow to more dependent, still atelectatic lung regions (Fig. 1.2). Also, PEEP higher than 10 cmH2O may be associated with derangement in hemodynamics [3]. This does not preclude the use of PEEP, but presumably an optimal and individual PEEP is needed to balance the effects of recruitment and circulatory impairment.


Fig. 1.2
Gamma camera images of lung blood flow in an anesthetized and mechanically ventilated patient in the lateral position. The left panel shows more perfusion to the lower lung, the middle panel shows how perfusion is almost absent in the upper lung with a general PEEP of 10 cmH2O, and the right panel shows how perfusion is redistributed to the upper lung when a PEEP of 10 cmH20 has been applied to the dependent lung only. The lung per se cannot be seen but the upper lung is larger than the lower one with no or global PEEP (From Ref. [28], with permission by the publisher)
Recruitment maneuver:
A “sigh,” or a double tidal volume, has been suggested to reopen collapsed lung and to improve gas exchange, both for intubated and non-intubated patients [17]. However, the amount of atelectasis does not change during normal tidal breathing or by a “sigh” using an airway pressure of up to 20 cmH2O [11]. At a sustained inflation of the lungs to an airway pressure of 30 cmH2O, atelectasis decreases to approximately half the initial size. Additional inflations of the lung to the same airway pressure (30 cmH2O) only result in minor further opening of lung tissue after the first maneuver. To reopen all collapsed lung tissue in anesthetized adults with healthy lungs, an airway pressure (recruitment pressure) of 40 cmH2O is required. In morbidly obese patients with increased chest wall elastance, a higher airway pressure is required to reach the same transpulmonary pressure as in normal-weight subjects. A high airway pressure of 55 cmH2O, kept for 10 s, was also used for lung recruitment in morbidly obese (BMI >45 kg/m2), anesthetized patient [18].
Recruitment maneuvers also have been used during cardiac surgery [19] (see also below) and in the intensive care setting [20]. As there is a complex interaction between time and pressure, the time frame possibly differs if other recruitment pressures are used [21]. As an alternative, a stepwise increase in PEEP can be used [22].
Oxygen and atelectasis during induction of anesthesia
Preoxygenation is provided to prevent hypoxemia in the event of a difficult intubation of the airway and will for the anesthetist be an important procedure to ensure maximum safety. However, the formation of atelectasis should be recalled, and it will by itself shorten the “apnea tolerance time,” i.e., the time before hypoxemia develops.
Avoiding the preoxygenation procedure and ventilating with 30 % instead of 100 % O2 prevents formation of atelectasis during the induction and subsequent anesthesia [23]. In studies atelectasis appeared in all patients who were preoxygenated with 100 % O2, was much smaller with 80 % O2, and was almost absent with 60 % O2. However, the smaller amount of atelectasis with lower oxygen concentration during induction remains only for a limited time. The patients receiving 80 % O2 during induction had as much atelectasis as those on 100 % O2 40 min later [24]. This is because the gas trapped behind closed airways consists of 80 % O2 and will be resorbed during the ensuing period and finally results in airlessness, i.e., atelectasis. Reopening of closed airways by a recruitment maneuver with lower O2 concentration, e.g., 40 %, even in the absence of atelectasis, will replenish the closed region with lower O2 gas, and this will slow down resorption atelectasis even more, hopefully for the rest of the anesthesia.
Anesthesia might be induced during ventilation with CPAP that will prevent the fall in FRC and atelectasis formation [25]. Oxygen can be used to full extent, and, moreover, the lung volume is higher compared to no use of CPAP/PEEP, resulting in a larger oxygen reservoir and increased safety time in the event of a complicated intubation of the airway.
Oxygen and atelectasis during anesthesia
Ventilation of the lungs with pure oxygen after a vital capacity maneuver that had reopened previously collapsed lung tissue resulted in a rapid reappearance of the atelectasis [11]. If, on the other hand, 40 % O2 in nitrogen was used for ventilation of the lungs, atelectasis reappeared slowly, and 40 min after the vital capacity maneuver, only 20 % of the initial atelectasis had reappeared. Thus, ventilation during anesthesia should be done with a moderate fraction of inspired oxygen to prevent atelectasis formation, but if higher oxygen is considered necessary, it can be given during PEEP ventilation.
Oxygen and atelectasis during emergence from anesthesia
Another situation where a high oxygen concentration is used is at the end of the anesthesia. A post-oxygenation maneuver is regularly performed to reduce the risk of hypoxemia during the wake up. This may be done in combination with airway suctioning to eliminate secretions. However, the combination of oxygenation and airway suctioning will most likely cause atelectasis, and there is indeed no other potential maneuver that can compete with post-oxygenation and airway suctioning in doing so.
The findings of atelectasis during anesthesia and the possibility to recruit lung tissue with an inflation of the lung has prompted studies on the use of recruitment maneuver at the end of the surgery and anesthesia. Again, the influence of inspired oxygen plays an important role. Thus, recruitment at the end of the anesthesia followed by ventilation with 100 % oxygen (the latter again being common in routine anesthesia) caused new atelectasis within the 10 min period before anesthesia was terminated but not if ventilation was with lower FiO2 [26]. Another approach to prevent atelectasis to persist into the postoperative period is to use PEEP until extubation of the airway and to continue with the CPAP for a limited time, e.g., 15–30 min during which period inspired oxygen concentration is lowered to 30 % in the air. In a small study where this technique was applied, atelectasis was reduced to less than a third compared to control patients with no PEEP/CPAP as assessed by CT one hour after wake up [27].
1.5 Individual Lung Ventilation
An individual lung ventilation technique was developed more than 30 years ago in order to optimize ventilation distribution in proportion to individual lung blood flow. It was successful in improving oxygenation but was considered too complicated to be used in intensive care. Thus, it required that:
- 1.
The patient was in the lateral position
- 2.
A double lumen endobronchial catheter was inserted
- 3.
Two ventilators were used
This made it possible to apply a higher PEEP to the lower lung where most of the atelectasis should be and to ventilate each lung separately so that 50 % of ventilation was given to the upper, nondependent lung and 50 % to the lower, dependent lung. This was assumed to match the distribution of blood flow between the two lungs [28] (Fig. 1.2). Despite its technical complexity, it was also tested in anesthetized patients. Also, during anesthesia, gas exchange could be improved, and CT scanning showed that atelectasis could efficiently be removed from the dependent lung without undue overexpansion of the nondependent lung. The concept has been revived recently, at least experimentally, using better monitoring technique and, more importantly, distributing ventilation in proportion to the lung mechanics of each lung rather than its perfusion. This may not optimize gas exchange but should reduce stress and strain of the lung with possible protective effect on inflammation. Having this as the objective, ventilation will be distributed automatically between the two lungs in proportion to their regional compliances (or, rather, their time constants). Recruitment of collapsed lung tissue with no overexpansion and ventilation of each lung at their optimum PEEP levels can be achieved, as shown in an animal model [29] (see also Fig. 1.3). A simple pneumatic system that allows the use of only one ventilator, still providing different PEEP to the two lungs, does exist [30], and there is today a double lumen tracheal tube that facilitates the insertion and fixation of the tube. The potential value of this in thoracic surgery and in intensive care remains to be studied.


Fig. 1.3
Amount of atelectasis in the nondependent and dependent lung in piglets in the lateral position after creating a ventilator-induced lung injury (repeated lung lavages and vulnerable ventilation). Note that when the end-expiratory pressure decreased from 20 cmH2O to downward, atelectasis rapidly increased from 12 cmH2O in the lower dependent lung and not until 6 cmH2O in the upper nondependent lung (From Ref. [29])
1.6 One-Lung Ventilation
During one-lung ventilation (OLV), one lung is separated from ventilation to enable surgery on that lung, and it does not participate in the pulmonary gas exchange. There is a persisting perfusion that causes shunt and decreased arterial oxygenation. Hypoxic vasoconstriction (HPV) reduces this blood flow, whereas kinking of pulmonary vessels because of compression and distortion of the lung seems to have less effect on blood flow [31].
The other lung is ventilated and perfused. The patient is normally in the lateral position with the non-ventilated lung in the upper position to facilitate surgery and the ventilated lung in the dependent position. Atelectasis is produced in the dependent lung, and pulmonary shunt is regularly larger than 11 % and the PaO2 reduced by 50 % or more during OLV [32]. A traditional approach to mechanical ventilation during OLV has been high tidal volume (10–12 ml/kg) and zero PEEP to the dependent ventilated lung, high tidal volume to keep the lung open, and no PEEP to preserve the effect of the HPV in the nondependent lung and minimize blood flow to it [33]. However, pulmonary complications are common, both during the anesthesia per se with shunt and hypoxemia (see above) and postoperatively. Pathophysiological disturbances include high airway pressures, ventilation/perfusion mismatch with shunt, increased pulmonary capillary pressure, and cyclic alveolar collapse. These events may result in alveolar damage followed by pulmonary edema with diffuse alveolar injury, leucocyte sequestration, and alveolar cytokine release, a series of events frequently called mechanotransduction (for a review, see [34]). Moreover, on termination of OLV there can be persisting hyperperfusion in the dependent, ventilated lung, associated with an increased diffuse alveolar damage score, as seen in porcine experiments [35].
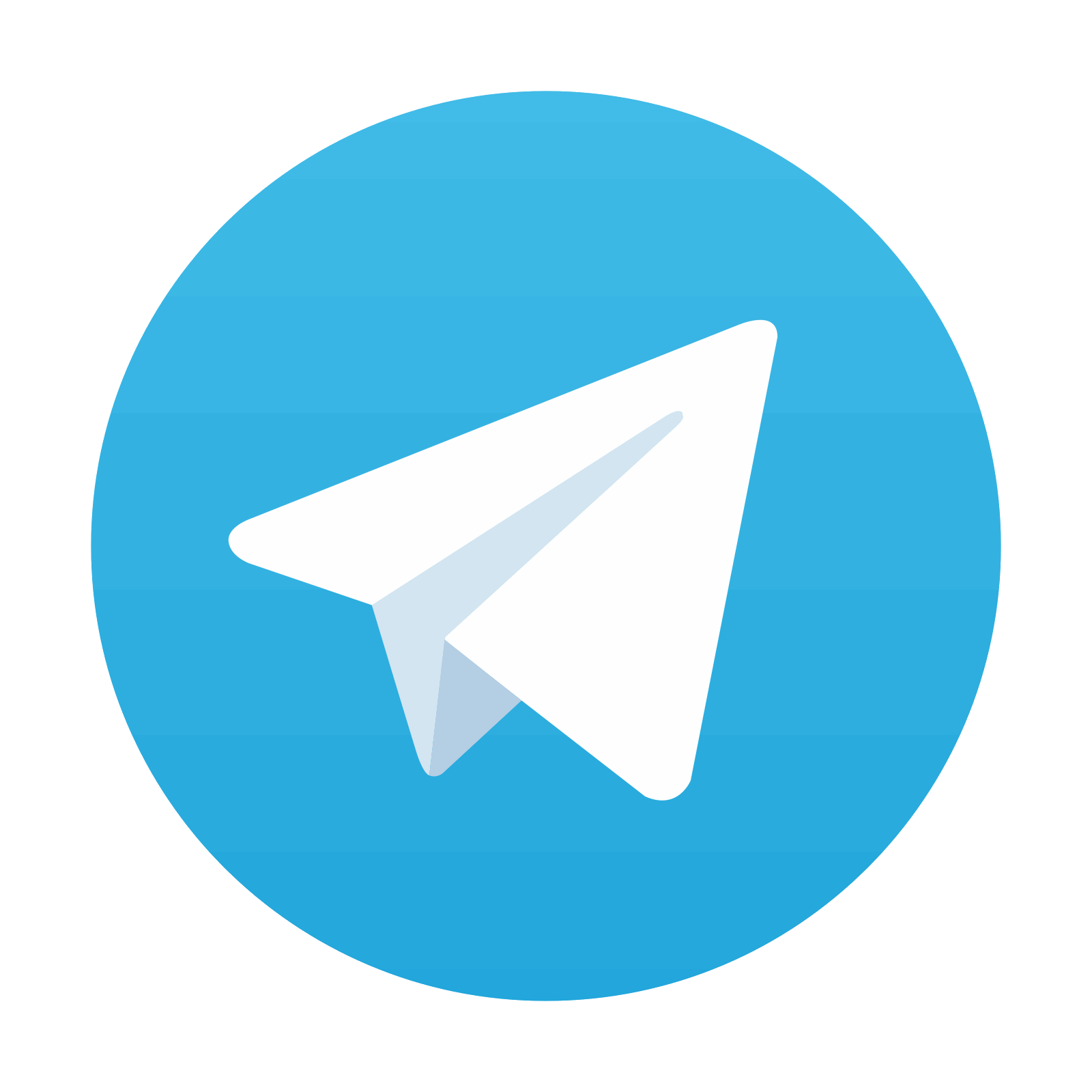
Stay updated, free articles. Join our Telegram channel

Full access? Get Clinical Tree
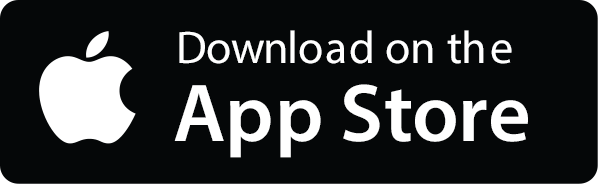
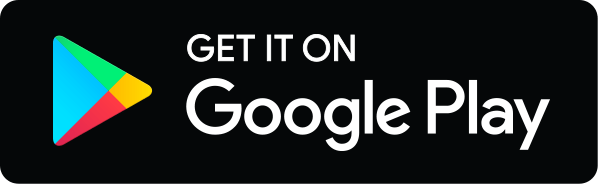