Abstract
Ventricular septal defects (VSDs) remain one of the commonest indications for surgery among infants and children with congenital heart disease. With advancements in echocardiography, there remains a very limited need for cardiac catheterization in the management of these patients. Although perioperative mortality and morbidity remain low for isolated defects, special scenarios like the surgical management of multiple VSDs and the decision making in patients with pulmonary hypertension remain a challenge. This chapter reviews both the classic and current evidence shaping the management of this condition with a brief mention of the data behind emerging interventional procedures used both in the catheterization laboratory and the operating room.
Key Words
Ventricular septal defect, perimembranous, pulmonary hypertension, surgical repair, periventricular
Definition
A ventricular septal defect (VSD) is an opening in the interventricular septum resulting in direct communication between the left and right ventricles. VSDs can be single or multiple. It is the most commonly diagnosed congenital heart anomaly, present in 20% of patients with congenital heart disease.
Embryology
Traditionally the ventricular septum is thought to be derived mostly from three sources. The primary fold arising from the apex of the primitive ventricle, eventually becoming the trabecular septum, fuses with the inlet septum, which originates posteroinferiorly, and with the infundibular or conal septum, which extends downward from the conal ridge. Some evidence, however, points to the inlet and the apical trabecular septa originating from the same source. The conal ridge fuses with the endocardial cushions to form the membranous portion of the interventricular septum. Fig. 49.1 shows the various components contributing to the formation of the interventricular septum.

Anatomy
There are several schemes for classifying VSDs. The traditional classification describes four types based on the location of the VSD: type 1 or supracristal/subarterial/conal, type 2 or perimembranous/paramembranous, type 3 or inlet/atrioventricular canal, and type 4 or muscular ( Fig. 49.2 ). A separate classification of “malaligned” defects is often employed to describe VSDs that are part of lesions like tetralogy of Fallot or interrupted aortic arch, in which the conal septum and the other components of the septum are fully formed but are displaced anterior or posterior with respect to each other. Perimembranous defects constitute 80% of the defects with the other types being 5% to 10% each.

Subarterial defects are also known as outlet, conal septal, supracristal, or subpulmonary VSDs. They are located beneath the pulmonary valve, and their superior edge is a fibrous ridge between the two semilunar valves. They can be associated with prolapse of the right coronary leaflet of the aortic valve with associated regurgitation. This type of defect is more common in the Asian population.
Perimembranous (paramembranous) defects are also known as membranous or infracristal defects. They are located between the anterior and posterior divisions of the septal band and between the conal and trabecular interventricular septum. The lateral border is formed by the tricuspid annulus; the superior border is usually the aortic annulus. There may be a variable amount of muscular rim at the superior and lateral borders. The defect can extend into the inlet, trabecular, or outlet portions of the interventricular septum. Extension of the defect to the base of the noncoronary leaflet of the aortic valve may cause aortic regurgitation (AR). The conal septum may be anteriorly malaligned as in tetralogy of Fallot, causing right ventricular outflow tract obstruction, or, less commonly, it may be malaligned posteriorly, causing left ventricular outflow tract obstruction.
Inlet defects are also called atrioventricular canal-type defects. The posterior margin of the defect runs along the septal leaflet of the tricuspid valve. The anterior leaflet of the mitral valve often has a cleft. The defect extends superiorly to the membranous septum.
Muscular defect s are located anywhere in the muscular septum. The margins are characteristically muscular. They are frequently multiple. They may be anterior, midmuscular, apical, or in the inlet septum. The latter differs from the inlet or atrioventricular canal-type VSD in that it is separated from the tricuspid valve and membranous septum by muscle tissue. Infundibular or outlet muscular VSDs differ from subarterial VSDs because of the presence of a rim of muscle separating the defect from the annuli of the aortic and pulmonary valves.
Associated Anomalies
VSDs can be isolated lesions or part of a variety of major congenital malformations such as tetralogy of Fallot, double-outlet right ventricle, transposition of the great vessels, and truncus arteriosus. Fifty percent of patients with VSDs requiring repair have associated cardiovascular anomalies, most commonly patent ductus arteriosus, atrial septal defect (ASD), aortic coarctation, aortic stenosis, and pulmonary stenosis.
Pathophysiology and Natural History
The dimension of the defect and pulmonary vascular resistance (PVR) determine the blood flow across a VSD (shunt). Smaller VSDs are said to be restrictive because there is a pressure gradient across the defect with a greater left ventricular pressure than right ventricular pressure. The left-to-right shunt depends primarily on the pressure differences. Larger defects approximate the size of the aortic annulus and are nonrestrictive with equal right and left ventricular pressures. In these cases the relative resistances in the systemic and pulmonary vasculature determine the left-to-right shunting.
PVR is high in the immediate postnatal period and begins to decrease within the first 2 weeks of life. For babies with large nonrestrictive VSDs the drop in PVR accentuates the left-to-right shunt, leading to a large volume load in the pulmonary circulation, increasing left atrial pressure, and causing left ventricular volume overload. Congestive heart failure (CHF) develops with decreased peripheral perfusion and increased work of breathing, associated with a drop in stroke volume and tissue oxygen delivery. This results in activation of the renin-angiotensin system, which further increases systemic vasoconstriction and the left-to-right shunting, exacerbating the CHF.
If infants with nonrestrictive VSDs remain untreated, the pulmonary overcirculation can eventually lead to a fixed elevation of PVR and pulmonary vascular disease. These changes are not reversible after VSD closure. Eisenmenger complex develops when PVR becomes greater than systemic resistance, reversing the shunt and causing cyanosis.
Infants and children with smaller restrictive VSDs remain asymptomatic. Those with larger nonrestrictive VSDs develop increasingly severe CHF within the first several months of life. This manifests as respiratory symptoms and failure to thrive. These infants have an appreciable mortality within the first year if left untreated. Children with moderate shunting develop a gradual increase in PVR over time, and as the PVR approaches systemic levels, the degree of shunting decreases with improvement in symptoms. If untreated, such patients go on to develop Eisenmenger complex and die in the third or fourth decade of life. The development of Eisenmenger physiology increases the risk of death 10- to 12-fold and carries a 25-year survival of only 42%.
Spontaneous closure of VSDs is well documented and is more likely to occur within the first several months of life in patients with smaller defects. Muscular defects are the most likely to close by further septal muscular development. This is based mostly on autopsy studies in which most of the postmortem reports of spontaneously closed defects are of the muscular type. Perimembranous VSDs have the next highest rate of spontaneous closure. The mechanism of spontaneous closure of these defects frequently involves the adherence of excess (aneurysmal) tricuspid valve tissue. Before the description of this mechanism in 1970, septal aneurysms were often presumed to be congenital in origin. A recent natural history study from the Belgian registry on adult congenital heart disease documented a 13% incidence of spontaneous closure of perimembranous VSDs. The authors also noted a 3% incidence of infective endocarditis, 3% incidence of progression to moderate to severe aortic valve insufficiency, and a low rate of atrial arrhythmia and heart block in the unrepaired perimembranous VSD group. Of note, the unrepaired patients had a significantly smaller VSD and left-to-right shunt compared with the repaired group.
Diagnosis
Symptoms
Infants with small defects are usually asymptomatic, and the detection of a murmur results in their diagnosis. Moderate defects result in a predisposition to pulmonary infection and varying degrees of growth retardation during the first few years of life but without severe signs of CHF. Larger defects, particularly nonrestrictive VSDs, produce signs and symptoms of CHF early in the first year of life, including tachypnea, poor feeding, sweating, irritability, failure to thrive, and poor weight gain. This symptom complex results from the increased work of breathing and energy expenditure associated with pulmonary overcirculation and poor peripheral perfusion.
Physical Signs
Children with smaller defects may present with a harsh holosystolic murmur with no overt signs of CHF. Those patients with larger defects and shunts (pulmonary-to-systemic blood flow ratio [Q p :Q s ]; see subsequent discussion) in excess of 2 : 1 may have signs of CHF, including poor growth, tachypnea, poor perfusion with reduced peripheral pulses, a palpable thrill along the left sternal border, and a harsh holosystolic murmur loudest over the fourth intercostal space. There may be a diastolic murmur related to increased blood return to the left atrium. There is accentuation of the second heart sound. Hepatomegaly and pulmonary congestion may be present. Resting oxygen saturation less than 90% before surgical repair is a sign of significantly elevated PVR and portends an increased risk of pulmonary hypertension and death after surgical closure.
Electrocardiography
Typical findings on the electrocardiogram include left ventricular hypertrophy and left atrial enlargement reflected in bifid P waves and prominent R and T waves in the inferior leads and V 6 , particularly with larger defects. Findings of right ventricular hypertrophy with an RSR′ pattern in lead V 1 occur later in the disease process.
Chest X-Ray Examination
Radiographic findings include cardiomegaly and an enlarged main pulmonary artery shadow. Left atrial enlargement and prominence of the pulmonary vasculature are also seen. These radiographic findings are more notable with larger defects and may be absent or more subtle with small VSDs.
Echocardiography
The type, size, number, and location of medium and large VSDs can be accurately defined by two-dimensional transthoracic echocardiography. Figs. 49.3 to 49.6 demonstrate the four common VSD types based on their location. Left atrial and ventricular dimensions can be measured and may be important in determining the management of a particular defect. Estimates of pulmonary artery pressure can be made using Doppler imaging of the velocity of a tricuspid regurgitant jet, if present. Other associated cardiac anomalies and the relationship of the VSD to the surrounding structures and valves can also be delineated with this modality. In the majority of cases echocardiography provides sufficient information to proceed with closure of the defect or to follow it expectantly. Transesophageal or epicardial echocardiography is particularly important for providing an intraoperative assessment of VSD closure. Small defects can be missed, especially if they are in the apex of the heart or in the vicinity of larger ones, and often reveal themselves after closure of the bigger VSD.




Cardiac Catheterization
Cardiac catheterization may be indicated when echocardiographic data are unsatisfactory, in patients with large defects and significant pulmonary hypertension, or if there is doubt about the anatomy of associated lesions. Recent American Heart Association and American Thoracic Society (AHA/ATS) guidelines for pediatric pulmonary hypertension recommend cardiac catheterization to measure PVR index (PVRI) if early repair has not been performed in the first 1 to 2 years of life for a significant VSD.
Cardiac catheterization can demonstrate the location, size, and number of defects, along with associated cardiac anomalies. It facilitates direct measurement of left and right heart pressures and oxygen saturations ( Fig. 49.7 ), from which quantification of the intracardiac shunt and PVR may be derived.

The shunt fraction is the ratio of pulmonary blood flow (Q p ) to systemic blood flow (Q s ). It is calculated according to the following formula:
Q p : Q s = [ ( AO 2 − MVO 2 ) / ( PVO 2 − PaO 2 ) ]
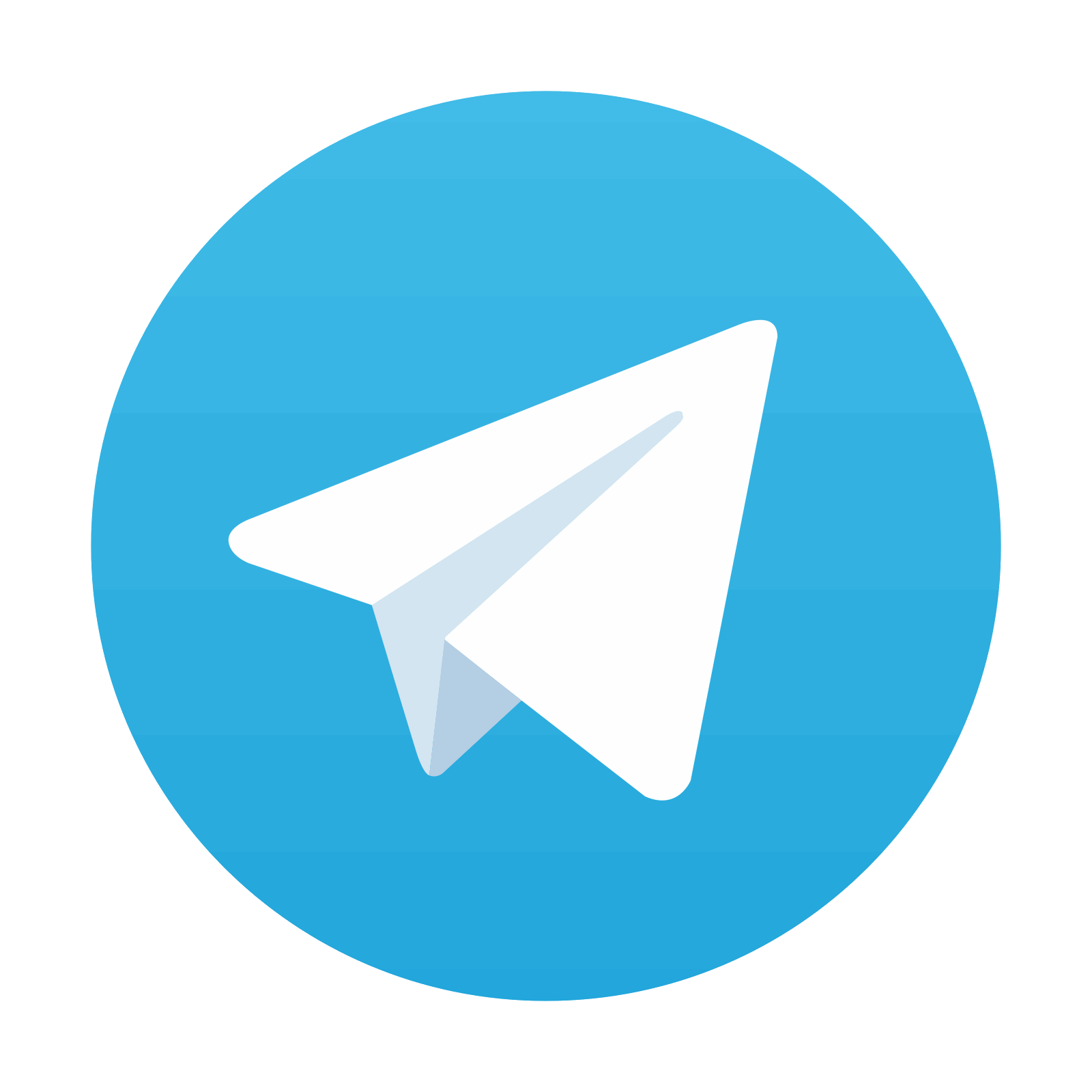
Stay updated, free articles. Join our Telegram channel

Full access? Get Clinical Tree
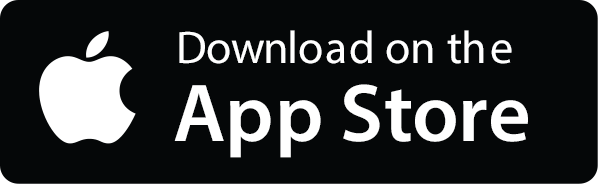
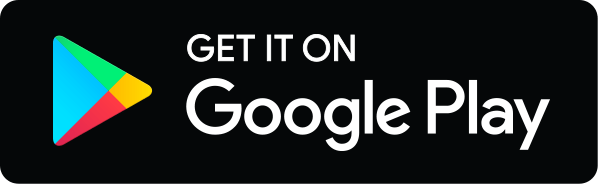