Visceral Arteries
11.2Anatomy, Variants, and Collaterals
11.4Normal Findings and Variants
11.7Comparison of Color Duplex Sonography with Other Modalities
11.8Importance of CDS and CEUS in Clinical Diagnosis
11.1 General Remarks
Diseases of the mesenteric arteries and celiac trunk are difficult to diagnose clinically due to a lack of specific symptoms. Frequently they are misidentified or diagnosed too late. The most common diseases are atherosclerosis, thrombosis, embolism, tumors, and inflammatory processes involving the visceral vessels. The diseases are classified as primary or secondary7:
•Primary diseases include giant-cell arteritis and panarteritis nodosa.
•Secondary diseases may be caused by extrinsic tumor compression, tryptic digestion in pancreatitis, or radiation-induced changes.
Symptoms depend on the degree and acuteness of hemodynamic changes. Moreover, there is a relatively large range of anatomic variance marked by varying degrees of compensation by collaterals. Slowly developing occlusions may remain asymptomatic, whereas an acute occlusion is usually a life-threatening event.
Despite significant advances in interventional therapy (thrombus aspiration, local thrombolysis, stenting of stenosis, etc.) and surgical options (bypass, intraoperative thrombolysis, intraoperative angiography, retrograde stenting), the mortality from acute intestinal ischemia continues to be high. The main reason for this is a frequent delay in diagnosis.8
▶ Angiography. Originally, contrast angiography was the only procedure available for the diagnosis of acute intestinal ischemia. Its early use could reduce mortality from over 90% to 53%. Angiography can also enable endovascular treatment of stenosis and occlusion. Another indication for angiography is in the localization and embolization of bleeding sites. Advantages of angiography are its ability to provide large-scale, nonsuperimposed views of the vascular tree, detect and evaluate caliber changes, and supply additional hemodynamic information. However, its use as a screening tool to investigate chronic abdominal pain or equivocal auscultation findings is obsolete. Today, it is most commonly used as an adjunct to computed tomography (CT).
▶ Computed tomography. Multislice computed tomography with contrast (CT) is considered the procedure of first choice in patients with no signs of peritonitis. It can detect thrombus in the mesenteric vessels (arterial and venous) and pneumatosis intestinalis or air in the portal vein as evidence of acute intestinal ischemia.8 , 9 CT is not a suitable screening tool for investigating equivocal findings because of its radiation exposure, contrast nephrotoxicity, and possible contrast allergy.
However, CT is the imaging modality of first choice in patients with an acute abdominal pain. The plain abdominal radiograph is still ranked theoretically ahead of CT for the detection of free air or bowel obstruction, but only if the film can be obtained without delay. Time delay is also of concern for ultrasound in case of suspected acute mesenteric ischemia; additionally, the image quality is often drastically impaired by bowel gas, making ultrasound not a screening tool in the acute setting.10 This is not true for chronic mesenteric ischemia or unclear mild abdominal symptoms, where ultrasound is generally used prior to CT as a screening procedure and may even establish a diagnosis, making it unnecessary to proceed with CT.11
▶ Ultrasound. Ultrasound imaging of the mesenteric vessels has been practiced since the mid-1980s.12 – 15 At that time, the main trunks of the large intestinal vessels could be visualized and evaluated with B-mode and “conventional” duplex ultrasound. These techniques were not widely used initially, however. Color duplex sonography (CDS) offered the decisive advantage of more rapid vascular imaging, making it useful in screening for diseases of the intestinal vessels and for the follow-up of interventional procedures and bypass surgery. Several studies have established criteria for the sonographic diagnosis of ≥ 50% and ≥ 70% stenosis of the superior mesenteric artery (SMA) and celiac trunk.10
Use of the ultrasound contrast agent Lumason® has further expanded the applications of contrast-enhanced ultrasound (CEUS). It has increased sensitivity in the evaluation of intraluminal thrombi, intimal flaps, and acute mesenteric artery occlusion. CEUS can also be used to evaluate end-organ perfusion (see Chapter 17: Contrast-Enhanced Ultrasound (CEUS) in Intestinal Diseases). It is noninvasive and can be used at bedside in critically ill patients without the disadvantages of CT noted above.17
The mesenteric arteries and veins comprise a pathophysiologic and functional unit. For didactic reasons, however, we will initially limit our attention to diseases of the visceral arteries and their sonographic findings. For ontogenetic reasons the mesenteric veins are discussed in the next chapter (see Chapter 12) along with the other abdominal veins and diseases of the mesosplenoportal axis.
11.2 Anatomy, Variants, and Collaterals
The abdominal organs and gastrointestinal tract derive most of their blood supply from the three unpaired visceral branches of the abdominal aorta: the celiac trunk, SMA, and inferior mesenteric artery. These vessels develop from an initially unpaired, stepladder-like vascular system through partial fusion and regression of the embryonic segmental arteries (Fig. 11.1). Disturbances in this complex developmental process lead to numerous variants of origin, position, and course, which may be misleading for the examiner.18 , 19 They are briefly discussed below.
Fig. 11.1 Embryonic development of the celiac trunk and superior mesenteric artery. 1, left gastric artery; 2, common hepatic artery; 3, splenic artery; 4, superior mesenteric artery; 5, accessory hepatic artery; 6, ectopic hepatic artery; 10—13, embryonic segmental arteries. (Reproduced with permission from Kadir S. Normal and Variant Angiographic Anatomy. Philadelphia: Saunders; 1991) (a) The embryonic segmental arteries arise in a stepladder pattern from the originally symmetrical aorta. They are initially interconnected by a longitudinal, ventral anastomosis. (b) After the regression of segmental arteries 11 and 12, the celiac trunk develops from segmental artery 9, the superior mesenteric artery from artery 13, and the inferior mesenteric artery from artery 20 or 21. The ventral anastomosis normally undergoes complete regression. (c) Absent or partial regression of the ventral anastomosis leads to an atypical hepatic blood supply from the superior mesenteric artery or via an accessory right hepatic artery or common hepatic artery. (d) Absent or partial regression of the ventral anastomosis leads to an atypical hepatic blood supply from the superior mesenteric artery or via an aberrant right or common hepatic artery. (e) The embryonic longitudinal anastomosis may persist as an anterior collateral (Bühler’s anastomosis). (f) Simultaneous regression of segmental artery 10 leads to the variant of a common arterial trunk called the celiacogastromesenteric trunk.
11.2.1 Celiac Trunk and Its Branches
The celiac trunk is a short arterial trunk that arises from the aorta at the level of the T12–L1 vertebrae, just below the aortic aperture in the diaphragm. It initially runs downward from the aorta, or occasionally may run horizontally or curve upward, and in 65% to 75% of cases it divides into three branches: the left gastric artery, common hepatic artery, and splenic artery (Fig. 11.2). In 5% to 10% of cases a fourth branch is formed by an additional pancreatic artery or an atypical origin of the dorsal pancreatic artery. In rare cases the celiac trunk may be absent or may have a common origin with the SMA, forming the celiacomesenteric trunk.18 , 20
Fig. 11.2 Angiographic (digital subtraction angiography [DSA]) views of the celiac trunk and superior mesenteric artery (see also Video 11.1). (a) Celiac trunk with the common hepatic artery (1), gastroduodenal artery (2), proper hepatic artery (3), left gastric artery (4), and splenic artery (5). (b) Superior mesenteric artery with the right colic artery (1), middle colic artery (2), ileocolic artery (3), jejunal arteries (4), and ileal arteries (5).
The left gastric artery is the first branch of the celiac trunk in 90% of the population. It runs between the layers of the lesser omentum and anastomoses with the right gastric artery on the lesser curvature side of the stomach. It also anastomoses with branches of the right gastroepiploic artery and the short gastric arteries. In 2.5% of cases the left gastric artery arises directly from the abdominal aorta.
The common hepatic artery arises from the celiac trunk and runs to the right, along the superior border of the pancreas, into the hepatoduodenal ligament. There it is typically located to the left of the common bile duct and anterior to the portal vein. After giving off the gastroduodenal artery from its inferior surface, the common hepatic artery continues to the liver as the proper hepatic artery and divides into the left and right hepatic arteries. In 45% of cases they give off an additional branch, the middle hepatic artery, which supplies the medial segment of the hepatic left lobe and the quadrate lobe.
Variants are numerous. In up to 20% of cases the liver receives some or all of its blood supply from the SMA. Local anatomy may be complicated by numerous accessory arteries arising directly from the celiac trunk, from the left gastric or splenic artery, or by branches that cross to the contralateral hepatic lobe (see Fig. 15.1). Vessels in the liver capsule, gallbladder, and small arteries in the porta hepatis can provide additional sources of collateral supply.18 , 20
The gastroduodenal artery runs behind the duodenum and anterior to the pancreas. It divides into the right gastroepiploic artery and into the anterior and posterior superior pancreaticoduodenal arteries, forming a pancreatic arcade that anastomoses with the territory of the SMA. They supply the head of the pancreas, pylorus, portions of the duodenum, as well as jejunal branches in some cases.21
11.2.2 Superior and Inferior Mesenteric Arteries
The SMA arises from the aorta 0.5 to 2 cm below the celiac trunk at the level of the L2 vertebra. It runs downward, passing behind the pancreas and posterior to the splenic vein. After descending for approximately 4 cm, it gives off the inferior pancreaticoduodenal artery and the middle and right colic arteries. The jejunum and ileum receive their blood supply from the jejunal and ileal arteries, which interconnect to form numerous arcade-like anastomoses (Fig. 11.2, Video 11.1). The ileocolic artery is the terminal branch of the SMA and supplies the proximal ascending colon, cecum, terminal ileum, and appendix.
Video 11.1 Selective mesentericography in the arterial and venous phases. View after selective catheterization of the superior mesenteric artery (SMA) initially shows the main trunk of the SMA with its divisions. The bowel opacifies in the capillary phase. In the late venous phase, blood is collected from the ileocolic artery and from the jejunal and ileal branches, and the main trunk of the SMA is densely opacified (see also Fig. 12.6b).
The inferior mesenteric artery arises from the anterior or left side of the aorta, approximately 3 cm above the iliac bifurcation at the level of the L3–L4 vertebrae. It first runs inferiorly, giving off the left colic artery after a distance of 3 to 4 cm. That vessel ascends to the left colic flexure. The descending branch supplies the sigmoid colon (sigmoid arteries) and crosses over the common iliac artery, at which point it becomes the superior rectal artery.
11.2.3 Preformed Arterial Collaterals
Numerous preformed vessels can supply collateral blood flow to the bowel in response to a slowly developing arterial occlusion (Fig. 11.3). The arc of Riolan (Riolan arcade) is a well-known anastomosis that connects the left colic artery to the first branch of the ascending limb of the SMA—generally the middle colic artery. The main collateral pathway in response to an occlusion or high-grade stenosis of the celiac trunk is usually the gastroduodenal artery with the pancreatic arcades.
Fig. 11.3 Preformed collateral pathways in the abdominal region. The most important cross-connections consist of anastomoses between the middle and left colic arteries (I, arc of Riolan), the superior and inferior pancreaticoduodenal arteries (II, pancreatic arcades), anastomoses between the middle colic artery and splenic artery (III), and connections with the internal iliac territory via the superior and inferior rectal arteries (IV). 1, abdominal aorta; 2, celiac trunk; 3, common hepatic artery; 4, gastroduodenal artery; 5, left gastric artery; 6, splenic artery; 7, superior mesenteric artery; 8, inferior pancreaticoduodenal artery; 9, middle colic artery; 10, left colic artery; 11, inferior mesenteric artery; 12, superior rectal artery; 13, common iliac artery; 14, internal iliac artery; 15, internal pudendal artery; 16, inferior rectal artery. (Reproduced with permission from Lusza.21)
Other cross-connections are formed by the Bühler’s anastomosis (see Fig. 11.1) and the marginal artery of Drummond, which runs along the mesenteric side of the colon and is supplied by branches of the middle, right, and left colic arteries.
With a complete occlusion of all the main visceral branches, collateral flow can still be supplied from above via intercostal, diaphragmatic, and esophageal arteries. Branches of the internal iliac artery and the inferior and superior hemorrhoidal arteries can deliver blood to the inferior mesenteric artery from below.22 The latter collateral pathway via the inferior mesenteric artery plays a significant role due to the frequent use of aortic stent grafts and associated occlusions and may cause reperfusion leaks in aortic stents used for endovascular aneurysm repair (see Chapter 10).
11.3 Examination Technique
11.3.1 Settings
Using the initial settings described in Chapter 2, the celiac trunk and the origin of the SMA are identified in a longitudinal scan (Fig. 11.4). Then they are imaged in two planes. The patient is scanned in the supine or lateral decubitus position using a 3- to 6-MHz transducer, or a 5- to 8-MHz transducer in children and thin patients, while moderate probe compression is applied. Besides curved-array transducers, trapezoidal or phased-array transducers have also proven useful for scanning the celiac trunk. If the B-mode examination employs tissue harmonic imaging (THI), a 1.5-MHz transducer can be used. The equipment, settings, and technique for CEUS are described in Chapter 2 and Chapter 4.
Fig. 11.4 Aorta with the celiac trunk and superior mesenteric artery. Longitudinal scan through the liver demonstrates the aorta and the origins of the celiac trunk and superior mesenteric artery. Aliasing at the origin of the celiac trunk (coded in light green) is an effect caused by the beam–vessel angle. In an angle-corrected measurement, the peak velocity was less than 100 cm/s, which is still within normal limits.
11.3.2 Patient Preparations
The patient should avoid drinking carbonated beverages on the eve of the procedure and preferably should avoid an evening meal. Next day, the fasted patient is placed in the supine position. The patients are given an antigas medication at some centers.
11.3.3 Color Duplex Sonography
Using the liver as an acoustic window, the celiac trunk and the main trunk of the SMA can be imaged in over 96% of cases when a modern scanner is used. B-mode imaging is followed by color Doppler activation and adjustment, and the celiac trunk and its branches are analyzed (Fig. 11.5). Then the main celiac trunk, common and proper hepatic arteries, splenic artery, and, if possible, the left gastric artery and gastroduodenal artery are identified in longitudinal and transverse scans (Fig. 11.6). All or part of the gastroduodenal artery can be defined in over 80% of cases as a vessel running perpendicular to the proper hepatic artery and anterior to the pancreatic head (Fig. 11.6). Next, the origin of the SMA is identified and traced as far as possible in longitudinal scans. In more than 65% of cases the artery can be traced to the level of the jejunal branches and ileocolic artery (Fig. 11.7).
Fig. 11.5 Celiac trunk and its division into the hepatic artery and splenic artery. (a) The celiac trunk and aorta are displayed in an upper abdominal transverse scan. Color Doppler shows the palm-frond-like division of the celiac trunk into the splenic artery and common hepatic artery. The flow may be coded in red or blue, depending on the beam angle relative to the flow direction. Flow is not detectable at sites with a perpendicular beam angle (note the fan-shaped beam pattern). (b) Celiac trunk in an upper abdominal transverse scan. When viewed in power Doppler mode, the celiac trunk, splenic artery, and hepatic artery are uniformly encoded in red, regardless of the flow direction. Comparison of the B-mode and color images shows that vessel diameter is overestimated in the color image because of blooming artifact. (c) Spiral computed tomography (CT) (arterial phase) demonstrates the aorta, celiac trunk, pancreas, and diaphragmatic crura. The gastroduodenal artery and portions of the curved pancreatic arcade are cut by the scan plane to the right of the pancreatic head.
Fig. 11.6 Celiac trunk and its branches. (a) Color duplex sonography (CDS) of the celiac trunk demonstrates continuous flow coded in red. The Doppler spectrum shows a low-resistance pattern with continuous forward diastolic flow. (b) Modified longitudinal scan displays the origin and proximal portion of the left gastric artery, which can be traced to the left (coded in blue) toward the upper part of the lesser curvature of the stomach. (c) Right paramedian longitudinal scan of the pancreatic head and uncinate process. After the common hepatic artery and bifurcation have been located, the transducer is rotated to a longitudinal scan of the gastroduodenal artery anterolateral to the pancreatic head. Its flow is evaluated and the flow direction is determined (red = flow towards the transducer, indicating normal downward flow from the hepatic artery). (d) Upper abdominal transverse scan of the pancreas, aorta, and superior mesenteric artery. The branches of the gastroduodenal artery—the anterior and posterior superior pancreaticoduodenal arteries—can be identified to the right of the pancreatic head (flow coded in blue).
Fig. 11.7 Superior mesenteric artery (longitudinal and transverse scans). (a) Longitudinal scan demonstrates the main trunks of the superior mesenteric artery and vein (coded in red and blue, respectively). (b) Transverse scan of the aorta and superior mesenteric artery and the origin of the second jejunal artery. (c) Transverse scan at a more distal level displays additional small branches (ileal arteries).
Evaluation of the inferior mesenteric artery begins by defining the aortic bifurcation. From there the aorta is traced cephalad in contiguous transverse scans, applying gentle transducer pressure, until the proximal trunk of the inferior mesenteric artery is visualized on the left side of the aorta approximately 3 cm above the bifurcation. The transducer is then rotated to a longitudinal orientation and the inferior mesenteric artery can usually be traced over a length of 3 to 4 cm (Fig. 11.8). For an optimum examination, the color gain and threshold should be adjusted just below the level at which color noise pixels appear. Artifacts due to peristalsis can be reduced by applying careful compression.
Fig. 11.8 Inferior mesenteric artery (transverse and longitudinal scans). (a) Transverse scan through the origin of the inferior mesenteric artery from the aorta, 2 cm above the bifurcation. (b) Further distally the vessel runs obliquely to the left of the aorta. Doppler indicates a high-resistance spectrum with low residual diastolic flow.
The velocity scale should be set to a moderate or high level to permit effective flow detection, especially in the proximal celiac trunk and SMA. By reducing the penetration depth, the operator can increase the pulse repetition frequency (PRF) so that higher flow velocities can be detected without aliasing. If color filling of the vessels is absent or fragmentary, the velocity and frequency settings should be lowered again and any wall filters should be reduced. Other technical problems can result from reflective interfaces in the near field (intestinal gas, etc.). Vascular segments with a horizontal orientation can be imaged in transverse section by angling the transducer.
If the B-mode image shows plaques and color Doppler shows flow acceleration and turbulence, or if one goal of the examination is to assess drug-induced effects or the regulatory capacity of mesenteric blood flow, Doppler spectra should be acquired at the origin of each vessel and also in any suspicious areas.
11.3.4 Examination Time
The length of the ultrasound examination depends on the clinical question. If acute intestinal ischemia is suspected, a few minutes should be allowed for ultrasound scans at the most so that necessary contrast-enhanced CT, with its therapeutic implications, will not be delayed. Ultrasound can quickly detect acute mesenteric ischemia due to an acute arterial or venous thrombotic occlusion, pneumatosis intestinalis, or air in the portal vein so that further therapeutic steps can be initiated. Besides acute thromboembolic occlusion, other possible conditions such as nonocclusive mesenteric ischemia (NOMI) can be diagnosed with CTA.9 , 23 If free intra-abdominal fluid is found and a ruptured visceral artery aneurysm is suspected, angiography with possible embolization would be indicated in a hemodynamically stable patient and open surgery in an unstable patient.
In patients with chronic intestinal disease, the search for stenoses and possible subsequent functional evaluation may take 15 minutes or more. If stenosis is found, all three intestinal vessels should be analyzed along with the flow direction in the pancreatic arcade.
11.3.5 Contrast-Enhanced Ultrasound
The combination of CDS and CEUS can significantly improve accuracy in the diagnosis of nonstenotic changes such as thrombi, dissections, intimal flaps, and mesenteric artery occlusion. End-organ perfusion can also be investigated by contrast-enhanced imaging with Lumason®: hyperperfusion is found in acute or chronic inflammation, hypoperfusion in ischemia.17 , 24 Perfusion defects will be seen in patients with a hepatic or splenic infarction.
CEUS is a noninvasive bedside procedure that can eliminate the need for further CT examination in some critically ill patients (e.g., in intensive care unit [ICU]).25
11.4 Normal Findings and Variants
11.4.1 Normal Findings
The celiac trunk has a palm-frond-like appearance when viewed in a transverse color Doppler scan (see Fig. 11.5). The common or proper hepatic artery and the splenic artery can be traced into the liver and spleen, respectively, appearing as vessels perfused by pulsatile, antegrade flow (see Fig. 15.2). The Doppler spectrum resembles that of the internal carotid artery. This similarity results from the low resistance in the distal vascular bed, which permits continuous antegrade blood flow even in diastole (Fig. 11.6, Fig. 15.4 b and c). In patients with little or no stenosis, the values shown in Table 11.1 are found in the region of the celiac trunk.20 The flow velocities measured during expiration are significantly higher than the inspiratory velocities.21
By rotating the transducer, the operator can trace the gastroduodenal artery down to the pancreas and its division into the anterior and posterior superior pancreaticoduodenal arteries. Normal flow in the gastroduodenal artery is directed inferiorly (Fig. 11.6).
The left gastric artery can be traced along the inferior surface of the hepatic left lobe in a modified longitudinal scan (Fig. 11.6). Usually only the proximal segment can be visualized, but in some cases the vessel can be traced to its possible anastomosis with the left hepatic artery. Its Doppler spectrum, like that of the gastroduodenal artery, shows greater pulsatility with very little diastolic flow.
The SMA arises from the anterior or anterolateral surface of the aorta. By varying the probe alignment as needed, the operator can trace the main trunk of the artery for up to 8 cm (Fig. 11.7). The Doppler waveform shows continuous, distally directed systolic flow with a steep upstroke and rapid downstroke. In the fasted patient, a notch occurs in the late systole, indicating a brief reverse-flow component encoded in the opposite color. Flow may cease in the late diastole (Fig. 11.9). Postprandial spectra will show an increased peak systolic velocity (PSV) and a disproportionate rise of end-diastolic velocity. This reflects the physiologic hyperemia that is induced by digestion. Analogous spectral changes are found after the administration of glucagon.
Fig. 11.9 Normal Doppler spectra from intestinal arteries and factors that affect them. (a) Normal spectrum from the superior mesenteric artery of a fasted patient displays a notch, indicating a small reverse flow component with very little residual diastolic flow due to high resistance in the distal vessels (analogous to the external carotid artery). In the variant with an aberrant hepatic artery arising from the superior mesenteric artery, higher diastolic flow can be found in spectra acquired proximal to the origin of the hepatic artery. (b) Physiologic hyperemic response in the postprandial spectrum of the superior mesenteric artery shows a marked increase in both the peak systolic frequency and the diastolic flow component due to hyperemia. (c) Normal spectrum from the inferior mesenteric artery with low residual diastolic flow. Inflammatory bowel disease or diarrhea would show a hyperemic response with a spectral change like that seen in the superior mesenteric artery.
Normal values for the SMA in patients with little or no stenosis are shown in Table 11.1. The maximum flow velocity in the SMA rises continuously after a meal, reaching a peak value (134 ± 14% of the baseline value) after a period of 41 ± 4 minutes.28
The inferior mesenteric artery can be imaged in over 92% of patients when modern equipment is used in conjunction with the examination technique described above.29 Its main trunk normally shows homogeneous color filling during systole (Fig. 11.8). Doppler spectra show a flow pattern similar to that of the SMA: high velocity in systole and little or no flow in diastole. The values listed in Table 11.1 are found in normal patients.29 In occlusive disease of the celiac and superior mesenteric arteries or the iliac artery, values in the inferior mesenteric artery are still normal (up to 189 ± 58 cm/s).30
11.4.2 Variants
Variants such as an isolated origin of the hepatic artery from the aorta (Fig. 11.10) or the common hepatic artery and right hepatic artery arising from the SMA (Fig. 15.2) are easily detected with CDS under favorable scanning conditions (see Chapter 15). The detection of these variants is helpful, for example, in planning interventions such as intrahepatic chemotherapy.
Fig. 11.10 Isolated origin of the hepatic artery from the aorta.
Bronchopulmonary Sequestration
Other variants detectable with transabdominal ultrasound are pulmonary malformations supplied by abdominal vessels. An example is bronchopulmonary sequestration, first described by Pryce in 1946.31 It is a rare congenital malformation of the lower respiratory tract characterized by a nonfunctioning mass of lung tissue that lacks normal communication with the tracheobronchial tree. It derives its arterial blood supply from the systemic circulation. The sequestration may be intralobar or extralobar, depending on its pleural investment. Sade expanded the classification of bronchopulmonary sequestration in 1974. In most cases the sequestration has a systemic arterial blood supply while venous drainage is via the pulmonary veins in the intralobar form and via systemic veins in the extralobar form. Both forms may exhibit any pattern of anomalous vascular supply and drainage. The parenchyma or bronchi in the aberrant lung segment may be unchanged, and there may be communication with the gastrointestinal tract.32 The combination of an atypical vessel arising from the abdominal aorta or its main branches and passing up to the diaphragm or a history of recurring bronchopulmonary infections should suggest the possibility of bronchopulmonary sequestration (Fig. 11.11).
Fig. 11.11 Bronchopulmonary sequestration receiving its arterial supply from the celiac trunk. (a) Longitudinal scan of the aorta and celiac trunk. A large-caliber artery arises from the celiac trunk and ascends with the aorta through the aortic aperture in the diaphragm. (b) Scans at a higher level trace the vessel toward the diaphragm. (c) Magnetic resonance angiography (axial cine sequences and three-dimensional angiographic sequences after intravenous administration of Gd-BOPTA). The aberrant artery runs from the celiac trunk to posterobasal lung segment 10 of the right lower lobe. The sequestration drains to the left atrium via a large pulmonary vein of the right lower lobe.
Intestinal blood flow is a complex process that is controlled and influenced by mechanical, metabolic, and neural factors as well as numerous vasoactive substances.33 Intestinal blood flow is dependent on dietary composition. It decreases in response to stress and physical exercise. The arterial blood supply to the bowel may become compromised as a result of stenosis, thrombosis, embolism, tumor compression, volvulus, adhesions, mesenteric vein thrombosis, or severe circulatory insufficiency (nonocclusive disease). Slowly developing processes may be compensated by collateral circulation. Clinically, patients may be asymptomatic or may present with acute or chronic intestinal ischemia. Acute occlusions, deficient collateral circulation, or involvement of two or more vessels can lead to intestinal damage.
Many of these disorders were formerly misinterpreted as inflammatory bowel disease. With the increasing use of endoscopy, endosonography, histology, refined angiographic techniques, multislice computed tomography angiography (CTA), and CEUS, we have been able to differentiate other entities such as ischemic colitis and drug-induced colitis.9 , 17 , 23 , 24
11.5.1 Plaque, Stenosis, and Occlusion
The principal cause of stenosis is atherosclerosis. Narrowing may also result from fibromuscular dysplasia (FMD). At the abdominal level this rare disease may affect, in descending order of frequency, the renal and splenic arteries, the SMA, and the celiac trunk. Other pathologies are extrinsic compression by benign and malignant tumors, the arcuate ligament syndrome, and stenotic changes due to vasculitic diseases.
Atherosclerosis
Atherosclerotic changes in the visceral arteries are found as early as the third decade of life. With further aging, plaques may form that cause luminal narrowing or obstruction. The lesions are classified by their location as involving the origin of the artery, its main trunk, first- and second-order branches, or diffuse involvement of the peripheral branches.
Atherosclerotic stenosis in the proximal portion of the visceral arteries is generally short, circumferential, or eccentric (ostial stenosis in aortic atherosclerosis). Often the only early findings are isolated wall plaques, which at most cause slight flow irregularities. As the degree of stenosis increases, turbulence and flow acceleration occur. Diabetic patients most often present a diffuse pattern of atherosclerosis with the concomitant involvement of peripheral branches. They appear irregular on angiography with no associated collateral circulation.
▶ Findings. Calcified plaques have a sonodense appearance on ultrasound and cast a typical acoustic shadow in accordance with their size and insonation angle. Depending on the degree of narrowing, CDS shows circumscribed flow acceleration that may cause aliasing, turbulence, and possible poststenotic dilatation. In high-grade stenosis, peripheral vascular segments may show waveform damping and/or retrograde flow in the gastroduodenal artery (Fig. 11.12). High-grade stenosis may also produce coarse vibration artifacts that transcend the vessel lumen (Fig. 11.13). This “confetti sign” was described previously for peripheral vessels and the aorta (Video 3.2).
Fig. 11.12 High-grade stenosis of the celiac trunk with an associated steal phenomenon. (a) Longitudinal scan through the aorta, celiac trunk, and superior mesenteric artery. The celiac trunk is narrowed by the crus of the diaphragm. Flow in the stenosis is accelerated to more than 300 cm/s, marked by aliasing and turbulence (coded in light green). (b) Longitudinal scan along the gastroduodenal artery. Flow reversal (arrow) contrasts with normal antegrade flow (see Fig. 11.6).
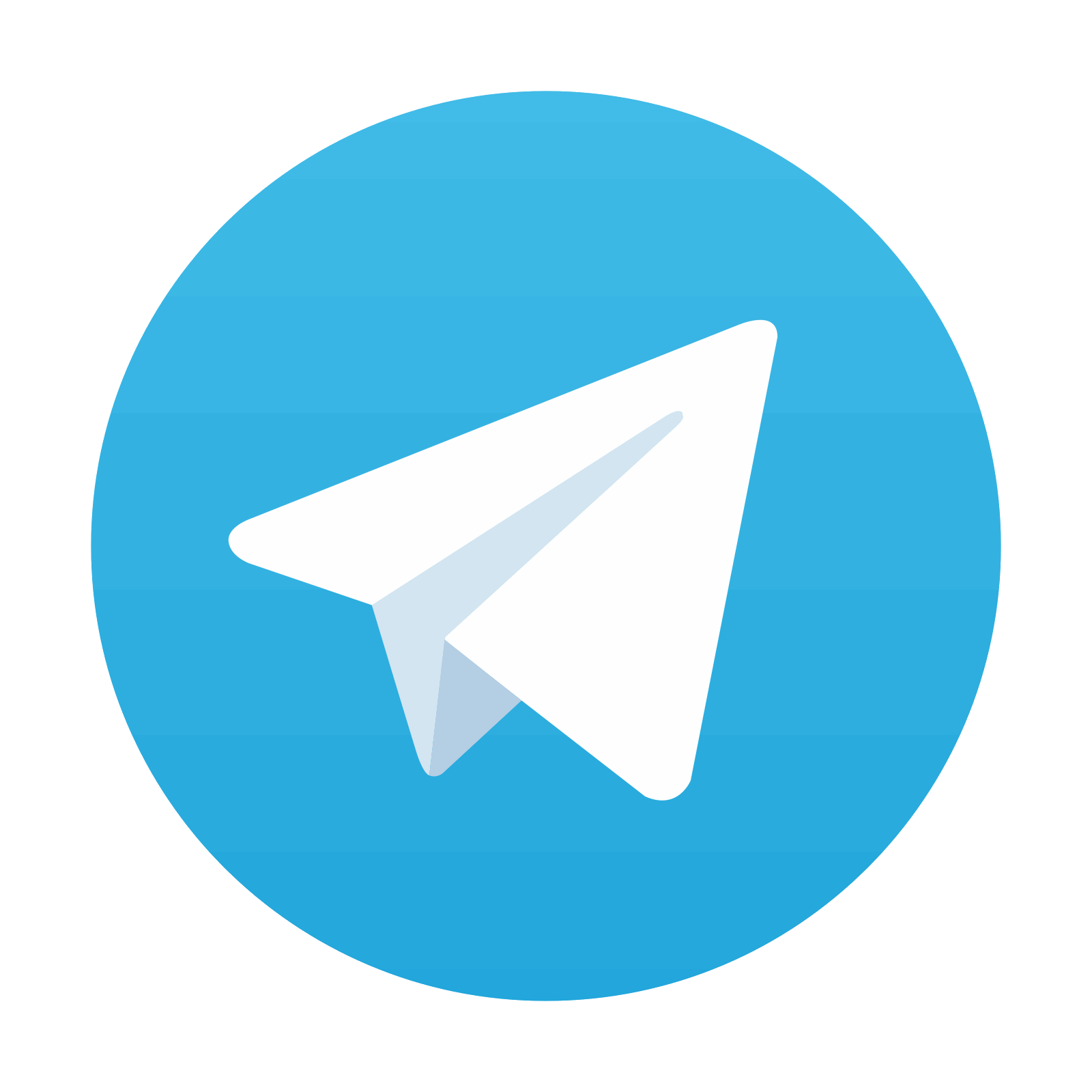
Stay updated, free articles. Join our Telegram channel

Full access? Get Clinical Tree
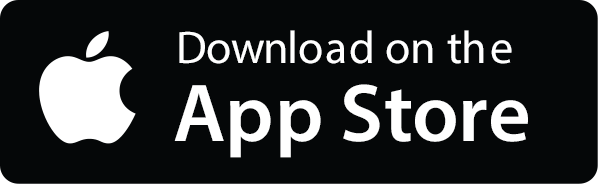
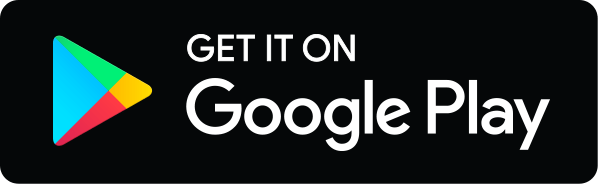