Fig. 12.1
Summary of the main findings regarding BNP and NT pro-BNP in CKD patients
Theoretically, overhydration increases parietal stress and secretion of BNP and NTproBNP; therefore, BNP and NTproBNP could be used as markers for volume status evaluation. However, the diagnostic value has been considered to be limited in CKD patients because renal dysfunction itself and associated cardiovascular diseases that may affect BNP levels. The use of BNP and NT-proBNP as adequate parameters for measurement of VO was compared with other validated methods, such as clinical assessment, BIA or IVC measurements [91–94, 99], with different conclusions. Jacobs et al. also demonstrated in 44 HD patients followed for 6 months that VO parameters measured by BIA had a strong correlation with baseline BNP and NT pro-BNP levels, independently of the patient’s cardiac history [94]. In 2013, Antlanger et al. reported similar results in 212 HD patients in which NT pro-BNP levels were strongly associated with overhydration (fluid status evaluated by bioimpedance), independently of inflammation or high BP [93]. In contrast, Panigua et al. in a large cohort including 753 dialysis patients (both HD and PD) found no correlation between NTproBNP and volume status parameters. Additionally, several studies described a statistically significant relationship between overhydration and BNP or NTproBNP only in patients with left ventricular dysfunction. David et al. found that a cut-off value of ≥7,200 ng/L for serum NT-proBNP discriminates HD patients without LV dilatation (LVD) from those with LVD and that persistent post-HD VO is associated with increased levels of NT-proBNP levels in patients with LVD [91]. Similarly, Booth et al. reported in 62 HD patients with LVD, that NT pro-BNP levels were significantly associated with markers of fluid overload assessed both by clinical evaluation (peripheral edema) and BIA measurement (ECW/TBW) [96].
Importantly, changes in NTproBNP could be better associated with volume status. Davenport et al., report in 2012, after conducting 189 serial measurements in 92 PD that changes in NT-proBNP levels were strongly associated with TBW, ECW and ECW/TBW assessed by BIA. Patients who experienced the highest decrease in NT-proBNP levels also presented with significant reductions of ECW, TBW and ECW/TBW and a decrease in systolic BP from 143.8 ± 24.6 to 136.5 ± 18.7 mmHg; patients with the greatest increase in NT pro-BNP suffered also an increase in systolic BP from 133.5 ± 22.7 to 142.7 ± 28.8 mmHg. Chazot et al. analyzed retrospectively the BNP levels at baseline and during the second quarter of HD treatment, in 46 patients that initiated HD in the last 6 months [100]. At HD initiation, the plasma BNP level was 1,041 ± 178 pg/ml and it was correlated with age, gender (males) and cardiac disease history, but not with mortality risk. The analysis performed at the second quarter of the HD treatment showed that the BNP levels decreased to 631 ± 707 pg/ml and this reduction was associated to SBP decrease. At this stage, higher BNP levels were independently associated with increased risk of mortality, suggesting that BNP could represent a beneficial parameter to follow patient dehydration after HD initiation and that initial fluid overload may act as a confounder factor for its predictive value [100]. In contrast, Breidthardt et al. found no correlation between hydration status and BNP in a 113 chronic stable hemodialysis cohort [101].
Bioimpedance Analysis (BIA) in CKD
This represents a non-invasive, volume measurement technique based on the electrical principle that the body is a circuit with a given resistance (opposition of high and low frequency current flow between intracellular and extracellular compartments) and a given reactance (the ability of cells to store energy) [1, 68].
The BIA method includes: monofrequency and multifrequency bioimpedance analyzed by segmental and whole-body spectroscopy. The details on BIA technologies, physics and methods can be found elsewhere [102–104]. The main difference between BIA methods includes the fact that whole-body single frequency BIA (SF-BIA) measures the resistance, the reactance, the impedance and the phase angle to calculate ECW and TBW, while whole-body multifrequency bioimpedance spectroscopy (MF-BIS) calculates extracellular and intracellular volumes, providing ratios (ECW to TBW, ECW to ICW) [105]. The SF-BIA is based on a regression model, while MF-BIS is based on the Cole-Cole equation that is dependent on measurements performed in healthy control subjects, allowing, at the same time, correction for cofounder parameters such as gender and age [103–105]. The segmental bioimpedance measurement is providing additional information and is considered more accurate since it allows the calculation of body composition parameters in each segment and the results are dependable on a MRI comparable model data [103, 105].
BIA measurements were validated in healthy and renal populations by different ‘gold-standard methods for fluid status assessment: blood volume monitoring (BVM) and by isotope dilution methods [106, 107]. The direct isotope dilution methods include: deuterium oxide (D2O) for the measurement of TBW, bromide dilution (Br) for the measurement of ECV and total body K (TBK) for the measurement of ICV; nevertheless, there are some data stating that these direct methods and their ‘confirmation’ relationship to BIA are not free from bias [107]. A recent study aimed to compare the precision and accuracy of SF-BIA and MF-BIS to direct estimation measurements (DEM) of TBW, ECV and ICV in 49 HD patients. The authors found that estimation of TBWSF-BIAin comparison to TBWMF-BIS and TBWDEM did not significantly differ; the same results were found for ECVDEM and ECVMF-BIS, but with a statistically significant difference between ECVSF-BIA and ECVDEM, suggesting that evaluation of ECV with MF-BIS is more accurate than using SF-BIA. This study, also, compared the overall DEM results of VO parameters with the indirect estimation methods (IEM) and concluded that, in dialysis patients, there is no real ‘gold standard’ [105].
BIA is a useful tool for fluid management in pre-dialysis CKD patients. There are several studies that are suggesting the importance of BIA in these patients for a better estimation of overhydration and improvement in clinical management [6, 7, 12, 108].
In 84 patients with mild to severe CKD, TBWSF-BIA was significantly higher than in healthy control subjects and this method was proven to be an efficient clinical tool to assess impairment in body composition [6]. The same results were found by Hung et al. who showed, in 338 patients with stages 3–5 CKD, that 20 % of them presented with VO ≥7 % in the absence of clinically detectable edema and concluded that BCM could be the most beneficial technique for detection of overhydrated CKD patients with clinically hidden signs [12]. In another study, ECV measured by MF-BIS was associated, in CKD patients, to renal function decline and various CV parameters [7]. Verdalles et al. aimed, in 50 CKD patients with resistant hypertension, to evaluate fluid status (using the BCM® device) and assess whether this method could be useful in controlling BP and diuretic treatment. The ECV expansion was observed in 60 % of the included patients; after 6 months of follow-up, BIS-guided fluid management lead, after an intensified diuretic treatment, to a decrease of TBW and SBP, with a GFR that remained stable [108].
Independently of the different BIA techniques, different targets and various protocols used, BIA-guided fluid management appears to be clearly associated with improved surrogate and hard end-points.
In dialysis patients, BIA was associated with: better management of fluid status [109–112]; improved BP control [11, 109, 111, 113]; improved arterial stiffness and other CV parameters [109, 111] and better survival both in HD and PD patients [11, 110, 114].
Wizemann et al. found that OH (defined as ECV/TBW cut-off value of >15 % or a VO >2.5 L) is an independent factor of mortality (HR = 2.1) and this value has become standard reference for VO definition in dialysis patients [13]. Measurement of VO status by BIA and measurement of before-HD values of systolic BP allow a classification of these patients into five categories [115]: Group I: VO >2.5 L and SBP >140 mmHg; Group II: VO <1 L and SBP >150 mmHg; Group III: under hydrated patients with normal-low SBP (<140 mmHg); Group IV: VO >2.5 L and SBP <140 mmHg; Group V: VO between 1 L and 2.5 L + SBP between 100 and 150 mmHg; this group is associated, in general, with healthy subjects or dialysis patients with well-managed VO.
Data provided by an analysis of 1,500 HD patients in 22 European centers showed that >25 % of these patients present with VO >2.5 L [116]. Therefore, the necessity for an objective and effective technique for the measurement and management of fluid status has become imperative. Current data is supporting BIA as a promising tool for the adequate assessment of DW in RRT patients [9, 106, 117].
Moissl et al. conducted the first trial that combined active BIS-guided fluid management with time-averaged fluid overload (TAFO) targets, in 56 HD patients, aiming to assess the feasibility and clinical consequences of this integrated technique for fluid management [106]. TAFO represents the average cardiovascular fluid overload over 1 week, assuming a linear fluid accumulation during the inter-dialysis period. The results showed that active fluid management with bioimpedance lead to a decrease in TAFO and BP for the patients in the VO group. At the end of the study, 86 % of all patients were either on TAFO target or closer than at baseline [106].
There are only two hard end points-RCT that evaluated the usefulness of BIS in fluid status management [109, 111]. The first one, conducted by a Turkish team, randomized 156 HD patients, with BIA measurements and clinical assessment of DW, into two groups: in the intervention group, the treating physician received information about fluid status and used it to adjust HD-fluid removal, whereas in the control group, DW was adjusted only by usual clinical practice. In the intervention group TAFO was significantly decreased in comparison with the control group, LVMI regressed from 131 ± 36 to 116 ± 29 g/m, in comparison to the control group where it remained the same and, also, other cardiovascular parameters were improved [111]. The second trial, performed by a Romanian team, showed in 131 HD patients that received DW prescription based on fluid management guided by BCM or by clinical judgment that, after a follow-up period of 2.5 years, the bioimpedance group showed a higher decline in arterial stiffness, SBP and a better control of VO [109].
Finally, a new bioimpedance technique was proposed for accuracy continuous monitoring of ECV during HD sessions – calf BIS (cBIS). This method is based on the hypothesis that, due to gravity, the lower limb is more likely to present an ECV expansion than the arms or trunk and, therefore, the calf could represent the last fluid feeding capital for the intravascular compartment during ultrafiltration [118]. During ultrafiltration, the extracellular resistance of the calf (cRE) increases until all fluid accumulation is completely removed and the bioimpedance parameters would not change (further increase), even if ultrafiltration still continues [119]. A very small study (N = 21 patients) compared the DW determined by cBIS and normal hydration weight predicted by whole-body BIS (NHWWBM). From the 21 included patients, 12 patients did not reach DWcBIS. Changes of whole-body ECV using whole-body BIS were more accurate in the DWcBIS group than in the non-DWcBIS group [120]. A new, also small study, that is actually an opening base for future research, showed that the use of cBIS for reaching DW in HD patients could, in fact, translate into a reduction of LV mass and BP in these patients, improving, therefore, cardiovascular outcomes [119].
The BOCOMO study is a multicenter, prospective RCT that will enroll 1,300 participants from 16 clinical facilities with the objective of evaluate the effects of BIA-guided fluid management compared to standard care for a minimum follow-up period of 36 months. The primary outcome will be a composite end point of death, acute myocardial infarction, stroke or incident peripheral arterial occlusive disease and the secondary outcomes will include LV wall thickness, BP, medication and hospitalization parameters [121].
Blood Volume Monitoring (BVM) and Volume Overload
This technique uses relative blood volume (RBV) monitoring devices incorporated in the dialysis machine that allow non-invasive, real-time assessment of intra-dialysis changes of hemoglobin/hematocrit concentrations (by optical absorbance) or of the concentration of total plasma protein (by ultrasound recorded blood waves velocities) [102, 122].
The BVM method has been proposed for predicting DW but was, initially, conceived for a better management of intra-dialysis hypotension [123–125]. However, there are several reports that contradict the usefulness of BVM for the management of fluid status and overall hemodynamic stability [126–128].
RBV monitoring offers information on RBV changes, but no data about the absolute blood volume and the interstitial hydration [119, 122]. Accordingly, this technique is not able to differentiate between the actual DW and excessive ultrafiltration rate (UFR) in relation to plasma refilling rate [119].
Lopot et al. found that flat intradialytic relative plasma volumes (RPV) slopes are correlated with VO in HD patients [129]. The DRIP study showed that HD patients with flat RPV slopes at baseline are overhydrated and that these patients had a higher decrease in interdialytic SBP upon probing DW [102, 130, 131]. In contrast, Reddan et al. found in the CLIMB study that interdialytic BVM was associated with higher hospitalization and mortality than for conventional monitoring patients, with no advantage for BVM regarding dialysis-associated complications or need for physician’s intervention [132]. Finally, Seibert et al. showed that combining BVM with cBIS may prove to be more useful in assessing the relationship between plasma refilling and tissue hydration during the HD session [119].
Hecking et al. are conducting a multicenter, prospective, triple-arm, parallel-group, crossover RCT to test the hypothesis that, in comparison to conventional HD (CHD), BVM-regulation of UF and dialysate conductivity (UCR) and/or regulation of UF and temperature (UTR) will decrease the complications that appear when UF volumes are systematically increased in volume overloaded HD patients [133]. The patients will be randomized 1:1:1 into UCR, UTR and CHD. The primary end-point will be a comparison between intra- and post-dialysis complications between groups, and, in addition, changes in relative weight reduction, residual renal function, quality of life and pre-dialysis laboratory parameters will be, also, assessed as secondary outcomes at the beginning and at the end of study [133].
Ultrasonography of Extravascular Lung Water (ELW) in CKD
ELW represents the water contained by the lung interstitium and it depends on the ventricular filling pressure of the LV [179]. Lung ultrasound (LUS) data of this compartment translates into ultrasound B-lines (BL-US), known also as lung comets. This method has been validated by CT scans, thermodilution with Swan-Ganz catheterization, natriuretic peptides levels and echocardiographic measurements [134–138]. LUS has been found to be a useful tool for risk stratification and fluid management of ICU patients, evaluation of lung water (LW) in HF patients, or acute respiratory failure patients and for the diagnosis of alveolar-interstitial syndrome [135, 139–141]. More than that, it seems that it is an excellent method for differential diagnostic between HF and COPD, with a sensitivity of 85.7–100 % and a specificity of 92–97.7 % [135, 142, 143].
Recently assessment of ELW by LUS has been proposed as an important and accurate mean of evaluation and fluid management guidance in HD patients [131, 142, 144, 145]. Mallamaci et al. found in 75 HD patients that 47 of them presented with LUS-evaluated severe lung congestion before HD and that this finding was observed in both symptomatic and asymptomatic HF patients [144]. In this study, LW excess was not associated with hydration status, but was strongly correlated with NYHA functional status, LV ejection fraction (LVEF), LA volume and pulmonary pressure; in a multiple regression model that included traditional and non-traditional risk factors, only LVEF maintained an independent association with LW excess. The authors concluded that this method is a reliable technique to detect pulmonary congestion at a pre-clinical stage in HD patients [144]. Consistent with these findings, Zoccali et al. wanted to test the prognostic value of LUS evaluation of ELW as a predictor for cardiac events and mortality in ESRD patients. The study, conducted in 392 HD patients, found that 45 % of them had moderate to severe lung congestion, 14 % severe lung congestion and that this latter group presented a 4.2-fold risk of death and a 3.2-fold risk of cardiac events in comparison to the first group. The results proved BL-US score as a strong, independent death and cardiac events predictor in HD patients [131].
Noble et al. assessed the dynamics of BL-US in 40 HD patients that underwent three LUS evaluations: the first one – before the start of HD session, the second one at the halfway into the HD session and the third one at the end of HD session [142]. This evaluation allowed the demonstration that B-lines change in real-time as fluid is being removed from the body. This fact could contribute to the importance of this method in assessing real-time changes of ECW during HD session and its usefulness as a fluid management guidance tool [142].
Siriopol et al. conducted recently an innovative, prospective observational study in 96 HD patients and compared, for the first time, three different strategies to predict mortality in this population [145]. The patients were analyzed, pre- and post-dialysis, by LUS, MF-BIS and echocardiographic parameters. The pre-dialysis ultrasound lung congestion score was strongly associated with all of the MF-BIS measurements, but, only the BL-US score had a significant discriminator power for survival. The BL-US score was found as the best predictor for the hydration status-mortality relationship, independently of MF-BIS-derived parameters [145].
Heart Rate Variability (HRV) and Volume Overload in CKD
Autonomic nervous system (ANS) dysfunction occurs in approximately half of all ESRD patients [148]. It has been demonstrated that these patients have a withdrawal in parasympathetic modulation of heart rate and an increase of the sympathetic input to the sinoatrial node [149]. Uremic toxins seem to be implicated in the genesis of cardiovascular dysautonomy, condition that is associated with dialysis hypotension, arrhythmias and sudden death [148]. The susceptibility of HD patients to cardiac dysfunction has been attributed to several factors: myocardial ischemia, LVH/LVD, serum electrolyte changes, VO and fluid removal during the dialysis session, OSA, the sympatho-vagal imbalance and dialysis-techniques related factors, all of which have an impact in cardiac reactivity – a pivotal parameter for the maintenance of hemodynamic stability during HD [10, 115, 148, 149].
HRV is a simple, non-invasive technique used to evaluate cardiac dysautonomy in healthy individuals as well as in pathological settings [150–153]. The HRV measurement provides data about the sympathetic and parasympathetic activity and reflects the ability of the sinoatrial node to modify the heart rate [10, 115]. In a simple manner, it measures the periodic variations in R-R interval from beat to beat [148].
The association between HRV and fluid overload is supported by several findings and hypothesis:
cardiac hypertrophy is associated with HRV modifications and volume overloaded patients have a decreased HRV due to cardiac hypertrophy [115]
ANS is affected by variations in central volumes [10]
the sympathetic activation observed in renal patients is influenced both by a decrease in the LV filling and by fluid status [10, 154]
The most commonly used and validated techniques for evaluation of HRV include time domain and spectral domain analysis. The time domain analysis includes the measurement of multiple statistical calculated parameters of the R-R interval duration and its variation over time (minimum, maximum, average and SD) [115, 148]. The spectral domain analysis estimates the sympathetic-vagal influence of the HRV through frequency domain analysis. It uses high-frequencies (0.15–0.40 Hz) that correlate respiratory-driven vagal efferent input to the sinoatrial node and low-frequencies, that correlate with parasympathetic and sympathetic activity and baroreceptors-mediated BP control [149].
HRV changes were described in HD patients and were associated with hematocrit, body mass index, HD duration, LVH and ischemic heart disease [115]. HRV was found to be an independent predictor of mortality in 383 HD patients with 24-h ambulatory electrocardiographic records and time and frequency domains analysis [156].
A recent trial was the first one to analyze the association between VO and ANS dysfunction in 69 HD patients. VO was evaluated by whole-body BIS before the start of the midweek HD session. Also, at the same time, a 24-h HolterECG was started. Even though this is small, observational study, their results are the first to demonstrate a correlation between reduced HRV and higher values of VO in these patients [10]. A 2013 study aimed to compare the effectiveness of various measurement methods of fluid status for an adequate estimation of DW [157]. Thus, 30 HD patients underwent BIS, BL-US score, BNP and IVC diameter measurements, that were further analyzed by two nephrologists. All methods, except for evaluation of IVC collapse index after the end of the HD session, were able to assess overhydration before and after HD. In terms of evaluating fluid status, BL-US correlated better with BIS than IVC diameter and the authors concluded that BL-US could provide real-time evaluation of fluid status [157].
Volume Overload-Consequences in CKD Patients
VO and Cardiovascular Disease (CVD)
Renal patients present a higher risk for the development and progression of cardiovascular disease (CVD) due to an increased prevalence of traditional risk factors (older age, male gender, hypertension, dyslipidemia, DM, LVH), but also due to non-traditional factors (albuminuria, anemia, hyperparathyroidism, ECV overload, oxidative stress, inflammation, malnutrition) [71]. The severity and incidence of CAD is higher with the reduction of the glomerular filtration rate (GFR) and CV morbidity and mortality are increased with impaired renal function (especially when GFR <15 ml/min/1.73 m2) [158]. Similarly, the risk of CHF is doubled in patients with a GFR <60 ml/min/1.73 m2) [159]. Two studies [160, 161], one from Canada and the second one from Taiwan investigated on a large scale the CV risk associated with CKD fluid overload is an important risk factor for CVD in CKD patients. Recently, Hung et al. reported in 338 patients with stages 3–5 CKD, that volume overload was strongly associated with both traditional and novel risk factors for cardiovascular disease in a multivariate analysis (male sex, diabetes, pre-existing cardiovascular disease, systolic blood pressure, serum albumin, TNF-α, and proteinuria) [12].
VO, Sodium and Heart Failure in CKD
VO is the most important mechanism leading to decompensated HF [162]. When kidney function is impaired or in chronic HF associated with renal hypo-perfusion, the kidney ability to excrete sodium is exceeded, salt sensitivity increases, the sodium-effect of aldosterone is reduced and other conditions, such as renal resistance to natriuretic peptides and non-osmotic release of ADH, appear [1, 68, 163]. In the initial phases, the etiologic factors induced by HF are represented by arterial under-filling and venous congestion; these are further translated into compensatory mechanisms [1]. First, the decreased distension of arterial baroreceptors during arterial under-filling determines the activation of SNS, RAAS and non-osmotic release of vasopressin, leading to a decreased water and Na excretion [1, 163]. The response is represented by vasodilatation with natriuretic peptide release, activation of the kinin-kalikrein system and expression of endothelial relaxation factor, for an increased water and Na excretion [1]. These compensatory adaptive mechanisms eventually become maladaptive, further contributing to VO [162, 163]. There is still a paucity of data assessing the importance of VO as a risk factor for poor clinical outcomes in patients with CKD and CVD, as renal patients are an underrepresented subgroup population in CHF randomized controlled trials (RCT).
Recently, a direct and strong association between plasma Na and LA volume (LAV) was described in asymptomatic patients with 3–5 stages CKD [164]. The plasma Na had an important impact on the variability of LAV, only second to LVM, LV volume and age. Plasma Na was found to be a predictor of approx. 16 % of the total variance in LAV, independently of LVM and LV volume. Also, the study showed a very high prevalence (41.8 %) of subclinical LA enlargement that was not associated with a worsening GFR [164, 165]. Mallamaci et al. performed a subsequent analysis on this study and found that a-2 mmol/L increase in plasma Na is responsible for a 1 ml/m2] increase in LAV [165].
VO, Hypertension and Left Ventricular Hypertrophy
The relationship between VO and BP was evaluated in several studies [9, 110, 166]. In predialysis patients, Verdalles et al. used bioimpedance to assess fluid status and to guide diuretic therapy for treating hypertension in these patients. Thirty patients with extracellular volume (ECV) expansion and a diuretic were compared to 20 patients without ECV expansion who instead received another additional antihypertensive medication. At 6 months of follow-up, SBP decreased by 21 mmHg in patients with ECV expansion compared to 9 mmHg in patients with normal ECW (P < 0.01). In addition, more patients achieved the target BP of less than 140/90 mmHg at 6 months in the group with ECV expansion (nine of 30 patients with ECV expansion cf. two of 20 without ECV expansion).
Based on bioimpedance and cuff BP measurements, Wabel et al. described four distinct categories of individuals in dialysis: (i) normotensive – normovolemics; (ii) hypertensive – normovolemics; (iii) hypertensive – hypervolemics; (iv) normotensive –hypervolemics [110]. It is clear that BP management by different classes of drugs could be tailored much easier and related to prevailing underlying pathophysiological mechanisms. Similarly, our group found in 160 HD patients, using multi-frequency body impedance spectroscopy (MF-BIS) measurements, a large group of hypertensive patients with VO patients, despite an apparently achieved dry weight (DW) on clinical evaluation. Therefore, body composition monitoring (BCM) could represent an accurate measurement tool for the adequate management of BP and risk stratification in HD patients, providing accurate information of more abnormal cardiac and vascular profiles [9]. Similarly, in CKD predialysis and in dialysis patients, the inadequate evaluation of OH and its association with LVH have been established for a long time [9, 13, 109]. Essig et al. investigated 104 patients with early CKD followed-up for 5 years, in order to evaluate body composition, cardiac alterations and their association with GFR. The study showed that ECV was an independent predictor of LVH [7]. Moreover, the correction of the FO could be associated with an improvement of hypertension and left ventricular hypertrophy. Hur et al. in a recent RCT perform bioimpedance spectroscopy in 156 patients; at half of them, fluid removal during dialysis was adjusted based on bioimpedance measurement. After 1 year of follow-up, a substantial regression of the left ventricular mass index (mean difference between groups −10.2 g/m [2]; P < 0.001) and blood pressure was noted in the interventional group but not in the control group [111]. Also in PD patients, FO was associated with hypertension and LVH. Cader et al. aimed to assess the prevalence and LVH and its determinants in a cross-sectional observational study that enrolled 31 stable asymptomatic PD patients. OH was identified as a predictor of higher LV mass index (LVMI) and, on multivariate analysis, was the main predictor of LVH in these patients [167].
FO and Arterial Stiffness
Arterial stiffness is best described by the viscoelastic property of the arterial wall that represents the relationship between pressure response and changes in volume [168]. The principal factors that alter the arterial wall are classified as stable factors (e.g. atherosclerosis) and dynamic hemodynamic changes (e.g. BP) [168].
In ESRD patients, the aorta, the common carotid artery and large arteries are enlarged in comparison to age-, sex- and BP-matched control subjects [169]. GusbethTatomir – Covic et al. found an association between VO (a common status in ESRD patients) and arterial stiffness [170], results that were confirmed by similar findings [65, 171]. Furthermore, an improvement in arterial stiffness was associated with a better survival in dialysis patients [172]. In these patients, chronic VO produces the proper conditions for arterial remodeling as a response to an increased wall stress, determining a thickening of the arterial intima-media [173, 174]. The increase in the arterial intima-media thickness (IMT) will further lead to a decreased arterial distension, an increased pulse wave velocity (PWV) and an early return of wave reflection [173, 175]. LVH in ESRD patients develops as a combined consequence of both non-hemodynamic uremic factors and of hemodynamic overload, the latter being the effect of flow and pressure overload [173]. Flow overload determines the enlargement of LV and it is caused by anemia, arteriovenous fistulas and chronic VO; pressure overload is associated with structural and functional modifications of large arteries including stiffening of the arterial tree. At the same time, the arterial tree is also suffering remodeling from the flow overload burden. It is evident that cardiac and arterial changes are the consequence of the same hemodynamic abnormalities [173].
Regarding the decrease/amelioration of arterial stiffness by improving VO with HD, the literature provides conflicting data. Ie et al. combined carotid pulse contour analysis with aortic outflow measurement and found that aortic compliance was improved by HD fluid removal in patients with already achieved DW and steady BP although PWV did not differ after the HD session, suggesting that arterial stiffness in HD patients could be explained by a reversal reduction of aortic compliance due to volume expansion [176]. Another study measured PWV in 19 HD patients before and 24 h after they had achieved post-HD DW. The results failed to associate an improvement in PWV after volume reduction; this correlation was highlighted only after adding angiotensin converting enzyme inhibitors (ACEI) treatment for 1 week in a subgroup of ten patients, suggesting that the contribution of angiotensin II to arterial stiffness [177]. A more recent study demonstrated, in the same clinical settings, that HD significantly reduced PWV from pre-HD to post-HD [178]. These later findings were in addition supported by a study conducted by the same group that evaluated the long-term association of VO with arterial stiffness. PWV was measured at baseline, at the end of the intervention period (2.5 years) and at the end of the study (3.5 years) and was analyzed in correlation with VO status assessed by clinical methods and BIA. The results showed significant difference in PWV between the groups in relationship to VO, with PWV decrease in the BIA-managed group and a significant increase in the clinical-managed group, underlying the advantage of an adequate fluid status management in arterial stiffness improvement [109].
The newest information is provided by a very recent study that investigated for the first time the effect of different HD techniques on arterial stiffness. The authors aimed for a comparative evaluation between hemodialysis and hemodiafiltration on PWV, wave reflections, and central hemodynamic parameters. The results showed a decrease in the augmentation index (AIx) by both methods. Also, the aortic and brachial PWV were not modified by any of the two HD methods [179].
FO and Obstructive Sleep Apnea (OSA)
The presence of OSA in ESRD patients was associated with hypertension, LVH and increased mortality [180–182]. OSA presents a higher prevalence in patients that have VO status, such as CHF and ESRD in comparison to the general population [183–185]. The hypothesized mechanism is that the fluid overload status, so common in these patients, could increase the amount of fluid displaced from the legs into the neck during nighttime, leading to a compression of the upper airways. Indeed, it has been demonstrated that OSA in ESRD is ameliorated with conversion from conventional to nocturnal HD or conversion from conventional ambulatory PD to nocturnal- cycler-assisted PD [186, 187].
The proposed mechanism is supported by data from several studies. In a cohort of 26 ESRD patients studied throughout polysomnography, with BIA and neck circumference measurements it was found that nocturnal rostral fluid shift is indeed associated with OSA [188]. Another cohort of 20 patients was evaluated with polysomnography, leg fluid volume measurement and magnetic resonance imaging (MRI) of the upper airway. It was found that VO, through increased internal jugular vein volumes and increased amount of mucosal water is a contributing factor in the pathogenesis of OSA in ESRD patients [189].
FO and CKD Progression
Tsai et al. published in 2014 the results of a prospective cohort study designed to assess the association of VO to CKD progression [14]. The study enrolled 472 CKD stages 4–5 patients that were followed-up for a median 17.3 months period; 39.6 % had a rapid GFR decline while 15 % reached ESRD and started dialysis. The study showed that VO was independently associated with an increased risk of rapid GFR decline and that the severity of VO was associated with an increased risk of RRT initiation [14]. The same group further investigated the enrolled 207/472 diabetic patients [8]. In this subsequent analysis, the authors reported that TBW, ECW and ECW/TBW were higher in diabetic patients with VO than in those who had only CKD + DM or only CKD + VO. In a multivariate analysis of progression to RRT initiation, a significant association between DM and VO was shown. Also, the decline in renal function was significantly more rapid in patients with VO than in patients without VO, independently of DM presence. The authors concluded that VO has a higher predictive value for an increased risk of CKD progression than DM in late CKD [8]. A modest, inverse relationship between OH and GFR was also observed by Hung et al. in 338 stages 3–5 CKD patients, but in a multivariate analysis, this correlation was no longer noted [12].
FO and Survival
FO, FO-determinants and FO-consequences are associated, in prospective observational studies [2, 3, 11, 65, 131, 145, 171, 190–192] and in RCTs [109], with an increased risk of mortality among renal patients. Poor volume control is mainly related to at least three factors (i) high interdialytic weight gain (IDWG); (ii) high ultrafiltration rate (UFR) causing as a consequence hypotension and (iii) chronic volume overload.
The association between higher IDWG and poor survival in HD patients was found in 11.142 USRDS database patients in which, pre- and post-dialysis BP values (by wide pulse pressures implication) were independently associated with mortality [171]. An analysis of DOPPS data showed that HD-nondherence lead to higher IDWG and increased risk of hospitalization and mortality. Comorbidities and lower dialysis dose were also associated with higher mortality risk [65]. In a prospective observational cohort study on 34,107 HD patients, the association between higher IDWG and all-cause mortality was analyzed. The comparison groups were 4,900 HD patients with IDWG between 0.5 and 1.5 kg and 29,207 HD patients with IDWG >1.5 kg. Higher IDWG was found in younger patients, in a greater percentage of males, with DM, higher BMI, protein intake, serum albumin levels, serum creatinine and phosphorus. Lower IDEG was more likely in females and older age patients. DM was associated with 94 % higher risk of VO and dialysis vintage >5 years with 67 % higher risk of VO. For the IDWG-survival analysis, 8 a priori defined increments of VO were created: seven 0.5 kg increments between 0.5 and 4.0 kg and the group with IDWG >4 kg. The 1.5–2.0 kg group was used as reference. When controlled for demographics and case-mix covariates, an IDWG >3.0 kg was associated with increased risk of death. A-2 consecutive IDWG >4.0 kg was associated with 25 % risk of CV mortality and 28 % risk of death, but minimal fluid retention (0.5–1.0 kg) was correlated with 26 % higher chance of survival and 23 % lower CV mortality. Mortality risk of higher IDWG (>1.5 kg) was a constant finding even in subgroup analysis [2].
High UFR could induce haemodynamic instability, intradialytic hypotension (IDH) and increased mortality risk [193]. Hypotension during HD and aggressive UFR increase the risk for intradialytic recurrent myocardial stunning and could determine, over time, irreversible fibrotic changes and chronic heart failure, arrhythmias and sudden cardiac death [194]. In 70 prevalent HD patients without severe left ventricular dysfunction, Burton et al. performed serial echocardiography during and at 30 min following HD [195] and found in 64 % of the patients, a significant reduction in cardiac function—the only predictors were the ultrafiltration volume and IDH. Shoji et al. found a 2-year mortality rate approximately 8 % higher for patients with intradialytic BP <110/59 mmHg [196] and Tisler et al. observed that patients with frequent IDH have a 25 % reduction in life expectancy in comparison with hypotension-resistant patients [197]. Agarwal et al. found in 308 HD patients an independent (from conventional and non-conventional CV risk factors, UF volume and rate) prognostic relationship of RPV slopes and mortality. Compared to steeper RPV slope, a flatter RPV slope was associated with 1.72 higher hazard of mortality [3].
Chronic volume overload and survival – Paniagua et al. showed in a prospective multicenter cohort of 753 prevalent adult patients on CAPD, APD and HD followed up for 16 months that, on a multivariate analysis, NT-proBNP levels and ECW/TBW were predictors of both all-cause and cardiovascular mortality, independently of dialysis modality and the presence of other known clinical and biochemical risk factors [198]. In 2012, Chazot et al. compared 50 selected (normohydrated) HD patients from Tassin, France to 158 patients from Giessen, Germany. The Dutch patients were divided into two groups: non-overhydrated (123 patients) and overhydrated (35 patients). After 6.5 years of follow-up, multivariate adjusted all-cause mortality was significantly increased in the overhydrated group (HR = 3.41), suggesting that VO is an important predictor for all-cause mortality in HD patients [11]. Siriopol et al. showed in 96 HD patients evaluated by three different methods of fluid status assessment (LUS, BIA and echocardiography measurements) the significant prognostic power for survival of BL-US performed before the start of HD session. After an observation period of 405.5 days, HR for mortality was higher in the group with severe lung congestion (UL comets >30) compared with the other two groups (HR = 5.03, 95 % CI: 1.5–16.5). In order to assess the real impact of lung water on survival, the investigators controlled in a multivariate Cox model for demographic, echocardiographic and BIA factors. LVMI and pre-HD BL-US score were survival predictors that maintained a statistical significance after adjustment [145].
A 2014 single-center retrospective analysis of prospectively collected data of 529 PD patients found that OH index (OH and OH/ECW) was an independent predictor of mortality in a multivariate analysis. The 30 % of patients that were most severely overhydrated as defined by ECW/TBW had the highest increased adjusted risk of death = 2.05 (95 % CI: 1.31–3.22, P < 0.005) [192].
The impact of VO may be modulated by the impact/interaction with aldosterone. In a 2013 study, Hung et al. aimed to investigate if the association of aldosterone levels with mortality is modified by the presence of VO in 328 HD patients followed for 54 months. The investigators found the following: (a) baseline aldosterone was significantly lower in the presence of VO than in its absence; (b) during follow-up, higher aldosterone levels and VO were associated with decreased HR for mortality and cardiovascular events; (c) in contrast, in the absence of VO, higher levels of aldosterone were associated with increased risk for mortality and cardiovascular events. The authors concluded that the association between aldosterone levels and adverse outcomes in HD patients is modulated by the confounding effect of VO and that their results support the treatment of hyperaldosteronism in HD normovolemic patients [5].
Volume Overload Treatment: Sodium Plays the ‘Leading Role’
Non-renal Patients
In non-renal population, the association between salt intake or sodium excretion OH, BP and different CV outcomes has been extensively studied, although with disparate results, depending on multiple factors, such as different characteristics of the included populations, different assessment protocols of salt intake, poor quality of measurement methods of urinary Na excretion and various intervention strategies.
INTERSALT, a multi-national epidemiological trial, conducted in 10,074 normotensive and hypertensive patients across 32 countries, reported that each 2.3 g increase in 24-h urinary Na excretion was associated with a 6/3 mmHg increase in BP [199]. Patients included in the PREVEND study had a 6 % higher risk of developing HTN for each 2.3 g increase in salt intake [200]. The DASH RCT used a controlled diet (low on fruits, vegetables and dairy and rich on fats) and randomized the participants into three categories, to a low, medium and high Na intake for 30 days. A decrease in Na intake from 3.2 to 2.4 g was associated with a SBP reduction of 2.1 mmHg [201]. The TOHP I and II studies found that a decrease of Na intake to 44 mmol/24 h and respectively to 33 mmol/24 h is associated with a 25 % reduction CV events, suggesting an advantage for a moderate salt reduction in the general population [202]. These results are consistent with the American Heart Association (AHA) recommendations [203]. In addition, reducing Na intake in patients experiencing resistant HTN, is associated with a decreased SBP and DBP suggesting that a high Na intake is an important determinant of resistance to antihypertensive treatment [204]. Also, the contributing effect of high Na intake on arterial stiffness and endothelial vascular function was reported in several studies [200, 205–208].
In contrast to these positive findings, a recent meta-analysis of seven clinical trials, aiming to evaluate the efficacy of Na intake reduction on BP and BP-driven hard end points, failed to found an association to a reduced CVD risk or mortality risk [209]. More importantly, there is data supporting the ‘U-shaped’ optimum salt intake and the ‘J-shaped’ association between 24-h urinary Na excretion and cardiovascular events.
O’Donnel et al. showed, in a posthoc analysis of the ONTARGET and TRANSCEND cohorts, that a higher 24-h urinary Na excretion is associated with a higher risk of cardiovascular events. The cardiovascular mortality risk was 9.7 % higher with an estimated Na intake of 7–8 g/24 h and 11.2 % for an estimated Na intake of >8 g/24 h. Nevertheless, a paradoxical inverse relationship was found in 12 % of participants at an estimated Na urinary excretion of <3,000 mg/24 h [210]. The FinnDiane study, reported as well a ‘J-shaped’ relationship between urinary Na excretion and cardiovascular events [211]. An inverse relationship between urinary Na excretion and CVD and all-cause mortality was noted in several other studies [212, 213].
Pre-dialysis CKD Patients
In pre-dialysis CKD patients, excess Na intake – as a risk factor determines VO increased BP and proteinuria. Therefore, controlling salt intake could account for a simple, modifiable measure for reduction of VO, BP and proteinuria, the principal conditions leading to CKD progression [214, 215]. In CKD patients, a Na intake >4.6 g/day, compared to patients with a Na intake of <2.3 g/day was associated with renal function decline and increased proteinuria [216]. A double-blind placebo RCT determined the effects of modest salt reduction intake on 24-h urinary albumin excretion rate and on PWV in 69 patients with mild-untreated HTN, followed-up for 6 weeks. The results showed that a 55 mmol (3.2 g salt) reduction in Na cf. to placebo determined a significant decrease in BP, urinary albumin, albumin/creatinine ratio and PWV [217].
In a RCT that enrolled 52 non-diabetic, hypertensive CKD patients the effect of Na dietary restriction was compared to angiotensin receptor blockade at maximum dose or RAAS dual blockade. The authors concluded that dietary Na restriction, as recommended by guidelines, is more effective for BP and proteinuria control than the RAAS dual blockade [218].
Reports show that 80–89 % of CKD patients ingest >100 mmol Na/day, a higher amount than what the guidelines are recommending [219–222]. Thus, the most important therapeutic strategy is reducing salt intake, but this is a challenging task due to patient’s poor adherence to diet restriction and the difficulty in achieving an unsalted diet, as the common western processed food contains high amounts of Na [215, 219–221].
A very recent RCT aimed to assess the effects of high versus low Na intake on ambulatory BP, 24-h protein and albumin urinary excretion, fluid status, renin and aldosterone levels and arterial stiffness in 20 stage 3–4 CKD hypertensive patients [223]. This trial represents, in fact, the first phase of the LowSALT CKD study, which is a 6 week randomized-crossover trial evaluating the impact of low Na intake (60 mmol/day) versus moderate Na intake (180 mmol/day) on cardiovascular risk factors and renal function decline in mild-moderate CKD. The phase II of this trial will assess the longer-term effectiveness of Na restriction and will continue the investigations initiated in phase I, with addition of patient-centered outcomes (dietary adherence, quality of life and taste assessment) [224]. The study phase I showed statistically significant and clinically important reductions in: BP (mean reduction of SBP/DBP of 10/4 mmHg), extracellular fluid volume, albuminuria, and proteinuria when a low Na intake was applied. These changes were more pronounced than those observed in patients without CKD, suggesting that patients with CKD are particularly salt sensitive [223].
Beside Na intake, water intake has been previously evaluated, in small, underpowered studies, for its relationship to cardiovascular outcomes and impact on kidney function [225–228]. Palmer et al. investigated in a longitudinal cohort study the association between fluid intake, kidney function and long-term mortality [229]. The study included 3,858 participants identified from 1992 to 1994 and from 1999 to 2000 by a door-to-door census of all residents in two urban postcode areas. The subjects completed a self-administered food frequency questionnaire that assessed the total fluid intake from food and other beverages beside water; further, participants were divided into quartiles corresponding to water consumption, as follows: <2 L/day. 2.0–2.4, 2.5–3.0 and >3 L/day; each subject participated in a detailed medical examination at baseline and during follow-up. The statistical analysis for all-cause and cardiovascular mortality did not found a significant correlation between these outcomes and fluid intake, on a median follow-up period of 13.1 years. A total of 1,127 death were recorded with 580 cardiovascular deaths, but the controlled analysis for clinical and demographic parameters did not found an association between daily fluid intake and all-cause mortality per 250 ml/increase [229]. Also, kidney function was evaluated by repeated measurements of serum creatinine over a 10-year follow-up period in 1,479 participants and from these, 1,207 were included for the final analysis; there was no statistically significant association between daily fluid consumption and changes in GFR [229]. These results do not support the international guidelines recommendations of a beneficial impact for the kidney when eight glasses of water/day are consumed [230].
Renal Replacement Therapy Patients
Volume control and sodium restriction is associated with improved BP values and increased survival rates in dialysis patients. Ozkahya et al. in 218 HD patients with a salt restriction diet: mean salt intake 4–5 g/day) and no antihypertensive treatment and a mean follow-up period of 47 ± 34 months [190] showed an improvement in BP values and mortality rates. Our group performed recently a RCT in 131 HD patients, randomly assigned into two groups (BIA-analyzed group and clinical-evaluated group). After a 2.5-year period of follow-up, all-cause mortality was significantly lower in the BIA group compared to clinical-evaluated group. After adjustments for age, gender, CVD, DM, dialysis vintage, BMI, SBP, albumin level and relative fluid overload, the HR for mortality in the BIA group was 0.100 (95 % CI: 0.013–0.805, P = 0.04) compared to the clinical-evaluated group [109]. In 2012 Covic et al. analyzed the existing data supporting the hypothesis that uremic toxins are the principal determinants of morbidity and mortality in ESRD patients versus salt and VO. The final conclusion, based on recent studies, was that volume and salt-intake management are accountable for a better BP control, reduction of cardiovascular events and mortality in these patients [193].
For HD patients, Na balance and ECV control, through an established appropriate DW and normotensive status, represent the most important factor for reducing negative outcomes. Na balance in HD patients is dependent of Na intake and removal by the dialysis procedure. A positive relationship between serum Na (SNa) and elevated BP was described in several experimental and clinical settings [231–233]. A lower Na intake leads to a reduction in BP and LVH and higher Na levels are associated with both elevated BP and IDWG [190, 234, 235].
A number of proposed strategies were created over time for a better control of fluid status and BP: (1) Na profilling (modelling); (2) Na individualization; (3) UF profiling; (4) different HD techniques. These methods are further analyzed in terms of achievability, efficacy and safety.
Na Profiling ± UF Profilling
The dialysate Na (DNa) prescription is a modifiable, but underused parameter for achieving Na balance in HD patients. In HD, Na removal is achieved by convection or diffusion; under the current practice, approx. 80 % of Na is removed by convection and 20 % by diffusion [236, 237]. Hypothetically, a regular removal of 1 L of plasma water by UF, when a theoretically isotonic Na concentration of 140 mmol/L is considered in the ultrafiltrate, could remove 140 mmol of Na (8 g NaCl consumption for each interdialytic day) [236]. Diffusive Na losses take place across the dialyzer membrane in line with the diffusion gradient between plasma and dialysate, whereas the convective Na losses represents the quantity of Na removed by UF and it depends on the prescribed UFR [237]. Currently, the regular dialysate prescription is based on an isotonic Na level of 135–145 mmol/L [236]. Experiments for a better fluid control in HD patients, by altering Na prescription to a higher value of DNa concentration (>141 mmol/L) or to a lower value of DNa concentration (<137 mmol/L) have been conducted in various forms and protocols. Higher DNa prescription determines a rapid UF and the removal of fluid excess from the interdialyitic period, but, as highlighted by Davenport et al., this is not as adequate method to achieve Na balance, as is leading to hypernatremia, increased thirst and higher IDWG, higher pre- and post-dialysis SBP and an intensified antihypertensive medication [237, 238]. On the other hand, using a low DNa concentration will lead to a rapid decrease in plasma osmolality and a hemodynamic instability and intradialytic hypotension [239, 240]. Various studies advocate for the benefits of using a lower DNa prescription, whereas others emphasize the ‘dark sides’ of the complications that arise with this method.
Na profiling is a HD method developed to avoid hypotension-related discomfort during HD (IHD) by modulating DNa prescription according to pre-established profiles; it was designed to preserve the advantages of a high DNa prescription without its intradialyitic complications [241]. It usually represents the prescription of a high DNa at the beginning of the HD session in order to determinate a hyponatremic status that will facilitate the water shift from the ICV to ECV compartment; during the HD session, DNa will be progressively decreased to avoid VO [242]. Time-averaged mean of DNa is associated with intradialytic diffusion Na overload and is generally higher with Na profiling than with a fixed DNa concentration [241, 243].
UF profiling is another way to prevent IHD and it represents the intermittent interruption or progressive reduction of UFR in order to promote plasma refilling. Therefore, during the high DNa period, a high UFR could provide an increase in plasma tonicity and a higher plasma refilling; during the low DNa period, a low UFR could avoid hypovolemia [241].
Song et al. conducted a prospective study to determine the optimal Na balance by Na profiling for prevention of IHD and to investigate if such an optimal Na profiling could result in HD sessions without Na gain-related complications. In 11 HD patients, 8 treatment modalities were evaluated: conventional HD (CHD) – control group, Na balance-positive step-down profiling HD (PS), Na balance-neutral step-down profiling HD (NS), Na balance-neutral alternating Na profiling HD (NA), UF only and PS + UF, NS + UF, NA + UF. Only PS, PS + UF, NS + UF and NA + UF had a significant positive impact; these were further analyzed in a phase II, randomized controlled, 6 weeks, crossover study [241]. The results were: (a) diffusive Na gain was significantly higher with both PS and PS + UF; (b) PS and PS + UF increased IDWG; (c) PS and PS + UF increased pre-dialysis weight and the amount of UF; (d) %UF achieved targetingDW was higher with NS + UF and NA + UF; (e) post-dialysis weight as closest to DW was achieved with NS + UF and NA + UF; (f) incidence of excessive weight gain and IHD increased significantly with PS. The investigators concluded that NS + UF and NA + UF are associated with less Na and weight gain, better UF performance, fewer IHD, a hemodynamic benefit and post-dialysis weight closest to DW [241]. DOPPS, an international database of dialysis patients was analyzed in an observational study by Hecking et al. respective to SNa and DNa [244]. The study found: (a) a higher DNa is associated with lower risk of hospitalization, even though it was, also, associated with higher IDWG; (b) DNa was not associated with mortality, but neither with lower SBP values [244]. The important disadvantages of inadequate Na profiling and the unconfirmed ‘benefits’ of this method are detailed elsewhere [245, 246].
Na Individualization
Na individualization was designed as an improved alternative for the adverse effects of Na profiling. It has been hypothesized that humans have an individual Na ‘set-point’, therefore, whenever there is a Na excess, higher than the fluid intake, SNa will increase above the set-point leading to a further fluid intake [69, 247], especially in HD patients where pre-dialysis SNa tends to remain stable over time [248]. Accordingly, if a patient has a positive Na balance during HD (dialysis against a higher DNa prescription) that individual’s thirst will be stimulated and he/she will drink the amount of water necessary to drive the osmolality back to its set-point [237]. As a general concern, lower DNa is considered a potential determinant of SNa [249, 250]. Data, associating a low DNa with mortality, was previously reported, so lowering DNa for an alignment of the DNa with pre-dialysis SNa has to be pursued with caution [249, 251–253]. Also, Na individualization was associated with better surrogate and hard end points, supporting the lowering/tailoring of DNa in line with the SNaset-point, rather than using a fixed DNa concentration or DNa modelling [103, 249].
Na individualization method is well described by Arramreddy et al.’s case series of 13 patients that were switched from CHD to DNa individualization prescription. The strategy began with a phase A-stepwise weekly reduction of the standard DNa (140 Meq/L) by 2–3 mEq/L until reaching a Na gradient of −2 mEq/L. The tapering phase lasted 4 weeks. The Na gradient was defined as difference between prescribed DNa and average SNa and average SNa was calculated as mean SNa over the preceding 3 months. During the intervention period, the DNa was not further readjusted in line to monthly SNa levels. Further, on the same day that patients reached a −2 mEq/L Na gradient, they entered a 4 week-phase B-period during which all patients were dialyzed with a Na gradient of -2 mEq/L. Baseline pre-HD SNa was 135.3 ± 3.7 mEq/L; in phase A, post-HD SNa increased by 1.5 ± 3.7 mEq/L compared to pre-HD SNa. HD with an individually reduced DNa, achieving a gradient of −2 mEq/L, in phase B did not modify pre-HD SNa. Compared to standard DNa, individualized DNa reduced IDWG without significant increase in the frequency of intradialytic hypotension or cramps [254].
Hecking et al. performed two separate investigations on DOPPS trial results [244, 255]. In the first one, the investigators found an association between SNa and DNa and that this correlation influenced the mortality: DNa >140 mEq/L was associated with a trend towards higher mortality in patients with SNa ≥140 mEq/L; higher DNa was associated with lower mortality in patients with SNa <137 mEq/L [249, 255]. In the second study, the analysis revealed: (a) a large variability of DNa prescription between centers: 55 % used fixed DNa and 44 % used DNa individualization; (b) in non-individualized DNa facilities, higher DNa was associated with higher SBP, but with a lower mortality; (c) in individualized DNa facilities, higher DNa was associated with lower SBP, but with higher mortality [247, 249]. Nevertheless, it should be noted that patients with higher non-individualized DNa were younger, lees likely to have DM and had lower serum albumin. Also, as in the non-individualized centers, DNa was not prescribed in line with patient’ characteristics, there is possibility for confounding by indication [249]. The authors concluded that there is rather limited support for lowering DNa to control HTN, especially in the view of this method’s association with hospitalization risk and mortality [244]. A higher DNa could be beneficial in some patients but detrimental in others [249]. De Paula et al.’s study, on 37 HD patients that received 3 weeks of HD with DNa at 138 mEq/L and 3 weeks of HD with DNa individualized according to patient’s average pre-dialysis SNa, found that DNa individualization lead to lower SBP in patients with uncontrolled HTN, but with no impact on BP in those with controlled BP (<150/85 mmHg) [256].
Sayarlioglu et al. showed that DNa individualization is associated with better outcomes in 18 HD patients that underwent HD with DNa of 135 mEq/L when pre-HD SNa was <137 mEq/L and HD with DNa of 137 mEq/l when pre-HD SNa was >137 mEq/L. After a follow-up period of 8 weeks, lowered DNa was associated with lower pre- and post-HD SBP, lower pre-HD DBP, lower IDWG and improved cardiovascular parameters (reduction in LV systolic diameter, tricuspid regurgitation, IVC diameter and pulmonary arterial pressure) [257]. Actually, this is the first of the only two studies that analyzed the relationship between a lower DNa and cardiovascular parameters. The second study was performed by Kutlugun et al., in 30 HD patients, and showed that endothelial dysfunction, measured by flow-mediated dilatation (FMD), was significantly higher after using low DNa prescription (137 mEq/L) than after assigned standard DNa (143 mEq/L). Lower DNa resulted in a better BP and IDWG control, but no improvement in the LVM [258].
The SOLID trial is a multi-center, prospective, randomized, single-blind, controlled parallel 3 years clinical trial designed to investigate the impact of a low DNa on cardiovascular risk in HD patients. The study protocol was initiated after a previous investigation performed by the same group that showed a decrease by 4–5 mmHg in SBP and 2–3 mmHg in DBP with a 3 mmol decrease in DNa [259]. The present study intends to enroll 118 home HD patients from 6 sites for a follow-up period of 12 months; during this time the intervention and control groups will undergo HD with DNa of 135 and 140 mmol respectively. The primary outcome will be LVMI changes with other approximately 11 more secondary outcomes [240].
Non-conventional Dialysis Techniques
The virtues of more frequent dialysis on improved BP control, and even on survival are supported by results of small, observational cohort studies, as well as larger, RCTs. Prolonged dialysis schedules represent a more physiologic solute and fluid removal (a gradual UF) that can be translated into an improvement of dialysis adequacy. All of these HD methods can be performed in different locations: in-center, home-assisted, home and include: Short Daily HD (SDHD) – less than 3 h/session, 6 times/week; Long Daily HD (LDHD) – >5 h/session, 6 times/week; Nocturnal HD (NHD) – 6 to 8 h/session, 3–5 or 6 times/week.
In 1983, Charra and the Tassin group were the first to report the benefits of 3 times/week, 8 h/session, diurnal and nocturnal HD, along with reduction of salt intake on volume and BP control and survival [260–262]. Consistent with their results, similar findings were further provided by different studies using the same strategy. Beginning with 1998, studies performed in patients receiving SDHD or NHD reported improved BP, LVH, phosphorus and hemoglobin levels, decreased risk of hospitalization and even a better survival [250, 263–273].
Nocturnal Hemodialysis
Nocturnal home HD (NHHD) is associated with better outcomes, as shown in several observational, prospective studies and in RCTs: increased hemoglobin levels, reduced cardiovascular hospitalization and improved OSA [274–279].
In 2009, Johansen et al. used United States Renal Data System (USRDS) database to investigate, in a cohort study, the hospitalization and mortality rates in patients from various centers that used NHD for at least 60 days, comparing these outcomes with characteristic-matched patients on CHD. NHD was associated with significant reduction in mortality risk and risk for major morbid events [275].
Ok et al. performed in 2011 a prospective controlled study in 247 CHD patients and 247 ICNHD. Overall mortality rates were 1.77 % in ICNHD and 6.23 in CHD group/100 patient-years, after a mean 11.3 ± 4.7 months of follow-up. ICNHD was associated with 72 % risk reduction for mortality, decreased LV and LVMI end-diastolic diameters, improved cognitive function, lower serum phosphate and lower usage of phosphate binders [274].
The randomized controlled studies performed by Culleton et al. in 2007 and Rocco et al. in 2011 found conflicting results. Thus, Culleton et al. performed a 2-group, parallel RCT to compare the effects of frequent NHD (6 times/week) versus CHD on LVM and health related quality of life, for a 6 months follow-up period. Frequent NHD: significantly improved mean LVM, was associated with improvements only in selected kidney-specific domains of quality of life and a better control of SBP and mineral metabolism [250]. However, the Frequent Hemodialysis Network Nocturnal Trial (Nocturnal FHN), which randomized 45 patients in the frequent NHD arm and 42 in CHD arm, failed to demonstrate a clear superiority of this method. NHD determined a 1.82-fold higher mean weekly stdKt/Vurea, a 1.74-gold higher average number of treatments/week, no significantly effect on LVM or mortality and a positive impact on HTN and hyperphosphatemia [276].
Nevertheless, there are also downsides reported respective to the NHD method: detrimental factors such as fear of self-cannulation, lack of confidence in conducting an adequate dialysis session, fear of possible complications and burden on family members were found in 56 patients that completed a survey [280].
Frequent Daily Hemodialysis
Kjellstrand et al. conducted in 2008 a survival statistical analysis of 415 patients treated by SDHD in 5 centers for a period of 23 years. 150/415 patients were treated in-center and 265/415 by home or self-care HD. The results showed a 5-year cumulative survival of 68 ± 4.1 % and a 10-year survival of 42 ± 9 %. Frequency of HD (5, 6 or 7 times/week) was not associated with mortality. The survival of patients on SDHD was 2–3 times better than that of matched CHD patients [263]. Johansen et al., in the same cohort study using USRDS database patients, showed for SDHD patients only a non-significant reduction in the risk of death compared to CHD [275]. In line with Johansen et al.’s report, Suri et al., found, in a multinational cohort study, that in-center daily HD did not improve the mortality rates. The study comprised in 318 patients from the International Quotidian Dialysis Registry that received >5 times/week daily HD and that were matched to 575 CHD-treated patients [281].
The effects of SDHD versus CHD, on LVH and inflammatory status, were investigated by Ayus et al. in a non-randomized, controlled trial. The study enrolled 26 SDHD (3 h/session, 6 times/week) patients and 51 matched CHD, for a 12 months follow-up period. After adjustments for cofounding factors, SDHD was associated with 30 % decrease in LVMI, improved fluid, phosphorus and inflammatory parameters control in comparison to CHD [282]. However, discordant results were found by the Daily FHN Trial: frequent daily HD (6 times/week) resulted into a significantly improved weekly stdKt/Vurea, improved control of HTN, hyperphosphatemia and significant benefits regarding LVM and mortality, even though the patients were more prone to vascular access-complications [283].
The FREEDOM study is a cohort matched pair study that will include approximately 500 HD patients from 70 centers trying to evaluate the benefits of home daily HD (DHD) – 6 times/week. The control group will consist of a matched CHD cohort derived from the US Renal Data System database using a 10:1 ratio, totaling 5,000 patients. The primary outcome is an analysis that compares hospitalization days/patient-year between the DHD and CHD groups. Other outcomes will include: non-treatment-related medical expenditures in both groups and, in addition, in the DHD cohort, changes in quality-of-life measures (baseline, 4 and 12 months, and every 6 months thereafter), urea kinetics, parameters related to anemia, bone and mineral metabolism, and nutrition, vascular access interventions, and medication will be examined. The follow-up period is at least 1 year [284]. An interim report of this study, in 128 patients, showed that DHD is associated with long-term improvement in depressive symptoms and post-dialysis recovery time [285] and another one, performed in 154 patients, demonstrates that DHD improves physical wellbeing, assessed by Health Related Quality of Life measurements [286].
Residual Renal Function
A major concern with all of these techniques remains whether they are helpful for preservation of residual renal function (RRF). RRF is increasingly becoming an important parameter for nephrologists and one of the major goals in the management of RRT patients. Jansen et al. showed in 279 incident HD patients and 243 PD patients that predictors for RRF decline are elevated DBP and higher proteinuria, dialysis hypotension and dehydration [287]. It has been also demonstrated that PD patients present, in general, a slower decline of RRF than patients on HD, maybe due to a more ‘natural’ fluid removal and fewer hypotension episodes [287–290]. It was found, in PD patients, that for every 1 ml/min increase in RRF there is a 50 % decrease in the risk of death; also, an analysis of the CANUSA study showed that a 250 ml increase in daily diuresis in associated with a 36 % reduction in mortality, suggesting, by expressing the RRF as urinary output, that a fluid control is more important than solute clearance [291–294]. Preserving RRF is associated with better fluid control and a more liberal fluid intake, maintained endocrine function, secretion of organic acids and a better dialysis adequacy [287]. RRF loss was associated with mortality, LVH, arterial stiffness and other ESRD complications [291, 293, 295–299].
An optimal management for preservation of RRF has not been yet determined since renal function decline appears both in dehydration and overhydration. Thus, some nephrologists are in favor of maintaining patients ‘wet’, whereas others prefer the patients ‘dry’. Hypovolemia and hypotension are known factors to induce loss of RRF, especially in PD patients [295, 296, 300], but so are overhydration-associated HTN and LVH [287]. Gunal et al. showed that controlling fluid status, by salt restriction and increased UFR, led to 2.8 kg weight loss and a 28 % reduction in urine output [301]. McCafferty et al. failed to demonstrate in PD patients that a better preservation of RRF is achieved with overhydration status [293].
As mentioned before, PD patients seem to preserve RRF for a longer time than HD patients, implying therefore that loss of RRF could be also determined by HD technique-related factors. Daugirdas et al. conducted recently an analysis of FHN studies to investigate if frequent HD could result in a more rapid decline of RRF, compared to CHD. They found that in both of FHN trials, baseline RRF was inversely associated with dialysis vintage. Frequent HD participants from these trials, with a percentage of RRF at baseline, suffered a significant progressive loss of RRF at the end of follow-up period: at 12 months, in the Nocturnal FHN, 67 % of patients had zero urine output in comparison to 36 % in CHD group and in Daily FHN, the method of treatment did not had a significant impact. The authors concluded that frequent NHD appearsto promote, by undetermined mechanisms, a more rapid loss of RRF [302].
< div class='tao-gold-member'>
Only gold members can continue reading. Log In or Register a > to continue
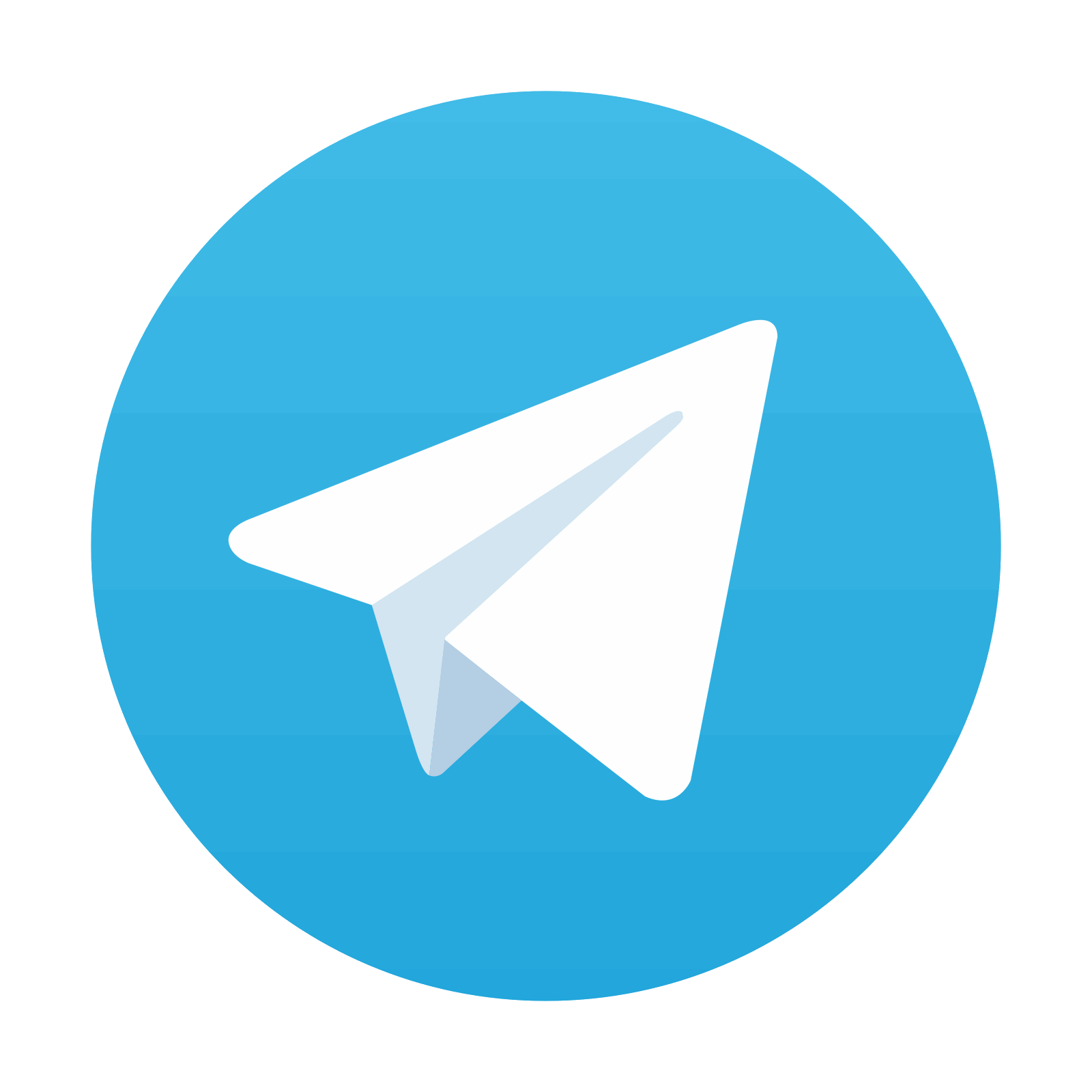
Stay updated, free articles. Join our Telegram channel

Full access? Get Clinical Tree
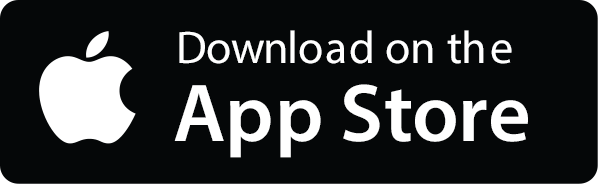
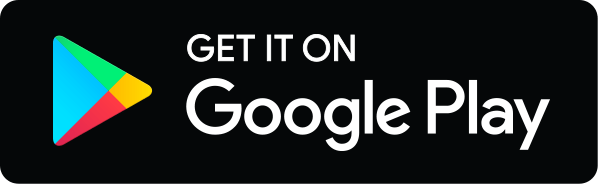