Fig. 7.1
Pathological images of coronary plaques. (a, b) Vulnerable plaques with thin fibrous cap (arrow) and large lipid core (*). (c, d) Stable plaques with relatively thick fibrous cap (arrowhead) and small lipid core (*)
7.3 Frequency of Vulnerable Plaque and Acute Coronary Syndrome
Since all vulnerable plaques do not always cause ACS, it is important to determine the incidence of ACS due to vulnerable plaque. Ohtani et al. followed patients for 4.8 years after angioscopy and found that respective incidence of ACS was 2.2- and 3.8-fold higher in patients with ≥2 and ≥5 yellow plaques per vessel, compared with those who had ≤1 yellow plaque per vessel [10]. On the basis of angioscopy, plaque is defined as a nonmobile, elevated, and/or protruding structure that can be clearly demarcated from the adjacent vessel wall. Coronary plaques are divided into yellow or nonyellow plaques (white plaque) according to the surface color. Ueda et al. estimated that vulnerable plaques may cause ACS at a rate of approximately 0.3–1.0 %/year [11]. Taking into consideration the estimated frequency of vulnerable plaque to ruptured/disrupted plaque, the frequency of ruptured plaques causing ACS may be approximately 1–5 %/year [11]. Another prospective angioscopic study that was conducted in the non-statin era reported that yellow plaques caused ACS at a rate of 5.9 %/ year, whereas white plaques caused ACS at a rate of 2.1 %/year [12].
In patients who died of plaque rupture, additional lesion sites remote from the culprit plaque show TCFAs in 70 % of cases. On the other hand, TCFAs are less frequent (30 %) in cases where death is attributed to fibrocalcific plaques with flow-limiting stenosis, regardless of whether there is myocardial infarction or plaque erosion [13].
All vulnerable plaques are not likely to progress to rupture. Therefore, it is important to consider the results of a pathological study in which the coronary arteries were sectioned serially from the coronary ostium to a distal intramyocardial location. In this study, mean luminal narrowing was the least in lesions with TCFAs (59.6 %), intermediate in lesions with hemorrhage into a plaque (68.8 %), and greatest in acute plaque ruptures (73.3 %) or healed plaque ruptures (72.8 %) [14]. Approximately 50 % of the TCFAs occur in the proximal portions of the major coronary arteries (left anterior descending > left circumflex > right coronary artery), with another one third in the midportion and the remaining few in the distal portion [14]. The number of vulnerable plaques correlated with both high total cholesterol and the total cholesterol/high-density lipoprotein cholesterol ratio [15].
7.4 Plaque Erosion as Vulnerable Plaque
Plaque rupture/disruption refers to a lesion consisting of a necrotic core with an overlying thin ruptured fibrous cap that leads to luminal thrombosis because of contact of flowing blood with a highly thrombogenic necrotic core [16]. On the other hand, plaque erosion shows a luminal thrombus with an underlying base rich in smooth muscle cells and proteoglycans with mild inflammation [17]. Erosion of proteoglycan-rich and smooth muscle cell-rich plaques lacking a superficial lipid core is one of the findings in sudden death due to coronary thrombosis. Compared with plaque ruptures, these lesions are more often seen in younger individuals and women, have less luminal narrowing and less calcification, and fewer macrophages and T cells [17]. Kolodgie et al. postulated that the lack of significant inflammation at the site of erosion raises the possibility that erosion represents chronic injury rather than true atherogenesis. The abundance of a proteoglycan and hyaluronan matrix suggests their potential role in the development of thrombosis [18]. Although erosion can be detected by angioscopy and optical coherence tomography (OCT) [19, 20], the exact type of images that indicate pre-stage erosion has not yet been determined. Angheloiu et al. observed superficial foam cells in erosion-prone plaques by intrinsic fluorescence spectroscopy and diffuse reflectance spectroscopy in an autopsy study [21].
7.5 Vulnerable Plaques Detected by Angioscopy
Angioscopy applies fiber-optic technology to directly visualize the luminal surface and is able to characterize plaque composition on the basis of luminal appearance and to distinguish between white and red thrombus [22]. Endoluminal irregularities such as ulceration, fissures, erosions, and tears also can be seen using angioscopy. Therefore, among the major criteria of vulnerable plaques that are mentioned above, endothelial denudation with superficial platelet aggregation and fissured/injured plaque are the features that can be detected by angioscopy. Furthermore, among the minor criteria, superficial calcified nodules, yellow color (on angioscopy), and intraplaque hemorrhage are the features that can be detected by angioscopy. Takano et al. compared angioscopic findings with the results of OCT [23]. Angioscopic findings were semiquantitatively classified based on the surface color as 0, white; 1, light yellow; 2, medium yellow; and 3, dark yellow. They demonstrated that there was an inverse relationship between yellow grade and the fibrous cap thickness (grade 0, 218 ± 89 μm; grade 1, 101 ± 8 μm; grade 2, 72 ± 10 μm; grade 3, 40 ± 14 μm). Angioscopic yellow plaques can be identified as lipid plaques with high sensitivity (98 %) and specificity (96 %) based on the fibrous cap thickness measured at 110 μm [24]. Therefore, another major criterion for vulnerable plaque (thin fibrous cap with a large lipid core) can be indirectly detected by angioscopy (Fig. 7.2). Regarding detecting vulnerable plaques, MacNeill et al. showed macrophage infiltration in coronary plaques in vivo using OCT and elucidated the relationships between increased macrophage infiltration and the incidence of ACS (Fig. 7.3) [25]. Virmani et al. reported that a thin cap <65 μm is a more specific precursor of plaque rupture [26]. However, Tanaka et al. demonstrated by OCT study that some plaque rupture may occur in thick fibrous cap depending on exertion levels (Fig. 7.4) [27].




Fig. 7.2
(Courtesy of Dr. Takano M and Prof. Mizuno K). Corresponding angioscopic images and optical coherence tomography (OCT). Upper: angioscopic images. Lower: OCT. (a) A dark yellow plaque (yellow grade 3) is presented as a lipid plaque (*) with a thin fibrous cap (white arrow). The minimal thickness of the fibrous cap is 20 μm. The arc of lipid was measured as an angle between the two straight lines that joined the center of lumen to both ends of the lipid area. (b) A white plaque (yellow grade 0) contains lipid core (*) under a fibrous cap. The fibrous plaque thickness at the thinnest part (white arrow) is 130 μm. (c) A white plaque (yellow grade 0) that is diagnosed as a fibrous plaque (*) by OCT. (D) A red thrombus on a yellow plaque is observed by angioscopy. Protruding thrombus (white arrow) overlying thin-cap lipid plaque (*) is recognized by OCT

Fig. 7.3
Fibrous cap segmentation and macrophage density images for lipid-rich plaques. (a) A normalized standard deviation (NSD) image. (b) Optical coherence image of a lipid pool (LP). *: guide wire shadow artifact. (c) Outline (red) of the segmented fibrous cap of the OCT image depicted in panel b. (d) NSD image superimposed over a standard intensity image shows locations corresponding to increased macrophage density. The color scale bar represents the color mapping of the NSD parameter (Ref. [25])

Fig. 7.4
Representative cases of plaque rupture occurring at rest or with exertion. Left: plaque rupture that occurred at rest. Fibrous cap was broken at the midportion, and a thin fibrous cap could be observed. Right: plaque rupture that occurred during heavy farm work. Thick fibrous cap was broken at the shoulder of plaque (Ref. [27])
Uchida et al. employed another classification of yellow color on angioscopic images [28]. They divided yellow plaques into glistening yellow plaques and non-glistening yellow plaques. A glistening yellow plaque is a plaque exhibiting yellow fluorescence-like color with a glistening portion usually located in the area of the plaque center. Yellow fluorescence-like color is a yellow color closely resembling the reflected light by yellow fluorescent paint. Collagen fiber density in fibrous caps in glistening yellow plaques was smaller than that in white or non-glistening plaques, indicating that glistening yellow plaques were histologically vulnerable plaques [24].
7.6 Mechanical Stress as a Vulnerability of Coronary Plaques
When plaques eventually start to intrude into the lumen, shear stress in the area surrounding the plaque changes substantially, increasing tensile stress at the plaque shoulders leading to fissuring and thrombosis [28]. This elevated shear stress has been proposed to cause mechanically induced rupture of the cap [29]. Local biologic effects induced by high shear stress can destabilize the cap, particularly on its upstream side, and turn it into a rupture-prone, vulnerable plaque [28]. Therefore, mechanical stress that influences plaque vulnerability is an important factor as well as the plaque tissue characteristics [30]. Shear stress induces important biologic effects in endothelial cells that can affect the crucial balance between cap-reinforcing matrix synthesis by synthetic smooth muscle cells and matrix breakdown by metalloproteinases, which are produced by macrophages [31]. In humans, outward vascular remodeling seems to be increased proximal to a coronary stenosis, compared with the impaired outward remodeling seen distally [32].
Preliminary calculations that were made by applying computational fluid dynamics coupled with solid deformation mechanics to a symmetric lesion model showed that a relatively small (20 mmHg) pressure drop can induce ≥10 kPa axial tensile stress in a 75-μm thick cap. Given that the average minimum cap thickness in one previous study was 5.6 μm in patients who died after exertion [33], a peak tensile stress of 134 kPa would load the cap as predicted by the model. This far exceeds the fracture stresses in the lowest tertile reported for caps covering lipid pools in human aortic tissue samples (75 kPa for non-ulcerated plaques and 20 kPa for ulcerated plaques) [34]. This finding might explain why plaque rupture at the midcap (75 %) was the most frequent cause of death (68 %) in the exertion group [33]. In contrast, plaque rupture accounted for only 23 % of the subjects who died at rest and mostly occurred at the plaque shoulders (65 %) [33]; thus, a blood pressure surge might have been a more likely trigger in this group. Fukumoto et al. demonstrated that localized high shear stress might be a trigger for fibrous cap rupture, although the absolute value of shear stress is not sufficient to directly provoke mechanical destruction of the fibrous cap using computational color mapping of the distributions of the streamline, blood pressure, and shear stress [35].
7.7 Recent Investigations on Vulnerable Plaques
The ability of environmental, physical, or emotional stressors to trigger myocardial infarction has been attributed to a surge in sympathetic nervous system activity and catecholamine release that leads to increases in heart rate, blood pressure, and cardiac work as well as local vasoconstriction at the site of vulnerable plaques, thereby precipitating plaque rupture [36]. There is an intriguing working hypothesis that shifts in environmental factors, including local saturation of cholesterol, temperature, pH, and hydration status, could alone or in various combinations lead to cholesterol crystallization with sudden and forceful volume expansion causing plaque fissure and thrombosis [36, 37]. However, this hypothesis needs to be confirmed in larger studies. Intraplaque hemorrhage can enhance this mechanism by triggering the crystallization of free cholesterol from erythrocyte membranes and causing abrupt enlargement of the necrotic core [38].
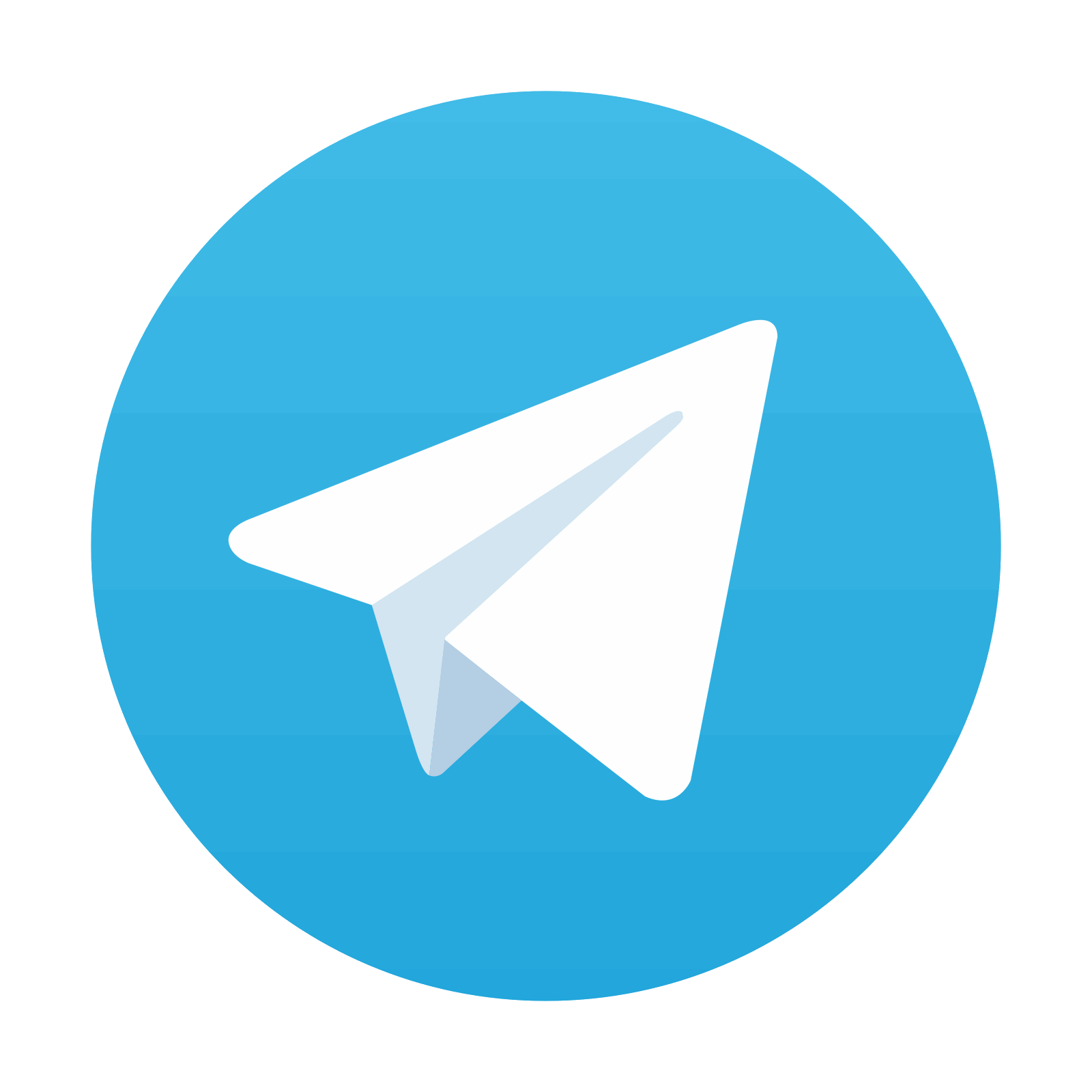
Stay updated, free articles. Join our Telegram channel

Full access? Get Clinical Tree
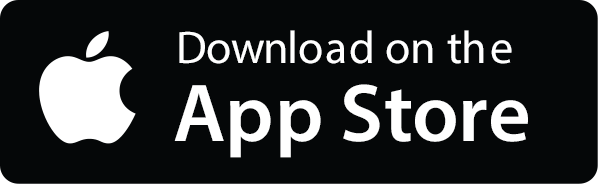
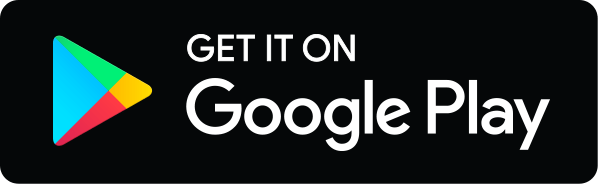