Introduction
Holes between the ventricles can occur as isolated anomalies but are also seen in association with many other defects. For example, they are found as integral parts of entities such as the tetralogy of Fallot, double-outlet ventricles, and most cases of common arterial trunk. They are also frequently encountered in association with transposition and congenitally corrected transposition. Although fundamentally different from ventricular septal defects, atrioventricular septal defects also commonly have a ventricular component (see Chapter 31 ). Ventricular septal defects, furthermore, are an integral part of the functionally univentricular circulation. Taken together, they unequivocally make up the commonest congenital cardiac malformation. This chapter considers only the “isolated” examples, although complications produced by other minor associations are discussed. Although holes between the ventricles have a long pedigree, it is with the name of Henri-Louis Roger that the anomaly is historically linked. Roger recognized that an isolated ventricular septal defect produced a typical murmur and that it could be consistent with prolonged life and good health.
Prevalence
Excluding aortic valves having two leaflets as well as prolapse of the mitral valve, which itself may be overdiagnosed, isolated ventricular septal defect is the most common congenital cardiac malformation. It is difficult to obtain an accurate assessment of prevalence. With the advent of cross-sectional echocardiography and color Doppler, nonetheless, a more accurate approximation is possible. The known high rate of spontaneous closure means that postmortem data certainly underestimated the incidence of the defect. In series depending heavily on clinical observation, the estimated prevalence has varied markedly. It has been shown to be higher in fetuses dying prenatally, and there is some variation depending on the time of fetal death. Hoffman summarized the literature addressing the proportional distribution of congenital cardiac defects from 22 series, showing that the median distribution for ventricular septal defect was 31%. The most accurate clinical data come from the prospective Bohemia Survival Study, which revealed a prevalence of 2.56 per 1000 live births, accounting for two-fifths of all cardiac malformations, very similar to the data from Merseyside in the United Kingdom, where the figure was 2.74 per 1000 births. A study from Malta, in contrast, revealed a figure of 3.94, a significant difference. This reflects the increased use of echocardiography in diagnosis. The trend is confirmed by data from the Baltimore-Washington Infant Study, which also used echocardiographic techniques and showed a remarkable increase in the diagnosis of muscular defects, with a 10-fold increase in prevalence. Ventricular septal defects accounted for one-third of the lesions identified in the infants of this study and made up over two-fifths of the malformations encountered in Malta. A particularly high prevalence of muscular defects has also been reported by those who scanned populations of neonates using color-flow imaging, noting subsequent spontaneous closure of many of the defects. None of these studies, however, was population based. Ventricular septal defects are more common in premature infants and in those born with low weight. In contrast, the incidence is not related significantly to race, sex, maternal age, birth order, or socioeconomic status.
Although there does not appear to be a genetic bias toward the incidence of ventricular septal defects, genetics do influence the specific type of defect. The doubly committed juxtaarterial defect is more common in Asian populations, in which muscular and multiple defects are less common. The frequency of the doubly committed defect requiring repair accounted for at least 30% in an Asian population, compared with the incidence of about 5% of occidental patients requiring surgery in Western societies. Muscular defects account for about three-tenths of defects requiring closure in the Western world, with multiple muscular defects accounting for approximately one-tenth of operative closure in this population. Such defects are uncommon in the Asian population referred for surgical closure, and multiple ventricular septal defects are very rare.
Morphology and Morphogenesis
Categorization of Defects
Surprisingly, there is still no consensus concerning the best way to categorize and describe channels between the ventricles. One explanation for the ongoing disagreement is that the lesion is not always as simple as it first appears. For example, when the channel between the ventricles is overridden by an arterial valve, which area represents the defect is open to debate ( Fig. 32.1 ). This situation is magnified in the setting of double-outlet right ventricle, where the channel between the ventricles functions as the outlet from the left ventricle. When patients with double-outlet right ventricle undergo surgical correction, therefore, the channel is tunneled to one or other of the ventricular outflow tracts. The area usually closed by the surgeon is the anatomic equivalent of the “ventricular septal defect” as described in the setting of tetralogy of Fallot ( Fig. 32.2 ). Most current textbooks, nonetheless, continue to describe the channel providing the left ventricular outlet in the setting of double-outlet right ventricle as the “ventricular septal defect.” There is therefore no current agreement on what area is best described as a “ventricular septal defect.”


This chapter is concerned with the situation in patients with concordant atrioventricular and ventriculoarterial connections. Our approach to definition and classification is based on several anatomic principles ( Box 32.1 ). We first define the margins of the area chosen to represent the defect. Having defined this area, our second principle is to account for all its various anatomic features. We take the stance that the most important features are its boundaries, since these features determine the phenotypic categorization. We recognize the necessity also of describing the position of the hole relative to the landmarks and components of the ventricular septum, including the surgically significant atrioventricular conduction axis. If present, we take account of any malalignment between the different septal components. Attention must also be given to the size of the channel chosen to represent the defect. These principles also underscore the categorization that will appear in the 11th iteration of the International Classification of Congenital Heart Disease established by the World Health Organization, known as ICD-11. In this categorization, nonetheless, the geographic location of the channels is used as the primary feature, with the phenotypic variants described as secondary features.
Define the area of the defect.
Define the boundaries of the defect: perimembranous, muscular, or doubly committed and juxtaarterial.
- ■
The relation of the defect to the atrioventricular conduction axis
- ■
The relation of the defect to the atrioventricular valves
- ■
The relation of the defect to the arterial valves
- ■
Define the geographic location of the defect relative to the right ventricular aspect of the ventricular septum: opening to the inlet, apical trabecular, or outlet parts of the right ventricle.
Define the presence of septal malalignment: subpulmonary outlet septum, or atrial and muscular ventricular septal malalignment.
Define the size of the defect.
What Is the Defect?
When there is a simple hole, punched as it were into the substance of the muscular ventricular septum or representing divorce of the muscular septum from the freestanding subpulmonary infundibulum, there is no problem in defining its margins or in agreeing that these margins are exclusively muscular ( Fig. 32.3 , left ). The majority of channels, however, have the crest of the muscular ventricular septum as one of the margins, but overridden to some extent by the leaflets of the arterial or atrioventricular valves or these valves in combination (see Fig. 32.3 , right ). In the latter situation, the areas forming the right ventricular border are of greatest practical importance, since it is around these margins that the surgeon will usually place a patch to restore septal integrity. We therefore define this area as the “ventricular septal defect.” The differences in the anatomic make-up of the margins of this area, as viewed from the morphologically right ventricle, provide the means of differentiating between the phenotypes.

Features Requiring Description
Appreciation of the borders of the channels between the ventricles as viewed from the right ventricle provides the means of distinguishing between the phenotypes. In the categorization to be used in ICD-11, the way in which the defects open to the right ventricle—in other words, their geography—is taken as the initial descriptive feature. On this basis, defects are described as being centrally located at the cardiac base, as opening either to the inlet or outlet of the right ventricle, or as found within the substance of the apical muscular septum. The defects are distinguished according to the nature of their borders. In this last respect, all defects can be described as being muscular, perimembranous, or doubly committed and juxtaarterial ( Box 32.2 ). A fourth group had been discussed, namely the allegedly juxtatricuspid and nonperimembranous, or inlet, defect. Patients suggested to have such defects, subsequent to echocardiographic interrogation ( Fig. 32.4 ), have subsequently been reclassified, either following direct surgical inspection or retrospective echocardiographic assessment, as having perimembranous defects with inlet extension or muscular inlet defects. We have never come across a specimen with the alleged fourth pattern in an archive of autopsied specimens.
Defects abutting on area of continuity between tricuspid and aortic valves (perimembranous)
- ■
Opening into inlet of right ventricle
- ■
Opening into outlet of right ventricle
- ■
Confluent defects
- ■
Defects encased within musculature of ventricular septum (muscular)
- ■
Opening to the inlet of the right ventricle
- ■
Opening between the apical components
- ■
Opening anterior to the septomarginal trabeculation
- ■
Opening to the subpulmonary outlet
- ■
Multiple
- ■
Defects roofed by arterial valves in fibrous continuity (doubly committed and juxtaarterial)
- ■
With muscular posteroinferior rim
- ■
Extending to become perimembranous
- ■

Muscular Defects
The holes within the substance of the septum, and some of those with divorce of the subpulmonary infundibulum from the apical muscular septum, have exclusively muscular rims. These channels are therefore described simply as being muscular (see Fig. 32.3 , left ). They can be associated, as will be shown, with malalignment of the septal components.
Perimembranous Defects
The perimembranous defects that open into the base of the right ventricle are positioned directly beneath its inner curvature. When the ventricular septum is intact, this area is occupied by the membranous part of the septum, with the supraventricular crest separating the area from the leaflets of the pulmonary valve. The membranous septum is crossed by the hinge of the septal leaflet of the tricuspid valve, which divides it into atrioventricular and interventricular components. The axis of atrioventricular conduction tissue penetrates through this septum from the apex of the triangle of Koch. Having penetrated, in the setting of septal integrity, the conduction bundle runs on the crest of the muscular septum, sandwiched between the fibrous and muscular septal components and emerging in the right ventricle beneath the medial papillary muscle, which takes its origin from the posterocaudal limb of the septomarginal trabeculation ( Fig. 32.5 ). Perimembranous defects are found when the ventricular septum is deficient in this area of normal continuity between its membranous and muscular components. Such defects, of necessity, will open centrally into the right ventricle. Indeed, central defects found at the ventricular base can only be perimembranous. Until very recently, we had presumed that their phenotypic feature was fibrous continuity between the leaflets of the aortic and tricuspid valves (see Fig. 32.3 , right ). In most instances, this is the case. We are now aware, however, that in a small proportion of cases fulfilling the criteria for the defect to be perimembranous, the fibrous continuity is found only between the leaflets of the mitral and tricuspid valves, with myocardium of the supraventricular crest interposing between the leaflets of the aortic and tricuspid valves. The strict definition for the perimembranous defect, therefore, should be the presence of fibrous tissue in the posteroinferior border of the defect producing continuity between the leaflets of the mitral and tricuspid valves, but usually also producing aortic-to-tricuspid fibrous continuity. In some instances, the tricuspid-to-mitral fibrous continuity will be through the substance of the central fibrous body.

Perimembranous defects were traditionally considered to be “membranous,” since they represent failure to close the embryonic interventricular communication by forming the membranous septum. The defects, however, are always larger than the normal dimensions of the membranous septum. The embryonic interventricular communication almost certainly fails to close because the muscular ventricular septum is deficient around its borders. That is why we prefer to describe the defects as being perimembranous.
Doubly Committed and Juxtaarterial Defects
The feature of the third phenotypic type of defect is that they occupy the region that, in the normal heart, is formed by the freestanding component of the muscular subpulmonary infundibulum. The phenotypic feature of this third type, which is both doubly committed and juxtaarterial, is fibrous continuity in the roof between the leaflets of the aortic and pulmonary valves ( Fig. 32.6 ). Such defects cannot exist in the normal heart, in which the freestanding muscular subpulmonary infundibulum has developed in normal fashion.

Position of Defects
Within the categorization of ICD-11, it is the position of the defects relative to the landmarks and components of the right ventricle or, in other words their geography, that serves as the initial feature for description. Muscular defects, which are punched within the substance of the muscular septum, can exist so as to open to the inlet, or anywhere in the apical parts of the right ventricle, including those that open anteriorly relative to the body of the septomarginal trabeculation. Muscular defects opening to the right ventricular outlet can also exist because of divorce of the apical septum from the subpulmonary infundibulum ( Fig. 32.7 ). The muscular defects can be multiple, particularly when they are in the apical part of the muscular septum, or they can coexist with perimembranous or juxtaarterial defects.

For the perimembranous defects, the situation is more complicated. Of necessity, all perimembranous defects, since they represent failure to close the embryonic interventricular septum, must open centrally within the base of the right ventricle ( Fig. 32.8B ). Depending on the extent of deficiency of the environs of the surrounding muscular ventricular septum, however, the defects can extend so as to open primarily to the right ventricular inlet ( Fig. 32.8A ), or to the right ventricular outlet (see Fig. 32.8C ). They can also be sufficiently large to open to both inlet and outlet and hence be confluent. We had previously described perimembranous defects as “excavating” into the inlet, outlet, or apical trabecular components of the septum. We now know this to be incorrect. Although much of the muscular septum, when viewed from the right side, seems to be positioned to separate the inlet of the right from the inlet of the left ventricle, this is not the case. Because of the deeply wedged location of the normal subaortic outflow tract, the majority of the septum as viewed from the right side, which is beneath the septal leaflet of the tricuspid valve, separates the inlet of the right from the outlet of the left ventricle. The seemingly septal surface of the right ventricular outflow tract, furthermore, is formed by the freestanding muscular subpulmonary infundibulum. The posterior walls of this component separate the cavity of the right ventricle from the aortic root, rather than forming a septum between the cavities of the right and left ventricles. It is not possible, therefore, in the normal heart, to recognize the boundaries of a discrete muscular outlet septum (see Chapter 2 ). Perimembranous defects that open to the outlet of the right ventricle do so because the supraventricular crest is divorced from between the limbs of the septomarginal trabeculation. In this situation, it does become possible to recognize a discrete muscular outlet septum, which forms the superior margin of the hole between the ventricles (see Fig. 32.8C ). In the central perimembranous defect, in contrast, and similarly with the perimembranous defect that opens to the inlet of the right ventricle, the outlet septum maintains its attachment between the limbs of the septomarginal trabeculation. The right ventricular edge of the supraventricular crest forms the cranial margin of the defects (see Fig. 32.8A–B ). When perimembranous defects permit shunting primarily to the inlet of the right ventricle, in addition to the fibrous continuity found between the aortic and tricuspid valves, there is an additional extensive area of continuity in the roof of the defect between the leaflets of the tricuspid and mitral valves. This results in loss of the normal offsetting between the valves. The septal leaflet of the tricuspid valve in hearts with such defects is frequently divided or deficient. If the two components of the abnormal leaflet are at all bound down to the margins, the shunting can occur from left ventricle to right atrium. Such shunting is frequently held to be caused by absence of the atrioventricular membranous septum. This can occur, but it is very unusual. If found, it produces an atrioventricular septal defect; but in the setting of separate right and left atrioventricular junctions ( Fig. 32.9A ). More usually, when there is ventriculoatrial shunting, this is first across a perimembranous inlet defect and only subsequently to the right atrium ( Fig. 32.9B ). The defects that permit ventriculoatrial shunting are described as Gerbode defects. Perimembranous defects can also, on occasion, be associated with failure of formation of the freestanding muscular subpulmonary infundibulum. In this instance, the defect will be bordered not only by fibrous continuity between the leaflets of the aortic and tricuspid valve but also by fibrous continuity in the roof between the leaflets of the arterial valves. Such defects therefore will be both perimembranous and doubly committed and juxtaarterial. Of necessity they will open to the outlet of the right ventricle ( Fig. 32.10 ).



Valvar Override and Septal Malalignment
The third important feature of any channel between the ventricles, and one that always requires description if present, is valvar override and its associated feature of malalignment of the septal components. Malalignment usually involves the outlet septum, as described for the perimembranous defect opening to the outlet of the right ventricle (see Fig. 32.8C ). In the situation illustrated in Fig. 32.8C , the outlet septum is a right ventricular structure, as is also the case in tetralogy of Fallot (see Chapter 36 ). The outlet septum can also be malaligned in posterior and caudal direction and will become a left ventricular structure, thus obstructing the outflow tract of the left ventricle ( Fig. 32.11 ). Such caudal deviation can also be found when the borders of the defect, as viewed from the right ventricle, are exclusively muscular. The outlet septum can similarly be deviated in either cephalad or caudal direction when it is fibrous rather than muscular. In the latter settings, the defect will of necessity be doubly committed and juxtaarterial. It can again have a muscular posteroinferior rim, as is usually the case for doubly committed defects (see Fig. 32.6 ), or it may extend to become perimembranous (see Fig. 32.10 ).

More rarely there can be malalignment between the muscular ventricular septum and the atrial septum. This is the phenotypic feature of hearts with override of the orifice of the tricuspid valve and straddling of its tendinous cords ( Fig. 32.12 ). The defects of necessity open to the inlet of the right ventricle. Some have described these defects as being of “atrioventricular canal” type, since the ventricular septum extends along the full width of the overriding tricuspid valvar orifice. In the allegedly “traditional” approach, however, it was suggested that the perimembranous inlet defect was of the “AV canal” type; hence it was called the “type III” defect. Both of these variants were said to be of the “atrioventricular canal” type; however, they should be distinguished from atrioventricular septal defects with common atrioventricular junction, since they have separate atrioventricular junctions, with a mitral valve guarding the left-sided junction. It is the malalignment between the atrial and ventricular septal structures that is the distinguishing feature of the lesion associated with a straddling tricuspid valve, over and above the fibrous continuity between the leaflets of the aortic and tricuspid valves (see Fig. 32.12 , left ), since the septal malalignment underscores the anomalous location of the atrioventricular conduction axis (see Fig. 32.12 , right ).

Size and Shape of the Defect
The size of any channel between the ventricles is obviously important in determining its hemodynamic consequences. Size can be described according to taste, either using subjective adjectives such as large, medium, or small or by relating the different dimensions of the plane of space measured as the defect to the diameter of the aortic root. In this regard, defects less than one-third the aortic valve annulus are considered small, whereas those greater than one-half the aortic valve annulus are considered large. The shape of the defect may also vary. Muscular defects are typically round or oval when seen from the right ventricle, although they can also be crescentic or cashew-like. Defects that are bordered by atrioventricular valves in continuity with arterial valves, or arterial valves in isolation, are typically half-moon shaped. The shape of the defect may appear different when it is encroached upon by prolapsing leaflets of the aortic valve. When it is not round, the defect may appear larger on one plane than on another as seen using cross-sectional and angiographic imaging.
The size of the defect plays a role in determining which channels are most likely to become smaller or to close spontaneously. Because perimembranous defects are closely related to the septal leaflet of the tricuspid valve, there is always the possibility that they may be closed by plastering down of the leaflet across the defect, with small ones being those most likely to close. Defects between the outlet components, particularly when complicated by malalignment, are unlikely to close by this mechanism. Neither are extensive inlet defects. A more frequent and closely related mechanism of closure is aneurysmal enlargement of fibrous tissue in the environs of the defect. Although often described as aneurysms of the membranous septum, it is unusual for the remnant of a membranous septum itself to be involved. In most cases the grape-like lesions are sculpted from the underside of the leaflets of the tricuspid valve. Aneurysmal prolapse of the right coronary leaflet of the aortic valve can be found in the setting of both the doubly committed and the perimembranous defects, particularly those latter defects that extend to the right ventricular outlet, when the outlet septum is markedly deficient. Sometimes the noncoronary aortic leaflet can also prolapse through the defect. Rarely, such valvar prolapse may produce partial or complete plugging of the defect and result in spontaneous closure. It can also provide a false sense of security that the defect is small when, in reality, it is a large defect. The prolapse can result in aortic regurgitation. In all of these possible mechanisms of closure or diminution in size, contraction and fibrosis around the edges of the defect are additional contributory factors.
Relationship to the Conduction System
The phenotypic variation is key to determining the location of the atrioventricular conduction axis. In the normal heart the penetrating component of the atrioventricular conduction axis passes through the central fibrous body and branches on the crest of the muscular ventricular septum (see Fig. 32.7 ) and the central fibrous body forms the posterocaudal margin of perimembranous defects. The conduction axis, therefore, will always penetrate posterocaudally. As in the normal heart, the atrial landmark to the site of penetration is the apex of the triangle of Koch. When a perimembranous defect extends to open to the right ventricular inlet, the triangle itself is displaced inferoposteriorly, but its apex still serves as the guide to the site of penetration of the conducting bundle ( Fig. 32.13A ). Having penetrated through the central fibrous body, the axis is related to the posteroinferior rim of perimembranous defects. It is much closer to the septal crest when a defect opens to the inlet, becoming more remote as the defect extends to open between the outlets ( Fig. 32.13B ). Should the posteroinferior rim be muscular, as is the case in most doubly committed defects ( Fig. 32.14 ) and in muscular outlet defects, the muscular margin will protect the conduction axis.


Morphogenesis
Almost three-quarters of a century ago, Maude Abbott commented that understanding of cardiac development should provide the basis for appreciation of the different types of congenitally malformed hearts. Thanks to the development of episcopic microscopy, we now have the evidence in hand to substantiate her prediction. The findings using this technique with regard to normal development of the ventricular septum provide evidence pertinent to the concepts used previously to differentiate and describe the phenotypes. Evidence from genetically perturbed mice provides additional evidence in this regard to support the notion that all defects can be categorized in terms of being muscular, perimembranous, or doubly committed and juxtaarterial. As already discussed in Chapter 3 , ballooning of the apical components of the developing ventricles, from the inlet and outlet components of the ventricular loop, produces the first evidence of discrete morphologically left and right ventricular chambers. As the apical components balloon, the primordium of the muscular ventricular septum develops between them ( Fig. 32.15A ).

Previous investigations had shown that the atrioventricular bundle is formed on the crest of the developing muscular septum. These studies, by charting the location of the bundle and following the relationship between the crest of the septum and the insulating plane between the atrial and ventricular septa, showed that postnatally the initial muscular septum gives rise to the entirety of the muscular ventricular septum. It had been suggested that an “atrioventricular canal septum,” along with a “conal septum,” were part of the definitive muscular ventricular septum. Absence of the alleged “atrioventricular canal” component was used to justify description of the perimembranous inlet defects associated with either atrioventricular septal alignment or malalignment as an “atrioventricular canal” or type III defect. As shown in Chapter 3 and as discussed in Chapter 31 , a septum is indeed formed to divide the atrioventricular canal. It is formed subsequent to muscularization of the mesenchymal cap of the primary septum and the vestibular spine. This component, however, subsequent to the completion of atrial septation, is sequestrated on the atrial aspect of the insulating plane. It does not form part of the muscular ventricular septum. (see Fig. 32.15B ). The sequestration of the atrioventricular canal myocardium and the separation of the atrioventricular junctions by growth of the vestibular spine accompany the process of expansion of the canal. This, in turn, involves remodeling of the initial embryonic interventricular communication such that the right atrial cavity is placed in direct communication with the developing right ventricle. Subsequent to this stage of development, however, the outflow tract remains supported only by the developing right ventricle (see Fig. 32.16 , left ), even though its proximal part is already being divided into potential aortic and pulmonary components by fusion of the outflow cushions (see Fig. 32.16 , right ).

It is only with ongoing development that the aortic component of the outflow tract is moved dorsally and leftward. This process also brings the fused proximal outflow cushions in line with the crest of the muscular ventricular septum, thus walling the aorta into the left ventricle. And only when the aortic root is committed to the left ventricle is it possible for the developing embryo to close the persisting embryonic interventricular communication. This is achieved by the growth of so-called tubercles from the ventricular surfaces of the atrioventricular cushions, with the tubercles subsequently becoming the membranous part of the ventricular septum ( Fig. 32.17A ). After the completion of septation, the core of the fused outflow cushion mass is converted into an area of fibroadipose tissue. This area separates the muscularized surface of the cushions from the aortic root (see Fig. 32.17B ). The muscularized surface becomes the freestanding muscular infundibular sleeve, also known as the pulmonary conus. Because of the attenuation of the core of the initial embryonic cushion mass, there is no “conal” or “outlet” ventricular septum to be found in the normal postnatal heart. The postnatal ventricular septum, therefore, has only muscular and membranous components, with the muscular part derived exclusively from the initial septum formed between the ballooning apical ventricular components.

The sequence of development as thus described provides the basis for understanding the abnormal processes that permit the persistence of interventricular communications. It is often thought that the initial trabeculations, which make up the larger parts of the ventricular walls at the early stages (see Figs. 32.15 to 32.17 ), coalesce to form the compact component of the definitive walls. This is not the case. The compact walls are formed by proliferation of the outer myocardial layer subsequent to provision of direct vascularization by coronary arteries connecting with the aortic root. The initial trabeculations, nonetheless, do coalesce to produce the papillary muscles. The appearances as revealed in the episcopic datasets also suggest that the trabeculations coalesce to form the muscular ventricular septum. Interrogation of the mice in which the Furin enzyme had been perturbed, furthermore, shows that incomplete coalescence of the trabeculations can produce defects within the muscular septum ( Fig. 32.18 , left ).

Such defects can be expected anywhere within the muscular septum, thus explaining the varied location of muscular defects. Incomplete fusion of the muscularized core of the outflow cushions with the crest of the muscular septum accounts for the presence of muscular outlet defects. Failure of closure of the embryonic interventricular communication provides the explanation for the perimembranous defects, with examples of the central defect being found in the mice with perturbation of the Furin enzymes (see Fig. 32.18 , middle ). The experiments with perturbation of the Furin enzyme also produced mice with doubly committed and juxtaarterial defects. Interrogation of the episcopic datasets has shown that this was the consequence of failure of muscularization of the fused proximal outflow cushions (see Fig. 32.18 , right ). The sequences of normal development and the perturbation of these normal processes, therefore, now provide a rational explanation for all the known phenotypes. Failure of complete expansion of the atrioventricular canal, for example, accounts for the perimembranous inlet defects with atrioventricular septal malalignment. Incomplete or excessive transfer of the aortic root provides an explanation for the various lesions encountered with malalignment of the outlet septum, which can either be muscular or fibrous depending on the extent of muscularization of the proximal outflow cushions.
Pathophysiology
The pathophysiology of ventricular septal defect is primarily determined by the size of the defect and the state of the pulmonary vascular resistance. The site of the hole between the ventricles has little influence. Between them, size and resistance govern the direction and magnitude of flow through the defect and thus the clinical features and symptomatology.
Effect of Size
The size of the defect is crucial. Below a critical size, the defect itself presents resistance to flow through it, which controls the magnitude but not the direction of the shunt. Above this critical size, there is no appreciable resistance to flow. Both the magnitude and direction of flow are determined by the level of pulmonary vascular resistance. There are few data concerning this critical size. Some authorities have argued, unsupported by scientific investigation, that defects smaller than the aortic orifice are restrictive. Others have attempted to quantify the critical size by comparing the diameter of the defect with the body surface area (BSA), suggesting that the key value was a diameter of 1 cm/m 2 BSA, corresponding to an orificial area of approximately 0.8 cm 2 /m 2 BSA. Defects having ratios smaller than this were restrictive and produced minor or no hemodynamic changes. This suggests that the critical size is smaller than the aortic orifice, since a normal aortic valvar orifice is calculated to be approximately 2 cm 2 /m 2 BSA. In practice, the judgment of the size of a defect is generally made on hemodynamic grounds. When there is a left-to-right shunt and there are no other associated anomalies, such as pulmonary stenosis, a large defect is one that permits equalization of systolic pressures in the two ventricles, and the defect is also unrestrictive. Since the right and left ventricles do not contract exactly simultaneously, there is always some inequality in the ventricular pressures. Consequently, throughout the greater part of systole, the pressure difference will promote left-to-right shunting. During isovolumic relaxation, additional right-to-left shunting occurs, which is cleared by the subsequent diastolic left-to-right shunt unless right ventricular ejection is obstructed or there is a significant elevation of pulmonary vascular resistance.
A restrictive defect, therefore, is one in which right ventricular and pulmonary arterial systolic pressures are lower than those in the left ventricle and aorta. The size of such defects may vary from those that are just restrictive, allowing some elevation of right-sided pressures, to small defects in which the right-sided pressures are normal. In effect, defects can be considered either restrictive or unrestrictive. In those that are restrictive, there is a continuum of size between those large enough to allow a serious hemodynamic disturbance and those so small that there is only a small left-to-right shunt, with normal right-sided pressures.
Effects of Pulmonary Vascular Resistance
The effect of pulmonary vascular resistance on the flow through restrictive defects is always secondary to the size of the hole. The smaller the defect, the lower the flow through it. When defects are unrestricted, the pulmonary vascular resistance and, to a lesser extent, systemic vascular resistance is the controlling factor. When the pulmonary vascular resistance is low, the flow through the defect and the flow of blood to the lungs will be high. Such a high flow is not present at birth. It takes a finite time for the pulmonary resistance to fall from the high intrauterine to the normal postnatal levels; this is usually accomplished in the normal infant within the first 2 weeks of life.
This fall may be delayed and limited in its extent in infants with a large ventricular septal defect. Contributory structural factors include limited postnatal growth of the lungs and limitation of the number of intra-acinar blood vessels. In addition, there is hypertrophy of the muscular coat of the intra-acinar arteries and veins (see Chapter 6 ). It is not entirely clear why growth of the lungs and the proliferation of pulmonary vessels is limited. Muscular hypertrophy of the walls of the vessels is probably secondary to the increased pulmonary flow. Such high flow to the lungs leads to increased left atrial pressure, which is known to cause pulmonary vasoconstriction in experimental animals. If the vasoconstriction is maintained, vascular muscular hypertrophy will presumably occur. This delay in the fall of pulmonary resistance means that the maximal hemodynamic effects of an unrestrictive defect may not be reached for some weeks after birth. The time and course and the extent of the fall in pulmonary resistance are all variable. The maximal flow through the defect is usually achieved between 1 and 6 weeks of age. This is usually enough to allow a very high pulmonary flow, but the fall in pulmonary vascular resistance may be so limited that little flow occurs through the defect. Such patients may escape detection until the effects of severe pulmonary vascular disease have become apparent.
Once it has fallen to its lowest value, pulmonary vascular resistance may increase again with the development of the pathologic changes of pulmonary vascular disease. This usually occurs only in patients in whom the pulmonary arterial pressure is high from birth. In consequence, it is almost entirely confined to those with an unrestrictive defect. As the resistance rises, flow across the defect decreases. When pulmonary vascular resistance exceeds that in the systemic circuit, the flow through the defect will reverse its direction; hence it will flow from right to left. Thus the level of resistance determines the direction of flow. Secondary effects of severe pulmonary vascular disease include enlargement of the right ventricle and the pulmonary trunk and dilation of the infundibular attachments of the leaflets of the pulmonary valve. These may cause detectable but usually hemodynamically insignificant pulmonary regurgitation.
When defects are restrictive, there is a wide spectrum of restrictiveness. In many the defect is so small that the pulmonary vascular resistance has little or no effect on the magnitude of flow through it. In others the defect is restrictive, not allowing equalization of pressures between the two ventricles, but it is still big enough to permit a significant elevation of right ventricular and pulmonary arterial pressures. In these defects, the level of pulmonary vascular resistance will play a significant albeit subordinate role in controlling the magnitude of flow of blood to the lungs. As mentioned previously, between the two extremes of restrictive defects, there is a continuum of size and therefore of pathophysiologic effects.
Effect of Obstruction of the Pulmonary Outflow Tract
All the discussion thus far has presumed an unobstructed pulmonary outflow tract. If there is coexisting obstruction within the right ventricular outflow tract, either valvar or subvalvlar, the resistance to ejection from the right ventricle will have a similar effect to elevation of the pulmonary vascular resistance. The flow through an unrestrictive defect and thus flow to the lungs will be limited in proportion to the severity of the obstruction. In restrictive defects, obstruction to the right ventricular outflow will result in elevation of right ventricular pressure, again in proportion to the severity of obstruction. In extreme cases, the right ventricular pressure may come to exceed that in the left ventricle. As in unrestrictive defects, obstruction of the pulmonary outflow tract in the larger of the restrictive defects will also have a limiting effect on the magnitude of flow. There will be a reversal in the direction of the shunt in all cases where right ventricular pressure exceeds pressure in the left ventricle. Although pulmonary valvar obstruction may be present unequivocally from birth, subvalvar obstruction can be acquired.
Response of the Heart to Flow Between the Ventricles
The cardiac effects of a ventricular septal defect depend initially on the magnitude of flow to the lungs. With florid pulmonary flow, usually in the setting of unrestrictive defects, left atrial and left ventricular end-diastolic volumes are increased and left ventricular muscle mass is also increased. Studies of pressure and volume in the left ventricle demonstrate the marked increase in left ventricular work imposed by a large defect. This necessitates left ventricular hypertrophy as a compensatory mechanism. With extreme pulmonary flow, there is also an increase in right ventricular dimensions. Because of the elevated right ventricular pressure, there will be an even more marked increase in right ventricular work. This results in additional right ventricular hypertrophy and may be one mechanism for the development of subvalvlar pulmonary stenosis.
Left ventricular work is increased by restrictive defects in relation to the flow to the lungs, but the right ventricle is relatively spared. Consequently there is left ventricular hypertrophy but little increase in right ventricular size or muscle mass. With elevation of pulmonary vascular resistance or development of right ventricular outflow obstruction, left ventricular work is diminished because of the decrease in left-to-right shunting and pulmonary flow. The elevation of right ventricular pressure results in right ventricular hypertrophy, which dominates the picture.
The pathophysiologic effects of a ventricular septal defect can, in three situations, result in congestive cardiac failure. The first is an unrestrictive defect with high pulmonary flow when the compensatory mechanisms are insufficient to provide an adequate systemic flow. These compensatory mechanisms include recruitment of sarcomeres to operate at an optimal end-diastolic length, muscular hypertrophy of right and left ventricles, and increased catecholamine drive to the ventricles. Those circumstances are usually reached before the age of 6 months. The second situation occurs much later. It is encountered when the right ventricular myocardium has undergone degenerative changes as a consequence of long-term ejection against high resistance. This is found with pulmonary vascular disease and with prolonged and severe obstruction to the right ventricular outflow tract. The third situation is when a ventricular septal defect is complicated by aortic regurgitation, imposing a further volume load on the left ventricle and outstripping the compensatory mechanisms, as discussed earlier. It is most frequently seen in older children and adults.
Clinical Features
Presentation
The typical murmur of a ventricular septal defect is rarely heard at birth, since it takes time for the pulmonary vascular resistance to fall from the high levels of fetal life—which are sufficiently high to limit the flow through even an unrestrictive defect—to levels insufficient to provide an audible murmur. The diagnosis can now be made during fetal life by means of echocardiographic screening. Even when cases are diagnosed during fetal life, it is necessary to exercise caution, since many subsequently close spontaneously. Very few, if any, of those diagnosed during fetal life will have the full clinical picture at birth. If diagnosis is made in the neonatal nursery, it is almost invariably during examination of the baby just prior to maternal discharge. At the end of the first week of life, when many murmurs are detected, it is rare for the pulmonary vascular resistance to have fallen sufficiently for the child to be symptomatic. Symptoms, when they occur, are related to the high flow of blood to the lungs, diminished pulmonary compliance, and elevation of the left atrial pressure as a result of the high pulmonary flow. This initially causes tachypnea, which progresses with the onset of congestive cardiac failure to produce dyspnea on effort, most obvious when the baby feeds. In a baby with a typical unrestrictive or large restrictive defect, the parents are likely to complain that feeds take progressively longer over the first month or two of life and lead to rapid exhaustion of the baby, often with profuse diaphoresis. Not only is the feeding prolonged but it does not provide sufficient caloric intake for weight gain. With the development of severe cardiac failure, the baby will become obviously dyspneic even at rest. Intercostal, subcostal, and supraclavicular retractions are readily seen. This increased work of respiration imposes an increased requirement for energy, which is not satisfied because of the difficulty of feeding, a vicious cycle resulting in failure to thrive. Failure to thrive is, indeed, an alternative mode of presentation in infancy. It occurs in those babies with defects large enough to allow high pulmonary flow but in whom the size of the defect, or the level of pulmonary vascular resistance, prevents the development of intractable congestive cardiac failure. Another association with high pulmonary flow is an increased susceptibility to respiratory infection, such as frequent viral upper respiratory infections. It may be during such an episode that a murmur is noted and even that cardiac failure is precipitated.
A small and restrictive defect may be detected at any age by the discovery of a murmur. But such a defect will rarely if ever cause symptoms. When a defect is found in this fashion after the age of 1 year, it is highly unlikely that, in itself, it will ever cause congestive cardiac failure. No such assurance can be given when the discovery occurs in early infancy. There is a further small group of patients with ventricular septal defect who escape detection in infancy. They present with diminished effort tolerance and cyanosis during middle childhood or adolescence. These patients usually have severe pulmonary vascular disease. Even careful history taking fails to elicit evidence of symptoms during infancy. It is probable that at no stage did their pulmonary vascular resistance fall to levels that permitted sufficient left-to-right shunting to allow recognition of the defect.
Another alternative and rare presentation is with respiratory symptoms from bronchial compression caused by grossly enlarged pulmonary arteries. Typically the right middle lobe bronchus is compressed, but the left main and upper lobe bronchi may also be involved. This presentation is most frequently seen in patients with severe pulmonary vascular disease or in those with so-called absent leaflets of the pulmonary valve.
Infants with holes between the ventricles, therefore, rarely present in the first days of life. As highlighted earlier, the clinical presentation and clinical course are dictated by the interplay between the size of the defect, pulmonary vascular resistance, and any concomitant lesions. These factors determine the magnitude of flow, which itself directs the presentation.
Physical Examination
The appearance of the patient is again dependent on the magnitude of flow through the defect. Those with small and restrictive defects are generally entirely normal. With large restrictive defects but without frank congestive cardiac failure, patients tend to be small and thin for their age, with evidence of dyspnea such as intercostal recession. Chronicity is suggested by a depression at the insertion of the diaphragm. There will also be bulging of the left chest, indicating cardiomegaly. In the presence of large unrestrictive defects, similar appearance of more severe degree will be seen without the evidence of chronicity. Cyanosis will not be seen except in those older patients with severe pulmonary vascular disease or in those with severe obstruction within the right ventricular outflow tract.
A systolic thrill is frequently palpated in these patients except those with tiny muscular or perimembranous defects. When a thrill is present, it is localized to the second, third, and fourth intercostal spaces at the left sternal border. If the thrill is maximal in the first intercostal space or higher and yet the auscultatory features are otherwise typical of a ventricular septal defect, the likelihood is that the defect is doubly committed and juxtaarterial. There are usually no other palpable abnormalities in the presence of small restrictive defects. When the defect is large with high flow, the cardiac impulse will be hyperdynamic and the thrill may be more widespread. In the presence of severe pulmonary vascular disease, the striking features are a localized left parasternal heave of right ventricular type and a palpable second sound. The peripheral pulses are normal if there is an isolated defect.
The most typical auscultatory finding, a loud pansystolic murmur localized to the second and third left intercostal spaces, is typical of many ventricular septal defects with a left-to-right shunt, even when they are small and restrictive. The murmur starts with the first sound and continues up to the second sound. If the murmur starts with the first heart sound but stops short of the second, the defect is likely to be a small muscular one closing in late systole. Large unrestrictive defects in infants sometimes give rise to a shortened ejection systolic murmur that is not accompanied by a thrill. It is said that a pansystolic murmur may continue past the aortic component of the second heart sound, although this may be difficult to appreciate. In general, the higher the velocity of flow through the defect, as when it is restrictive, the higher the pitch of the murmur. When flow to the lungs is limited by pulmonary resistance, the systolic murmur is abbreviated and may be completely absent. In such cases, an early diastolic murmur of pulmonary regurgitation may be heard, as well as a pulmonary click. In patients who have excessive flow to the lungs, the flow through the mitral valve is sufficient to produce a middiastolic murmur at the apex. The presence of this murmur is taken to indicate that pulmonary flow is more than twice the systemic flow. In patients with no other signs of pulmonary vascular disease, the appearance of a high-pitched early diastolic murmur is highly suggestive of the onset of aortic regurgitation.
In the absence of pulmonary vascular disease, the heart sounds are normal. The pulmonary component of the second heart sound is louder in patients with pulmonary hypertension. In those with severe pulmonary hypertension, the increased intensity is easy to detect. In less severe cases, the fact that the second heart sound is audibly split at the apex indicates that the pulmonary component is louder than normal. Development of this finding during follow-up should alert to the possibility of development of pulmonary vascular disease. The second heart sound becomes single once severe pulmonary vascular disease has developed.
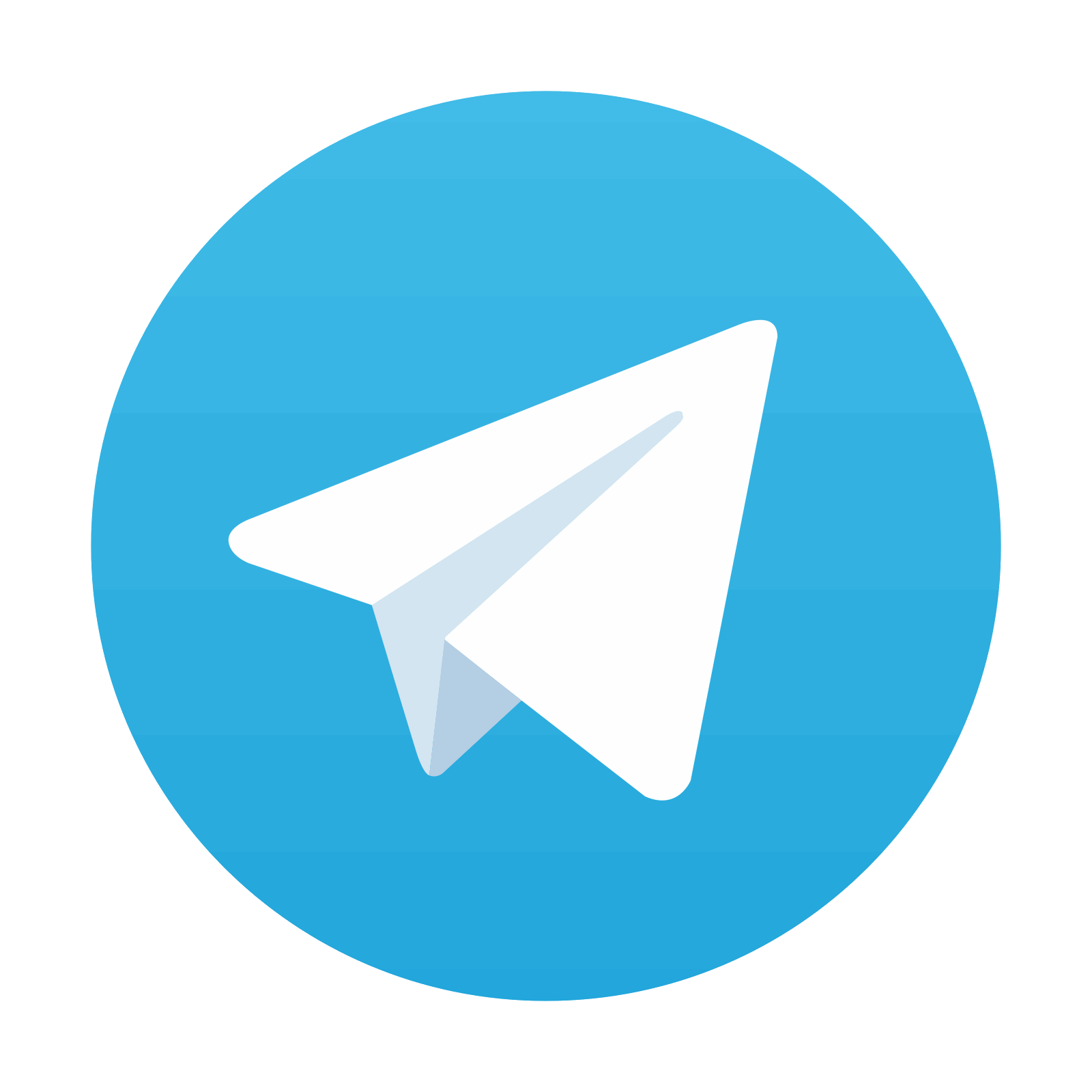
Stay updated, free articles. Join our Telegram channel

Full access? Get Clinical Tree
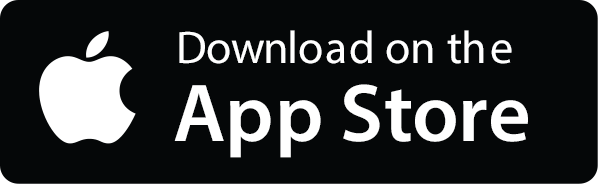
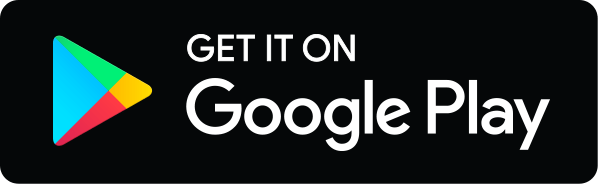