The electrocardiographic concept of chamber enlargement includes wall hypertrophy, chamber dilation, and a combination of the two. Anatomically, the term “ventricular hypertrophy” refers to an increment in myocardial mass and fiber size, while “dilation” is an increase in the volume of the internal cavity. The electrogenesis of the morphologies that appear with ventricular enlargement are conditioned more by wall hypertrophy than cavity dilation, the opposite of what occurs in atrial enlargement. On the other hand, for years it has been affirmed (Sodi‐Pallares 1956) that ventricular block homolateral to the cavity involved influences the morphology. In any case, it is clear that the modifications undergone in the P, QRS, and T loops account for the morphological alterations found in the 12 ECG leads. Mild or even moderate degrees of ventricular enlargement may not affect ECG results. When heart disease involving the ventricles is not very important, such as the right ventricle with mild pulmonary stenosis, or of the left ventricle with mild aortic stenosis, it can progress for some time without ECG signs of ventricular enlargement, despite the fact that the chamber enlargement has already produced some hemodynamic overload. On the other hand, with the passing of time, often the same severity of the disease responsible for the enlargement will more or less alter the ECG, signaling the stage of the disease (see below). Echocardiography is a sensitive, innocuous, and reproducible method for determining left ventricular dilation and left ventricular mass. Its reliability is comparable to that of anatomic and angiological studies (Reichek and Deveraux 1981), and it provides more exact information than isotopic studies (Subirana et al. 1981). The dimensions of the right ventricle can be even better measured using two‐dimensional echocardiography (Bommer et al. 1980). Cardiovascular magnetic resonance imaging (CMR) is a reproducible standard of reference technique for the assessment of ventricular mass and volumes (Sheridan 1998). The correlation between CMR left ventricular mass measurements and post‐mortem left ventricular weights in humans has a correlation coefficient with a range of 0.95–0.99 (Allison et al. 1993). CMR results correlate closely with those of echocardiography with possibly even greater accuracy in the measurement of ventricular mass (Reiter et al. 1986). Diagnostic and prognostic utility of the ECG for left ventricular enlargement (LVE) defined using CMR imaging in relation to ethnicity has been studied (Jain et al. 2010). It has been demonstrated that the ECG has a low sensitivity but high specificity with some race‐related differences, if the echocardiography is the standard reference technique. However, we are not aware that any systematic comparison of the two techniques (echocardiography and CMR) exists in order to better determine the accuracy of ECG criteria for ventricular enlargement. The ventricles are subject to two types of hemodynamic overload: systolic and diastolic. They suffer systolic overload when ventricular emptying is impaired. In the left ventricle, this occurs with aortic stenosis, aortic coarctation, and arterial hypertension, as well as in some cases of ischemic heart disease. In the right ventricle, systolic overload occurs with pulmonary stenosis and pulmonary hypertension. The ventricles suffer diastolic overload when there is excessive diastolic filling, phenomenon that occurs in the left ventricle with aortic regurgitation and in the right ventricle with atrial septal defect (ASD). Cabrera and Monroy ( 1952) coined the electrocardiographic terms “systolic overload” and “diastolic overload” based on this hemodynamic concept. They report that there are ECG morphologies characteristic of these two types of ventricular overload. According to this study, systolic overload is manifested in the right ventricle by a high‐voltage R morphology in V1 with a negative asymmetric T wave, while in the left ventricle, it is manifested by a high‐voltage R wave in V5–V6, again with a negative asymmetric T wave. Diastolic overload in the right ventricle, in contrast, is manifested by the rSR′ pattern in V1 with a negative asymmetric T wave and by a high‐voltage qR morphology with a positive, tall, symmetric, and peaked T wave in the left ventricle. Our opinion is that while Cabrera’s terminology is applicable in some cases, its indiscriminate use is not correct. It may be considered that, regardless of the underlying heart disease, the ECG morphology of Cabrera’s diastolic overload usually corresponds to mild or moderate stages of right or left ventricular enlargement, while the systolic overload morphology usually reflects advanced stages of ventricular enlargement (“strain pattern”) (Bayés de Luna 2012). Probably, correlation exists between the changes in myocardial fibers that produce myocardial “strain,” detected with imaging techniques, with the electrophysiological changes that exist when LVH develops the ECG pattern of “LVH with strain” (electrical remodeling). A new conceptual model has recently been developed in which the structural, bioelectrical, and biochemical changes are interconnected and may explain ECG changes and have prognostic implications (Bacharova et al. 2010, 2011). Right ventricular enlargement (RVE) is manifested in patients with systolic overload (pulmonary stenosis, pulmonary hypertension usually due to mitral valve disease) by wall hypertrophy (right ventricular hypertrophy), which in advanced cases is associated with some degree of dilation (right ventricular dilation). Dilation appears earlier in heart diseases with hemodynamic diastolic overload, such as ASD. However, in some processes (pulmonary embolism or acute decompensation in a patient with chronic obstructive pulmonary disease (COPD)), acute right ventricular dilation can appear without evident wall hypertrophy, which occurs rarely in the left ventricle. RVE is observed mainly in infants and children with diverse types of congenital heart disease (pulmonary stenosis, ASD, pulmonary hypertension, Ebstein’s disease, etc.) and in adults with valvular heart disease (historically, mitral stenosis with right heart repercussion in particular) or some type of cor pulmonale. The existence of any type of RVE counteracts more or less the normally dominant left ventricular forces, directing the dominant forces (QRS loop and vector) to the right and forward or backward (Figure 10.1). Associated right ventricular conduction delay influences the presentation of RVE morphology. Consequently, it is not necessary that the right ventricular mass exceed the left in order for the ECG morphology of RVE to appear. The resultant depolarization vector is directed to the right because of the following association: increase in mass plus conduction delay. In global and significant RVE, the direction of repolarization is usually altered due to the fact that the right ventricular subendocardium begins to repolarize when subepicardial wall depolarization has not yet concluded, and due to the presence of some degree of right ventricular block. As a result, the T loop and corresponding T vector often follow the opposite direction to the QRS loop, which is analogous to what occurs in the left side in advanced left ventricular hypertrophy (LVH). It should be remembered that it is difficult, if not impossible, to make an ECG diagnosis of mild RVE because the powerful left vector forces overwhelm mild, and even moderate, degrees of RVE. Therefore, the specificity and especially the sensitivity of the ECG criteria for the diagnosis of mild and moderate RVE are lower than those for diagnosing LVE. The QRS loop in RVE has different morphologies, but mainly it is directed to the right and forward or backward (Figure 10.2) (see later). Projection of these loops on the frontal and horizontal planes (on the positive and negative hemifields of the different leads) illustrates the ECG modifications seen in RVE (Figures 10.2 and 10.3). Figure 10.1 Right ventricular enlargement (RVE) counteracts the usually dominant forces of the left ventricle (A), shifting the dominant forces to the right (B), sometimes forward and other times backward (B). Figure 10.2 Top. Parting from a normal loop in the horizontal plane. Progressive degrees of right ventricular enlargement (RVE) always produces a rightward shift in direction, sometimes forward (A) and other times backward. (B) If the loop moves forward, (A) it produces different QRS morphologies in V1 as it becomes progressively more anterior, with R gradually becoming taller and T more negative (from I to V). Often, the loop begins rotating counterclockwise and ends up rotating clockwise, producing an rSr′ morphology in V1 identical to that seen in partial right ventricular block. (B) If the RVE directs the loop to the right and backward, a normal morphology (rS) or QS or rSr′ can be seen in V1, always with a marked S in V6 (VI and VII). This type of loop is seen in global RVE in particular, in cases of left valvular heart disease with pulmonary hypertension and in congenital heart diseases with evident RVE. With time, RVE increases in intensity and the QRS loop becomes progressively more anterior, with its final portion directed more to the right. In the first phase, it conserves the counterclockwise rotation in the horizontal plane (Figure 10.2I). Later, when the whole loop becomes anterior and more to the right, it rotates in a figure‐of‐eight or clockwise pattern (Figure 10.2II–V). Figure 10.3 Diagram of the QRS loops in the frontal plane (FP) and horizontal plane (HP) in patients with right ventricular enlargement (RVE). B corresponds to the type of moderate RVE seen in mitral stenosis, while A corresponds to severe RVE as seen in severe pulmonary stenosis. C and D correspond to the RVE frequently seen in cor pulmonale, with right ÂQRS (C) or SI, SIII, SIII (D). The SI, SII, SIII morphology is also encountered in some cases of zonal right ventricular block, and in normal individuals. In cor pulmonale, the SI, SII, SIII morphology is probably due to late depolarization of the anterosuperior subpulmonary zone of the right ventricle, which is caused by RVE. This loop shows a clockwise rotation in the frontal plane and all the loop located more anteriorly (Figure 10.2A1). This loop is frequently observed in mitral stenosis with mild pulmonary hypertension, although it can be seen in almost all heart disease with mild global RVE. The ECG changes (Figures 10.2–10.4) seen are as follows: The QRS loop has a figure‐of‐eight rotation in the horizontal plane in type II, a predominantly clockwise rotation in types III and IV, and an exclusively clockwise rotation in type V. In the frontal plane, a progressively larger part of the final portion of the loop is observed in the negative hemifield of lead I (Figure 10.3B). The type IV and V loops of Figure 10.2 are seen in severe global RVE (severe pulmonary stenosis or severe pulmonary hypertension). The loop rotates clockwise and a large part of the loop is directed to the right (Figures 10.2 and 10.3B). In the types II and III of Figure 10.2, a lesser part of the loop is directed to the right and the frontal plane loop morphology falls between types A and B of Figure 10.3. The ECG changes in the frontal plane are as follows (Figures 10.2, 10.3–10.5): The ECG changes in the horizontal plane are as follows: The rsR′ morphology in V1 with a negative, asymmetric T is most often seen in ASD, which evolves more with right ventricular dilation than with hypertrophy (Figures 10.2II and 10.7). However, it can also be seen in other heart diseases with mild RVE, such as mild pulmonary stenosis (Figure 10.4C‐9). Figure 10.4 Right ventricular enlargement (RVE) is fundamentally seen in three types of processes: (A) valvular diseases with right repercussion, especially mitral stenosis; (B) lung diseases with right repercussion (cor pulmonale); and (C) certain congenital heart diseases. In (A), we can see different V1 morphologies, as well as ÂP, ÂQRS, and V6 morphologies, in different cases of mitral stenosis with associated RVE: (1 and 2) Mitral stenosis with mild pulmonary hypertension. (3) Mitral stenosis with moderate pulmonary hypertension and functional tricuspid regurgitation. (4) Mitral stenosis with severe pulmonary hypertension. The same characteristics can be seen in (B) in different types of cor pulmonale. Cases (5) and (6) correspond to chronic cor pulmonale secondary to chronic obstructive pulmonary disease (COPD) in elderly patients. Cases (7) and (8) are two cases of subacute cor pulmonale in young patients with severe pulmonary hypertension. (C) Several cases of congenital heart diseases: (9) A 9‐year‐old girl with mild pulmonary stenosis. (10) A case of mild pulmonary stenosis in a young boy. (11) Moderate pulmonary stenosis in a 10‐year‐old girl. (12) Severe pulmonary stenosis in a 16‐year‐old female. (13) Ostium secundum‐type atrial septal defect (ASD) with mild pulmonary hypertension in an 8‐year‐old girl. (14) Ostium secundum‐type ASD with important pulmonary hypertension in a 29‐year‐old woman. (15) A 10‐year‐old girl with typical Fallot’s tetralogy. (16) A 15‐year‐old boy with a typical Eisenmenger syndrome. Observe how in the three situations (A, B, and C), morphologies are seen in V1 from rS or rSr′ to R alone (with high voltage), according to the severity of the disease and the degree of evolution. The solitary R morphology in V1 and usually V2 with negative, asymmetric T recorded in V1–V3 and even V4 is seen in cases of important RVE and closed interventricular septum as isolated severe pulmonary stenosis, or idiopathic pulmonary hypertension (the “barrier‐type” of the Mexican School) (Figure 10.7). In Fallot’s tetralogy (pulmonary stenosis with overriding aorta and ventricular septal defect), the morphology changes from solitary R with negative T in V1 to RS with positive T in V2, because the last part of the loop is located backward (compare Figures 10.8 and 10.9) (the “adaptation type” morphology of the Mexican School) (Sodi‐Pallares 1956). In addition, an RS morphology with a positive T wave in V1 can be seen in moderate, not long‐standing cases of Fallot’s tetralogy (Figure 10.10). Figure 10.5 Two cases of severe right ventricular enlargement (RVE), one with great dilation of right ventricle and the other with great right ventricular hypertrophy. (A) Atrial septal defect with marked right ventricular dilation (arrow). (B) Typical ECG of RVE in a 12‐year‐old patient with severe pulmonary stenosis. Figure 10.6 Typical morphology of ostium primum‐type atrial septal defect (ASD). Observe the rSr′ morphology in V1 with the ÂQRS in the frontal plane extremely deviated to the left and the ECG–VCG correlation. Other ECG features include: Throughout the evolution of right‐sided heart diseases, the QRS loop often suffers modifications, gradually passing from the type I loop to the type V loop, seen in Figure 10.2, over the years. The reverse can happen after corrective surgery in these cases (see later) (Figure 10.11). The different ECG patterns of Figure 10.4 may be seen in congenital heart disease (Figure 10.4C), mitral stenosis (Figure 10.4A), or cor pulmonale (Figure 10.4B). The patterns with solitary R in V1 are more frequently seen in congenital heart disease with concentric RVE as pulmonary stenosis or severe pulmonary hypertension (Eisenmenger syndrome, Figure 10.4C‐12 and C‐16) than in mitral stenosis or ASD because these latter heart diseases usually present with milder RVE. However, if associated severe pulmonary hypertension is present, the same morphologies with solitary or predominant R can also be seen in V1 in patients with mitral stenosis (Figure 10.4A‐4). Even young patients with subacute cor pulmonale and severe pulmonary hypertension may present occasionally this ECG pattern (Figure 10.4B‐8). However, old patients with severe COPD usually present with the type VI or VII QRS loops (Figure 10.2) (QS or rSr′ in V1 with rS or RS in V6), but with a rightward‐directed ÂQRS in the FP or an SI SII SIII pattern (types C and D, Figure 10.3) during the course of the disease. These types of loops (VI and VII of Figures 10.2, 10.3, 10.12, and 10.13), presenting significant posterior and right final forces, are especially seen in COPD and, more rarely, in some cases of mitral stenosis. In these cases, the RVE is located in basal posterior zones in particular, and the heart presents with a verticalized and descended position because of the low diaphragmatic situation frequently found in COPD. All these factors explain the predominance of the posterior forces in COPD (Figure 10.2VI–VII). Figure 10.7 Typical morphology of ostium secundum‐type atrial septal defect (ASD). Observe the rSr′ morphology in V1, the right‐deviated ÂQRS, and the ECG–VCG correlation. In typical cases, the QRS loop has a clockwise rotation with an open loop in the frontal plane (Figures 10.3C and 10.10), but often a folded frontal loop with a maximum axis situated at +30° to −150°, similar to the loops seen in patients with anterosuperior segmental right ventricular block and certain normal individuals (SI, SII, SIII morphology), is observed (Figures 10.3D and 10.13). The ECG changes are as follows: Because of the difficulties in finding diagnostic criteria for RVE that are both specific and sensitive, many have been described (Walker et al. 1955; Reeves 1970). Their utility largely depends on the population studied and the technique used to detect RVE. We must remember that: (i) the higher sensitivity of one criterion, the lower the specificity and (ii) criteria based on left precordial lead alterations are more sensitive but less specific, and those based on V1–V2 modifications are more specific but less sensitive. Sensitivity increases when a population with a higher incidence of congenital heart disease or advanced right ventricular involvement is studied. Likewise, the use of autopsy material for ECG correlations can distort the results because only patients with an advanced stage of the disease are studied. Therefore, an improved evaluation of the ECG criteria for RVE can be carried out when non‐invasive imaging techniques such as echocardiography and cardiovascular CMR are used to compare diagnostic results. Echocardiography has been useful in detecting right ventricular mass and volume (Bommer et al. 1980), showing a good correlation with autopsy (Prakash 1981). In the past, isotopic techniques have also been used (Subirana et al. 1981) (Figure 10.5), but echocardiography and CMR give better results because they are more reproducible and accurate. From a practical point of view, the use of CMR is at large scale now difficult in 2020 (see also LVE: Diagnostic ECG criteria). Figure 10.8 An 8‐year‐old patient with important pulmonary valve stenosis with a gradient over 100 mm Hg. The patient presents with a typical RVE morphology with a barrier‐type systolic overload (R in V1–V2 with negative T wave). The ÂP in the ECG seems deviated leftward. In fact, the patient presents with wandering pacemaker between sinus and junctional rhythm (see strip of lead III). Note the ECG–VCG correlation. We can assume that the diagnosis of RVE using the QRS criteria of right atrial enlargement (see Table 9.1; Figure 9.5) is usually certain because these criteria are usually a consequence of RVE. In Table 10.1, ECG criteria with a specificity better than 85–90% and a corresponding low sensitivity are listed (Walker et al. 1955; Prakash 1981). To enhance sensitivity, Horan and Flowers (1980) conceived a score point system (Table 10.2) based on combinations of different criteria for RVE. This score is not useful in the presence of ventricular block or lateral infarction. In daily practice, we must try to use very specific criteria (about 90% or more) (Table 10.1), despite the lower sensitivity, which will reduce the number of false‐positive diagnoses. In contrast, when making epidemiological studies or in cases of computerized or semi‐computerized ECG interpretations (Minnesota Code) (see Chapter 25), it is necessary to use more sensitive criteria for preliminary screening. To summarize, what can be affirmed is that in the presence of heart diseases with right involvement, and in the absence of RBBB, lateral infarction or Wolff–Parkinson–White (WPW) pattern, the ECG criteria shown in Table 10.1are very specific for RVE. Macfarlane et al. (1981) postulated that hybrid criteria derived from a 12‐lead ECG plus three orthogonal leads would produce a sensitivity ranging from 55% to 65%. However, this scoring system has not been popularized. Pipberger et al. (1982) used a new classification system for epidemiological studies that increased sensitivity at the expense of specificity. It may be useful to carry out the preliminary screening. We will now examine the ECG changes found when abrupt right ventricular overload accompanied by pulmonary hypertension induce right ventricular dilation. This includes cases of decompensated cor pulmonale and pulmonary embolism. Patients with cor pulmonale often present with acute hypoxemic episodes accompanied by pulmonary hypertension that are generally reversible but accompanied by alterations in the right ventricular structure or function. In these cases, right ventricular dilation can appear without ventricular wall hypertrophy, at least in the initial stages. Dilation of the right ventricle may therefore be the initial, and sometimes the only, sign of cor pulmonale (Kilcoyne et al. 1970). Figure 10.9 A 3‐year‐old patient with typical tetralogy of Fallot. The ECG corresponds to the Mexican School‐named “RVE with adaptation‐type systolic overload” (R in V1 with negative T and rS in V2 with positive T). Note the ECG–VCG correlation. Figure 10.10 A 7‐year‐old patient with moderate Fallot’s tetralogy and RS with positive T wave morphology in V1. Note the ECG–VCG correlation. Figure 10.11 (A) ECG of an 8‐year‐old patient with severe pulmonary stenosis (Rs in V1 with negative T wave). After surgery (B), the morphology regressed considerably (rSR′ in V1). Figure 10.12 A 65‐year‐old patient with important chronic obstructive pulmonary disease (COPD) and no left heart disease. There is a typical P pulmonale as well as other signs of right ventricular enlargement (RVE), with the QRS loop posterior and to the right (P negative in V1, absence of the first vector in the HP, rS in V6, ÂQRS ≥110°, etc.). Figure 10.13 SI, SII, SIII morphology in a normal case (A) and in chronic obstructive pulmonary disease with right ventricular enlargement (RVE) (B). The ECG morphologies often do not differ. In some cases, the P and T wave pattern can help distinguish them. Table 10.1 ECG criteria for right ventricular enlargement. There are certain ECG changes that lead us to suspect that the degree of pulmonary hypertension varies abruptly as a consequence of decompensation in patients with cor pulmonale. These include: Table 10.2 Electrocardiographic criteria for right ventricular enlargement in adults without conduction detects known not to have infraction. (Adapted with permission from Horan and Flowers (1980)). Interpretation of point score: These criteria do not take into account serial electrocardiographic comparisons. Such additional data may be the interpreter’s impression of the likelihood of fixed enlargement or dynamic overload. Figure 10.14 A 60‐year‐old patient with chronic cor pulmonale. Because of respiratory infection, the patient presented with an ECG morphology of acute right ventricular overload (above) that ceased in a few days (below). The most common ECG signs found in pulmonary embolism may be similar to those commonly found in acute decompensated cor pulmonale, including the following: This pattern is very characteristic of pulmonary embolism (high specificity) but does not appear frequently (low sensitivity). When it does appear, it usually corresponds to a major embolism. A differential diagnosis must be made, especially for inferior myocardial infarction (see Chapter 13). In the neonatal period, it is difficult to diagnose RVE because there is already a physiological RVE. Nonetheless, any of the following criteria suggest the existence of abnormal RVE in the newborn (Burch and DePasquale 1967; Moss and Adams 1968; Rowe and Mehrizi 1968; Fretz et al. 1992): Figure 10.15 (A) A 59‐year‐old patient with pulmonary embolism and typical McGinn–White morphology: SI, QIII, negative TIII. (B) The ECG after the patient recovered. Figure 10.16 (A) Pre‐operative ECG of a 58‐year‐old female with no heart disease. (B) During the post‐operative period, the patient presented with a massive pulmonary embolism with an ECG that showed a sharply right‐deviated ÂQRS, advanced RBBB, and sinus tachycardia. The patient died a few minutes later. Figure 10.17 Patterns of left ventricular geometry in hypertensive patients. LVMI: left ventricular mass index; RWT: relative wall thickness (Reproduced from Ganau et al. 1992). Later on and until adulthood, some of these signs undergo modifications. RVE is then suggested by: We consider the following to be indirect signs of RVE: Regression of RVE signs is frequently seen post‐surgery in patients with congenital heart diseases such as ASD or pulmonary stenosis (Figure 10.11). After correction of Fallot’s tetralogy, post‐operative ECG often shows advanced RBBB (see Chapter 11) (Figure 11.12), masking the regressive changes of the RVE. A differential diagnosis should be made with all cases that present with prominent R in V1, as a result of an anterior and mainly right‐oriented loop (Table 10.3). Table 10.3 Presence of prominent R or r′ in V1: differential diagnosis. The presence of a QRS complex without final anterior forces and consequently with rS morphology in V1 or even QS (see Figure 10.2VI), or, at most, rSr′ with a low‐voltage r′ (Figure 10.2VII), sometimes with a much deeper S wave in V2, can cause confusion between this type of RVE and left ventricular or biventricular hypertrophy, septal infarction, or zonal right ventricular block, a frequently associated pathology. We will discuss the most important ECG characteristics in these cases. In this type of RVE, the S waves are prominent until V6, there is dominant R in V3 R–V4R and in aVR there is a terminal R because often the final vector is oriented backward, but also upward and to the right (see Figure 10.3C and D). Sometimes there is a shallow S in V1 with greater voltage of S in V2, with an RS pattern until V6. In biventricular hypertrophy, a deep S in V2 with small S in V1 (shallow S inV1) may also exist, but in left precordials, there is a R or Rs pattern. In LVH, the forces are directed backward and to the left, originating a tall R wave in V5–V6 and usually a deep S in right precordials, V3R and V4R. Moreover, in the frontal plane, ÂQRS is deviated to the left and in aVR, the terminal r is minimal or not found. Biventricular enlargement diagnosis is possible when there is a much larger S wave in V2 than in V1, with R or Rs morphology in V6, and SI, SII, SIII morphology, or right ÂQRS in the frontal plane. In chronic septal infarction a negative post‐ischemic T wave is seen from V1 to V2, V3, frequently with QS or qrS in the same leads, and with an R/S ratio in V6 > 1 and a generally normal P wave. The C loop of Figure 10.3(“SI, RII, RIII”‐type) (Figure 10.12) should be distinguished from inferoposterior hemiblock (IPH) of the left bundle branch (see Chapter 11). In IPH, the q of III and aVF is more marked, the R/S ratio in V6 > I, and the atriogram is not suggestive of right atrial enlargement. The D loop of Figure 10.3(“SI, SII, SIII”‐type) (Figure 10.13) is explained by the existence of zonal right ventricular block and/or RVE (see Chapter 11). In patients with COPD, this type of loop is usually due to associated RVE with a certain degree of zonal conduction delay in the right ventricle (Bayés de Luna et al. 1987). However, the presence of the SI, SII, SIII pattern in normal individuals is likely to be caused more by a variation in the distribution of the right ventricular Purkinje fibers with some physiological delay of conduction than by a true right peripheral block (see Figure 11.19). In addition, the differential diagnosis between the SI, SII, SIII pattern and hemiblock of the superoanterior division of the left bundle branch must be made. The morphology is that of SI, SII, SIII in RVE and/or peripheral right ventricular block, with SII ≥ SIII and a low‐voltage R in I (Figure 10.3D), while in left superoanterior hemiblock (SAH), there is an exclusive R wave in lead I with high voltage and SII < SIII. These changes may be seen as the most evident findings in cases of RVE, especially in acute dilation of the right ventricle. The clinical setting may be very useful to rule out other etiologies, especially ischemic heart disease. In the presence of advanced RBBB (QRS ≥ 0.12 sec) (Figure 11.13A), RVE is suggested when the following are found: In the presence of advanced LBBB (QRS ≥ 0.12 sec), RVE is suggested (Figure 11.13B) when the following are found: The ECG diagnosis of RVE is difficult, but when achieved, it generally indicates that significant enlargement is present. The prognosis of important global RVE (tall R in V1) with narrow QRS is worse than isolated advanced RBBB (tall R in V1 with wide QRS). The differential diagnosis in patients presenting with a prominent R wave or rSr’ in V1 has been discussed previously (see Table 10.3). The sensitivity of ECG criteria for RVE is relatively low. Therefore, a normal ECG may be found in many cases of mild/moderate RVE or even acute right ventricular dilation (pulmonary embolism). However, its specificity is very high (see Tables 10.1 and 10.2). The echocardiography measurement of RV wall is more sensitive for the diagnosis of RV hypertrophy than ECG criteria (Prakash 1981). Electrocardiographic signs are fundamentally caused by hypertrophy of the ventricular wall (LVH) and are frequently associated with partial left bundle branch block (LBBB). It is sometimes possible to infer the diagnosis of ventricular dilation accompanying the hypertrophy (see later). In heart diseases with diastolic volume overload (e.g. aortic regurgitation), dilation of the cavity is associated with hypertrophy (eccentric hypertrophy) earlier than in heart diseases with systolic pressure overload, such as aortic stenosis and hypertension (concentric hypertrophy) (Ganau et al. 1992) (Figure 10.17). The acquired heart diseases that most frequently produce LVE are valvular diseases, especially aortic valve disease, systemic hypertension, and cardiomyopathies, including coronary cardiomyopathy. The congenital heart diseases that most often produce isolated LVE are aortic stenosis, aortic coarctation, and fibroelastosis. Depending on the severity and evolutive stage of the heart disease, different ECG morphologies may appear (see later). The increment in left ventricular mass enhances the already dominant vectorial depolarization forces of the left ventricle, which shift backward and often somewhat more upward, in cases of predominance of enlargement of the left ventricular free wall (Figure 10.18B). If important enlargement of the septum predominates, the vectorial forces are directed to about 0° in the horizontal plane or even somewhat forward (Figure 10.18C). In advanced LVH (the term LVH is more frequently used than left ventricular enlargement—LVE), ventricular repolarization direction changes because the subendocardium begins to repolarize when subepicardial depolarization is still incomplete. Associated left ventricular block also plays a role in this process (Piccolo et al. 1979; Bayés de Luna et al. 1983). This explains why the T loop and vector are directed opposite to the QRS in advanced LVH. It also accounts for the abnormal ST vector opposed to QRS at the conclusion of ventricular depolarization (J point). These circumstances determine that in advanced LVH, the QRS and T loops are abnormal and assume opposing directions (Figure 10.19B–E). Different types of QRS and T loops are seen in LVE. In Figure 10.19, five of the most common configurations are shown. The type A loop corresponds to mild or moderate LVH, while B‐ and C‐type loops correspond to more severe cases. Type D corresponds to the LVH sometimes observed in HCM and type E to LVH with a predominance of apical septal hypertrophy over free wall hypertrophy. This figure summarizes the morphological features of these loops. Loop projection on the frontal and horizontal planes (on the positive and negative hemifields of the different leads) accounts for the ECG alterations found in LVH, which will be described later. However, it is important to remember that mild or even some moderate degrees of LVH may not change the ECG. Figure 10.18 (A) Under normal conditions, the dominant forces of ventricular depolarization are directed downward, backward, and to the left. In cases of predominantly enlarged free left ventricular wall (B), the forces are generally directed more to the back and frequently upward, and in cases of predominantly enlarged septum (C), the forces can be directed to 0° or even somewhat forward. Figure 10.19 Diagram of the QRS and T loops in the frontal plane (FP) and horizontal plane (HP) in mild or moderate left ventricular enlargement (A) and severe enlargement (B and C). The D loop (or similar) is seen in some cases of hypertrophic cardiomyopathy, and the E loop is seen in cases of apical hypertrophic cardiomyopathy. Note in the five cases the typical morphology that appears in aVF, I, V1, and V6 according to the loop–hemifield correlation. Figure 10.20 Typical ECG of a 22‐year‐old male with important, although not very long‐standing, aortic regurgitation. It corresponds to moderate left ventricular hypertrophy but satisfies the diagnostic criteria for LVE (Romhilt–Estes score). In effect, R in V5–V6 > 30 mm (3 points); intrinsicoid deflection time (IDT) = 0.07 sec (1 point); duration of QRS = 0.10 sec (1 point). Total: 5 points. Left ventricular dilation is suggested by IDT > 0.07 sec and the R wave height in V6 greater than that in V5. When septal hypertrophy (especially in the apical zone) predominates over that of the left ventricular free wall (asymmetric septal HCM), or when there is biventricular enlargement, the maximum loop vector can be fairly anterior (at about 0°), and the QRS may even show an RS pattern in lead V2 or even V1 (Figures 10.19E and 10.25). This morphology can be confused with isolated levorotation, or all causes of high R in V1 and/or V2 in the absence of RVE (see Table 10.3). Figure 10.21 ECG of a 47‐year‐old male, typical of severe, long‐standing aortic stenosis. The left ventricular hypertrophy pattern is typical. R in V4–V5 > 30 mm (3 points); ST–T opposite to R in V4–V6 (3 points); IDT = 0.055 sec (1 point). Total: 7 points (Romhilt–Estes score). Figure 10.22 ECG and VCG in a patient with severe aortic valve disease. There is no q wave in V5–V6. Myocardial biopsy showed more than 10% of septal fibrosis. Figure 10.23 ECG and VCG in a patient with severe aortic valve disease. There is q wave in V5–V6 > 1 mm. Myocardial biopsy showed less than 10% of septal fibrosis. Figure 10.24 Typical but infrequent ECG of obstructive hypertrophic cardiomyopathy in a 25‐year‐old patient. There are no voltage criteria for left ventricular enlargement, but the deep, clean Q wave in V5–V6 and QS in the middle precordials, together with the “q” wave of the frontal plane (FP) and the absence of repolarization alterations in the leads with abnormal Q wave, suggested the diagnosis, which was confirmed by echocardiography. Very conspicuous “q” waves are observed in 10–35% of cases of HCM, with the “q” wave sometimes more prominent than the “R” wave, with QS or QR patterns appearing (Figures 10.19D and 10.24). However, the presence of a prominent Q wave attributed to septal hypertrophy is a poor predictor of septal thickness (Moro et al. 1995). It is essential to rule out the “Q” wave of necrosis. The Q waves of HCM are thinner and, although they may be deep, they are usually followed by positive T waves (Figure 10.24) (see Chapter 13). Figure 10.25 ECG and VCG of an asymptomatic patient who had been diagnosed with coronary artery disease because of deep negative T waves. The ECG shows a morphology characteristic of hypertrophic cardiomyopathy, with mainly apical involvement (tall R without “q” in V5–V6 and very deep negative T waves in different leads). The VCG shows the typical T loop morphology (“arrow point”) directed to the back and right. (A: global loop, B: T loop, C: initial vectors). Two factors contribute to the appearance of repolarization alterations: the severity of the lesion and the duration of its evolution (Figures 10.19, 10.25, 10.26, and 10.27B). In valvular disease, as well as in hypertension or cardiomyopathies, the ST–T changes are determined especially by the duration of evolution. Figure 10.26 Four typical examples of morphology evolution. (A and B) are two cases of important aortic stenoses, the second lacking the q wave from the first ECG, and the first case with a progressive reduction in q depth coinciding with the repolarization “strain pattern.” (C) shows advanced aortic regurgitation with a progressive decrease in the q wave with the appearance of “strain pattern,” while in (D), we see a patient with ischemic and hypertensive heart disease. As the ECG evolves, we can observe the changes in the ECG patterns (see text). In the last 60 years, many ECG criteria for LVE have been proposed (Sheridan 1998; Van der Wall et al. 1999). In the beginning, most were based on radiological and necropsic studies, followed by echocardiographic correlations. It has been demonstrated (Reichek and Deveraux 1981) that left ventricular mass measured by echocardiography correlates with anatomic post‐mortem weight, showing a high sensitivity (93%) and specificity (95%). Figure 10.27 (A) ECG if a healthy, lean, adolescent and (B) if a patient with hypertension. Observe the mild differences in the ST segment/T wave. In (A), there is from the beginning an upsloping of the ST segment (V4–V6, I, and II). In contrast, in (B), there is a rectified ST with symmetric T wave (V5, V6, I, II, VF). Figure 10.28 Four examples of atypical morphology of ST/T according to the concept of systolic and diastolic overload. The second is an aortic stenosis that looks like aortic regurgitation (there is “q”), while the first is an aortic regurgitation that mimics stenosis (exclusive R wave without “q”). The last two cases correspond to double aortic valve disease (stenosis + regurgitation), one without q and the other with very conspicuous q. The first case with evident septal fibrosis and the second with mild septal fibrosis (see text). These last two cases show very altered repolarization because a primary repolarization disorder appeared during the course of the secondary disorder, due to ventricular enlargement. Using echocardiographic validations, the ECG criteria may obtain, at least in some subsets of patients, a sensitivity of about 50% while maintaining a 90% specificity.M‐mode echocardiography is very useful in assessing left ventricular mass in patients with normal cardiac shape, as is the case in most hypertensive patients. Using two‐dimensional echocardiography, left ventricular mass can be accurately assessed, even in patients with distorted left ventricular geometry due to myocardial infarction or RVE. CMR is the ideal imaging technique for the assessment of left ventricular mass and volume, with good reproducibility and probably even greater measuring accuracy than echocardiography. In ventricles of normal shape, including those found in athletes, both echocardiography and CMR are the “gold standard” technique for accurately assessing cardiac dimensions, mass, and volume with a correlation close to 100%. However, CMR is more precise, accurate, and reproducible, especially in patients with distorted left ventricular (IHD patients with myocardial infarction scars). The echocardiography is cheaper and has better time resolution, but it is more expertise‐dependent than CMR. Currently, the definitive correlation of the two techniques to certify the ECG criteria for the diagnosis of LVE is lacking. For all that, CMR is unrealistic for large‐scale use in clinical practice. The ECG has a high specificity (SP ≈ 90%) comparable to echocardiography (few false positives), but the sensitivity (SE) of the ECG is much lower (more false negatives), especially in early stages of LVH. The SE increases very much according to the severity of LVH in the population studied (Schillaci et al. 1994) (Figure 10.29). However, it is important to remember that the ECG is a superior predictor to echocardiography for cardiovascular complications. Table 10.4 ECG criteria for left ventricular hypertrophy according to ECG and echocardiography (*) and cardiovascular magnetic resonance imaging (**) correlations: sensitivity, specificity, and cardiovascular risk of death (see text). Figure 10.29 Three‐dimensional bar graph showing sensitivity of the ECG criteria (Cornell voltage, Romhilt–Estes score, left ventricular strain) according to quartiles of severity of LVH (left ventricular mass assessed by echocardiography). (Reproduced with permission from Schillaci et al. 1994). There are various ECG methods to detect LVH, which may be categorized as follows: (i) voltage criteria, including the product of QRS voltage and duration; (ii) point score systems; and (iii) the regression equation models (Hsieh et al. 2005) (Table 10.4). The ECG diagnosis of LVH is based mainly on the increase of the QRS voltage generated by the increase of left ventricular mass. The voltage criteria correlates better with ventricular wall thickness than with left ventricular mass, and the sensitivity of the ECG criteria declines with cavity dilation. As with many diagnostic techniques, specificity of these criteria is better than sensitivity. Table 10.4shows many of the voltage criteria with its sensitivity, specificity, and accuracy. We will now comment on the most used criteria. The best‐known QRS voltage criteria and voltage × duration products are as follows: Romhilt and Estes (1966) devised a score (Table 10.5) that attained a global specificity of 97% and a sensitivity of ≈55% in a post‐mortem series of mostly hypertensive and coronary patients. Compared with the voltage criteria, this system is somewhat more accurate (see Table 10.4). In this series, 58% of hypertrophic patients scored 5 points and 62% at least 4 points, while only 2 patients (3%) without hypertrophy scored 5 points. In patients with hypertension, sensitivity was 45% and in coronary patients it was 55%. When hypertension and coronary disease coexisted, sensitivity was 88%. Although an objective demonstration has yet to be carried out, the Romhilt–Estes point system would probably improve in sensitivity with little loss of specificity if the following additions or modifications would be performed: (i) ST–T vector opposite to QRS with digitalis = 2 points instead of 1; (ii) other repolarization alterations (flattened or negative T, straightened ST, negative U) = 1 point; and (iii) atrial flutter or fibrillation = 2 points. As mentioned above, however, in other group of populations that are based on standards of echocardiography or CMR for validating LVH, the sensitivity of this score is much lower while maintaining a very high specificity (≈100%). Table 10.5 Romhilt–Estes score. There is left ventricular enlargement if 5 or more points are obtained. Left ventricular enlargement is probable if the sum is 4 points. This is based on the presence of a strain pattern and at least one of the following voltage criteria: (i) R I + S III ≥ 2.5 mV; (ii) S V1/V2 + R V5/V6 ≥ 3.5 mV; (iii) the S wave in V1/V2/V3 > 2.5 mV + R wave in V4/V5/V6 ≥ 2.5 mm. This involves positivity in one of the following three criteria: (i) Cornell voltage: S V3 + R aVL > 24 mm (men) or 20 mm (women); (ii) left ventricular strain pattern; (iii) Romhilt–Estes score ≥ 5 (Schillaci et al. 1994). This shows similar results and confirms that the point score systems are the best criteria for assessing LVH (Morrison et al. 2007). Regression equations for estimating left ventricular mass were used to identify LVH. Table 10.4 shows the most common models. However, they are not used in clinical practice, although they may be implemented in automatic interpretation. The accuracy in detecting LVH and evaluating risk of cardiovascular death is similar to the best voltage or score systems (see Table 10.4). Having already commented on some of the limitations of the diagnostic criteria due to methodology used, type of patient cohort, etc., we will now expand on some of these aspects (Levy et al. 1990; Hsieh et al. 2005; Jain et al. 2010).
Chapter 10
Ventricular Enlargement
Concept: preliminary considerations
Critical review of the electrocardiographic concepts of systolic and diastolic overload
New concepts
Right ventricular enlargement: hypertrophy and dilation
Concept and associated diseases
Mechanisms of ECG changes
Changes of QRS–T in cases of progressive anterior and right QRS loop displacement (Figures 10.2 and 10.3)
Type I QRS loop (Figures 10.2 and 10.3A)
Type II, III, IV, or V QRS loop (Figure 10.2)
Other electrocardiographic features of the anterior QRS loop
A similar pattern in V1 may be seen in the evolutive process of RVE in different diseases (Figure 10.4)
Changes of QRS–T in cases of progressive posterior and right QRS loop (Figures 10.2, 10.3, 10.12, and 10.13)
Diagnostic ECG criteria: anatomic and imaging correlations (Tables 10.1 and 10.2)
Value of other electrocardiological techniques
Special characteristics of some types of right ventricular enlargement
Acute right ventricular dilation
Decompensated cor pulmonale
Criterion
Sensitivity (%)
Specificity (%)
V1
R/S V1 ≥ 1
6
98
R V1 ≥ 7 mm
2
99
qR in V1
5
99
S in V1 < 2 mm
6
98
IDT in V1 ≥ 0.35 sec
8
98
V5 − V6
R/S V5 − V6 ≥ 1
16
93
R V5 − V6 < 5 mm
13
87
S V5 − V6 > 7 mm
26
90
V1 + V6
R V1 + S V5 − V6 > 10.5 mm
18
94
ÂQRS
ÂQRS ≥ 110°
12–19
96
SI SII SIII
24
87
Sign
Points
1. Ratio reversal (R/S V5: R/S V1 ≤ 0.4)
5
2. qR in V1
5
3. R/S ratio in V1 > 1
4
4. S in V1 < 2 mm
4
5. R in V1 + S in V5 or V6 > 10.5 mm
4
6. Right axis deviation >110°
4
7. S in V5 or V6 ≥ 7 mm and each ≥2 mm
3
8. R/S in V5 or V6 ≤ 1
3
9. R in V1 ≥ 7 mm
3
10. S1, SII and SIII each ≥1 mm
2
11. SI and OIII each ≥1 mm
2
12. R′ in V1 earlier than 0.08 and ≥2 mm
2
13. R peak in V1 or V2 between 0.04 and 0.07
1
14. S in V5 or V6 ≥ 2 mm but <7 mm
1
15. Reduction in V lead R/S ratio between V1 and V4
1
16. R in V5 or V6 < 5 mm
1
Pulmonary embolism
Right ventricular enlargement in children
Indirect signs of right ventricular enlargement
Regression of ECG signs
Differential diagnosis (Table 10.3)
Differential diagnosis of right ventricular enlargement with dominant R or rSr′ morphology in V1
Clinical setting
R or RN(r′) morphology in V1
QRS width
P wave morphology in V1
1. No heart disease
<0.12 sec
P– in 2° IS P+ or ± In 4° IS
<0.12 sec
Normal
<0.12 sec
Final negativity
2. Classical RBBB
From <0.12 to >0.12 sec
Normal
3. Atypical RBBB
Usually ≥0.12 sec
Usually peaked and/or ±
May be 0.12 sec
Usually pathologic
Sometimes ≥0.12 sec
Normal
4. Right or biventricular enlargement (athletes) or even LVH (Hypertrophic or others CM)
<0.12 sec
Usually tall and peaked. Sometimes ±
5. Wolff–Parkinson–White syndrome
From <0.12 to >0.12 sec
Normal P wave with short PR
6. Lateral MI
<0.12 sec
Usually normal
7. Block of middle fibers (especially if the pattern is transient (see Chapter 11)?
Prominent R in V1 or more frequently V2
<0.12 sec
Usually normal
Differential diagnosis of right ventricular enlargement with rS or Qs morphology in V1
Differential diagnosis of right ventricular enlargement in the presence of ST/T changes
Diagnosis of RVE in the presence of ventricular blocks
Advanced RBBB
Advanced LBBB
Clinical implications
Left ventricular enlargement: hypertrophy and dilation
Concept and associated diseases
Mechanism of ECG changes
Changes in the QRS complex (Figures 10.19–10.25)
Changes in ST and T (Figures 10.19, 10.25, 10.26, and 10.27B)
Diagnostic ECG criteria: anatomic and imaging correlations (Tables 10.4 and 10.5)
Criteria
Sensitivity (%)
Specificity (%)
CV risk of death (adjusted hazard ratio and 95% CI) according to Hsieh et al. (2005)
(A) Voltage and duration of QRS
1) Sokolow and Lyon (1949)
21* 26**
89* 92.6**
1.9 (1.6–2.2)
2) Cornell voltage (Devereux et al. 1984)
16* 15.1**
97* 97.3**
3.1 (2.5–3.8)
3) Framingham adjusted Cornell voltage (Norman and Levy 1993)
17 (men)*
98*
1.4 (1.2–1.6)
22 (women)*
15.1**
97.2**
4) Cornell duration product (Mulloy et al. 1992; Okin et al. 1995)
50* 14.8**
96* 97.3**
2 (1.5–2.6)
5) 12 leads sum (Siegel and Roberts 1982)
6
91.7
2.6 (2.1–3.2)
6) 12 leads sum voltage and QRS duration (Siegel and Roberts 1982)
7.5
96.7
2.7 (2.3–3.1)
7) Talbot et al. (1976) (see text)
Low
High
—
(B) Scores systems
1) Romhilt and Estes ≥4 (1966)
18* 15.9**
99* 97**
2.9 (2.5–3.4)
2) Romhilt and Estes ≥5 (1966)
15* 5.7**
100* 99.1**
3.7 (3.0–4.4)
3) Framingham (Levy et al. 1990)
6.9* 7**
98.8* 99.2**
3.4 (2.4–4.9)
4) Perugia (Schillaci et al. 1994)
34* 24.7**
93* 93.2**
2.9 (2.5–3.3)
5) Glasgow R.I. (Morrison et al. 2007)
23.9*
95*
—
(C) Regression models
1) Rautaharju et al. LV mass index equation (1988)
38.8*
70*
2.6 (2–3)
2) Wolf et al. logistic regression (1991)
53.7 (men)*
94.9 (men)*
3.7 (2.7–5)
3) Casale et al. logistic regression (1987)
63.4 (women)*
92.9 (women)*
62 (necropsis)
92 (necropsis)
3.3 (2.8–3.9)
ECG criteria based on QRS voltage
Point score systems
Romhilt–Estes score
A. Criteria based on QRS modifications
1. Voltage criteria
3 points
One of the following should be present:
2. ÂQRS at −30° or more to the left
2 points
3. Intrinsicoid deflection in V5–V6 ≥ 0.05
1 point
4. QRS duration ≥0.09 sec
1 point
B. Criteria based on ST‐T changes
1. ST‐T vector opposite to QRS ( ) without digitalis
3 points
2. ST‐T vector opposite to QRS ( ) with digitalis
1 point
C. Criteria based on P wave abnormalities
1. Negative terminal P mode in V1 ≥ 1 mm in depth and 0.04 sec in duration
3 points
Framingham score (Levy et al. 1990)
The Perugia score
The Glasgow Royal Infirmary score
Regression models
Limitations of the diagnostic criteria
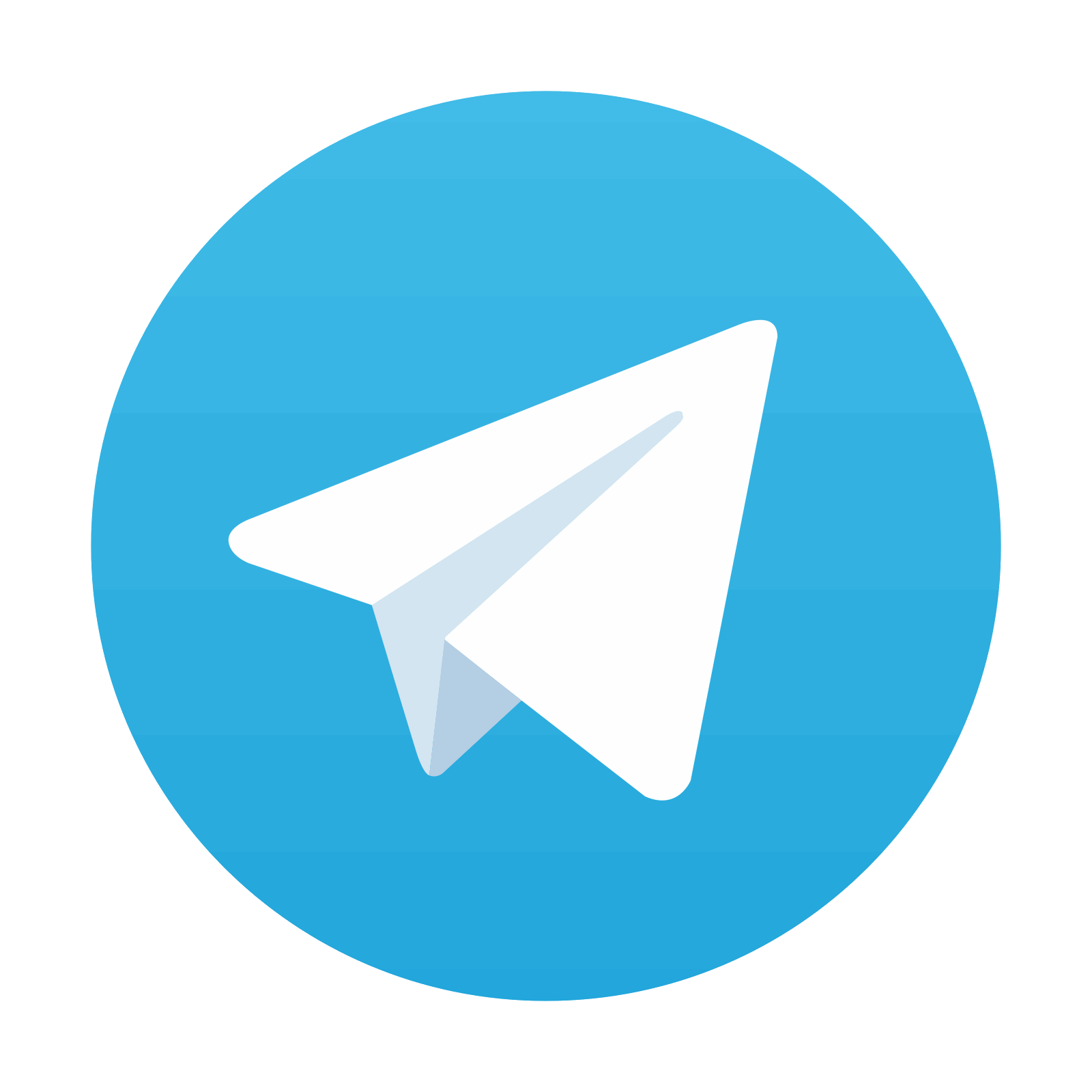
Stay updated, free articles. Join our Telegram channel

Full access? Get Clinical Tree
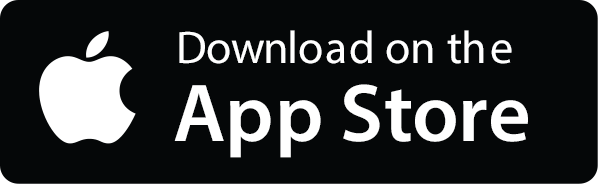
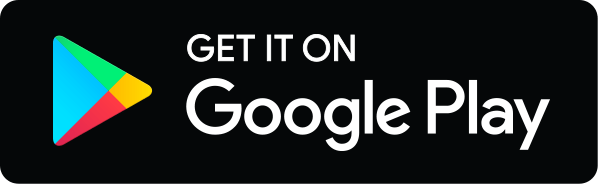