Venous shunts are surgical reconstructions involving an anastomosis between one or both venae cavae to one or both pulmonary arteries (PAs), and were developed to palliate infants born without two ventricular chambers. Staging venous shunts are typically performed during infancy and childhood, and include the older Glenn shunt, which anastomosed the superior vena cava to the left PA, and the more recent bidirectional cavopulmonary shunt, which anastomosed the superior vena cava to the PA, leaving both PAs in continuity. Subsequently a Fontan-type repair is performed to anastomose the inferior vena caval flow to the PAs, classically achieved by anastomosing the right atrium to the PAs. The Fontan repair achieves separation of the pulmonary and systemic circulations, resulting in a circulation without a subpulmonic ventricular pumping chamber.
The introduction of venous shunts to the management of patients with univentricular hearts has extended survival for patients with the most complex forms of congenital heart disease to greater than 75% by 25 years following surgery. In general, these procedures are applied to patients with “functionally univentricular physiology.” As first performed in 1968, the Fontan surgery channeled systemic venous return to the PAs, with the inclusion of inflow and outflow prosthetic valves. The Fontan palliation was initially applied to patients with tricuspid atresia and anatomic single left ventricles (LVs), whose mortality without surgery was more than 90% in the first year of life. The Fontan principle was extended gradually to more complex forms of functionally univentricular anatomy, including unbalanced biventricular anatomy and later to patients with single right ventricles (RVs) ( Fig. 12.1 ). In centers seeing adults with congenital heart disease, the Fontan population represents about 5% of patients. Due to the complexity of their cardiac anatomy, the insidious nature of disease progression, the high incidence of arrhythmias, and the challenges of assessing the Fontan “circulation” as opposed to traditional cardiac assessment of ventricular contractility and valve abnormalities, the patient with Fontan palliation poses unique and growing challenges to optimal care.

Surgical Techniques for Patients With Univentricular Physiology
To survive the neonatal period, infants with univentricular physiology require adequate pulmonary flow and protection from excessive pulmonary flow, adequate atrial-level mixing without restriction at the atrial septal level, and relief of aortic outflow obstruction when present. As a first stage of surgical interventions, slightly more than 80% of infants undergo surgery for pulmonary flow modification: augmentation of pulmonary flow with systemic-to-pulmonary shunts in 63% to 80% or restriction of pulmonary flow with PA banding in 12% to 25%. Surgical atrial septectomy to allow adequate atrial mixing without pulmonary venous hypertension was required in up to 14% of patients. Repair of the aortic arch was needed in 7% to 10% of patients ; subsequent application of Fontan repairs in the 1980s to patients with hypoplastic left heart syndrome required reconstructive surgery of the ascending aorta (Ao) in all of these patients (Damus-Kaye-Stansel or Norwood procedures).
Once pulmonary blood flow and atrial-level mixing are stabilized, the introduction of the classic Glenn shunt (superior vena cava to right PA) or the bidirectional cavopulmonary anastomosis has been used as the second stage of surgery prior to the Fontan repair, often with associated PA augmentation performed ( Fig. 12.2 ). In 1958, Glenn published his series of shunts from the superior vena cava to the right PA, whereby the right PA was divided and anastomosed to the right side of the superior vena cava after ligation and division of the azygos vein. The superior vena cava was then ligated at the cavoatrial junction. This operation quickly gained the eponym the Glenn shunt, and implies that the right and left PAs are not in continuity with each other. The early effects of the unidirectional Glenn shunt showed that it was a relatively simple operation, improved oxygen saturation, and provided excellent palliation for many patients. Unfortunately, late deterioration occurred because of decreased effective pulmonary blood flow, resulting from the development of systemic venous collateral vessels and pulmonary arteriovenous malformations. The increased venous pressure to the lungs caused systemic venous collateral vessels to develop, thereby shunting blood flow away from the PA. Pulmonary arteriovenous malformations were initially attributed to lack of pulsatile flow, but later found to result from the exclusion of hepatic venous flow from the pulmonary circulation.

The development in 1989 of anastomosis of the superior vena cava to the main PA without branch PA division took on the name bidirectional Glenn (or bidirectional cavopulmonary) shunt. The bidirectional Glenn shunt is performed by anastomosing the superior vena cava to the right branch of the PA using fine sutures and then dividing the proximal main PA, leaving the branch PAs in continuity. The introduction of cavopulmonary shunt surgery between neonatal surgery and the Fontan repair of childhood coincided with a marked improvement in early survival after the later Fontan surgery, by allowing stepwise diversion of systemic venous return from the upper body directly to the PAs. Subsequently, at the time of Fontan surgery, acute ventricular volume unloading (which results from the complete separation of pulmonary and systemic flows) is avoided, allowing ventricular function to adapt to the changed loading conditions. The bidirectional cavopulmonary anastomosis improves systemic arterial oxygen saturation without increasing pulmonary vascular resistance and maintains continuity of the PAs but can also lead to development of systemic venous collateral vessels and pulmonary arteriovenous malformations. For these reasons, cavopulmonary shunts are usually short-term, palliative procedures performed in young children (usually <2 years) who are being prepared for an eventual Fontan procedure. Simultaneously, the age at which Fontan completion surgery is performed has decreased substantially to limit the period of cyanosis and volume overload and is now generally performed before the age of 2 years, compared with ages 5 to 8 years, which was customary three decades ago.
The third stage of surgical intervention is the Fontan operation and its many modifications, one of which is the Kreutzer procedure ( Fig. 12.3 ). The Fontan repairs are characterized by complete separation of the pulmonary and systemic circulations, and depend on high systemic venous pressure and low PA pressure/resistance to propel nonpulsatile blood flow through the pulmonary circulation without the benefit of a pumping chamber. Fontan and Kreutzer published their findings within 2 years of each other and together proved that systemic venous pressure would be sufficient to propel blood flow through the pulmonary circulation in the absence of a subpulmonary ventricular pump as long as other hemodynamic considerations were optimal. It was Fontan’s thought that the right atrium, which is quite thickened in patients with tricuspid atresia ( Fig. 12.4 ), could be made to function as an RV; hence, the originally perceived necessity for inflow and outflow bioprosthetic valves. Kreutzer’s contribution was the direct atriopulmonary anastomosis, which eliminated the need for interposed venous valves, and resembles more closely the type of cavopulmonary connections that are encountered today.


Between 1970 and the early 1990s, the right atrium-to-PA direct connection (both retroaortic and anteroaortic) became standard therapy, as did the Björk modification in which the right atrial appendage is anastomosed to the right ventricular outflow tract or to the main PA ( Fig. 12.5 ). Due to the compliance and growth potential of atrial tissues, progressive right atrial dilatation, venous stasis and thrombosis, and atrial reentrant tachycardia developed in patients with atriopulmonary connections, especially those individuals with anteroaortic connections. The gradually enlarging right atrium created a size mismatch to the pulmonary anastomosis, with excessive “power loss” or turbulence of passive venous flow to the PAs, as well as compression of pulmonary venous return from the right lung (see Fig. 12.5 ). The desire to limit atrial distention and thus avoid obstruction to atrioventricular valve inflow led to the development of the total cavopulmonary lateral tunnel connection, which was demonstrated to have superior blood flow characteristics and allowed unimpeded pulmonary venous return to a right-sided atrioventricular valve. The increased suture load used in the right atrium to construct the lateral tunnel was not initially recognized as a future arrhythmogenic consequence of the procedure. Further surgical modifications were developed to allow application of the Fontan surgery to patients with hypoplastic LVs and to limit the development of atrial arrhythmias, (see Fig. 12.5 ).

The latest modification of the Fontan operation was the extracardiac total cavopulmonary connection, which was introduced by Marcelletti et al. in 1988. He and many colleagues showed that an extracardiac tube graft could link the inferior vena cava directly to the PA without the obligatory suture load within the right atrium. Given the relative technical ease of the extracardiac operation, often requiring no cross clamp and sometimes being performed without cardiopulmonary bypass, ideally the surgery would be associated with a decreased incidence of atrial arrhythmias and limit the potential for size mismatch between the enlarging right atrium and PAs. To achieve optimal flow dynamics, the anastomosis of the tube graft to the inferior aspect of the PA needs to be offset from the superior bidirectional Glenn anastomosis, avoiding collision of blood streams; reconstruction of the left PA is often needed. Attention to each of these technical details is crucial to the long-term flow dynamics. The material that was used for the extracardiac connection has changed over time: aortic homografts were initially used but were prone to calcification and induced preformed antibodies, a concern for a population that would potentially require later heart transplantation. As a result, the 16- to 20-mm polytetrafluoroethylene (Gore-Tex) tube became the graft of choice for initial extracardiac connections, which is not prone to calcification. The extracardiac connection has the advantage of improved flow dynamics, but does not have growth potential commensurate with body growth, and is noncompliant. As the body surface area of the patient increases and flow increases, the extracardiac connection becomes a potential source of increased pathway resistance and hemodynamic inefficiency, which has been demonstrated by magnetic resonance imaging (MRI) studies. Due to the restrictive size of the graft, the ensuing decrease in ventricular filling and preload may adversely affect ventricular performance. In this scenario, one can expect to see an increased incidence of ascites and protein-losing enteropathy (PLE) at a younger age compared with older atriopulmonary Fontan patients, presumably with a decreased incidence of atrial reentry tachycardia.
Fontan Surgical Sequelae
Systemic venous pathway obstruction can result from stenotic atriopulmonary connections; lateral tunnel or extracardiac graft stenosis, calcification, and size restriction; superior vena cava stenosis; and peripheral PA stenosis. Any obstruction to the passive venous flow to the lungs leads to hepatic congestion, atrial enlargement, and fibrosis with thrombus formation ( Fig. 12.6 ); decreased pulmonary flow; and decreased cardiac output. In particular, atriopulmonary obstructions can be subtle, often showing only 2- to 3-mm Hg gradients by catheterization, which are nonetheless quite important hemodynamically due to the requirement of passive venous flow. Although these stenotic lesions can occur in any Fontan patient, they are more likely to develop in patients with certain types of anastomoses: (1) patients with a Glenn shunt to the right PA and atriopulmonary anastomosis to only the left PA, (2) an anteroaortic connection from the right atrium to the PA, (3) a valved or nonvalved conduit from the right atrium to the RV or PA, and (4) an aortic homograft extracardiac anastomosis. Pulmonary venous obstruction in Fontan patients usually occurs as a consequence of severe right atrial dilation causing compression of the right pulmonary veins ( Fig. 12.7 ), or marked coronary sinus dilation causing left pulmonary vein obstruction. Left ventricular outflow tract obstruction occurs most commonly in patients with (1) a double-inlet LV and transposition of the great arteries with a closing bulbo-ventricular foramen producing subaortic stenosis, (2) staged correction of hypoplastic left heart syndrome who develop recurrent coarctation or increased aortic stiffness from the use of prosthetic or homograft material, and (3) anastomotic problems from the various forms of Damus-Kaye-Stansel operations causing supra-aortic stenosis. Associated lesions that negatively impact the Fontan circulation include aortic aneurysm, residual atrial and ventricular shunts, discontinuous PAs, and the development of venovenous collaterals to the left atrium (LA).


Fontan Revision
Fontan revision refers to a surgical intracardiac intervention in a Fontan patient, such as subaortic resection, valve repair, or enlargement of PAs, leaving the same form of atriopulmonary connection in place. In the dilated single ventricle with declining systolic function, atrioventricular valve annular dilatation and regurgitation may be present. By raising left atrial pressure and pulmonary venous pressure, moderate or greater atrioventricular valve regurgitation results in further decline of cardiac output; valve repair poses the risk of worsening ventricular function by removing the afterload reduction provided by valvar regurgitation. The incidence of significant regurgitation is highest with common atrioventricular valves, followed by tricuspid valves ; mitral valve repairs in older Fontan patients show inconsistent results and may require prosthetic valve replacement.
Fontan Conversion Surgery
Technically, “Fontan conversion” refers to the replacement of an atriopulmonary anastomosis with an extracardiac total cavopulmonary connection, usually in association with arrhythmia surgery. Fontan conversion operative technique consists of three components: takedown of the existing atriopulmonary communication and repair of associated hemodynamic lesions, arrhythmia surgery, and epicardial pacemaker implantation. The first stage is challenged by the extensive chest adhesions from multiple prior sternotomies and avoidance of unwanted atrial or aortic entry during sternotomy. The enlarged right atrial anterolateral wall is widely resected, followed by takedown of the existing atriopulmonary connection. An extracardiac polytetrafluoroethylene (Gore-Tex) tube graft (usually 24 mm in diameter) replaces the atriopulmonary connection, anastomosed inferiorly to the inferior vena cava and superiorly to the underside of the PA. The atrial septum is widely resected to form a single atrium to receive pulmonary venous inflow. Additional right and/or left pulmonary arterioplasty may be necessary, or pulmonary reconnection in cases with a right Glenn shunt and an atrio left PA connection. The coronary sinus may require unroofing in patients with left pulmonary vein compression from massive coronary sinus dilatation.
Right atrial macro-reentry tachycardia is predominantly present and is addressed using a modified right-sided maze procedure ( Fig. 12.8 ). In some patients, the atrial reentry tachycardia is present in the LA, and increasing numbers of adult Fontan patients develop atrial fibrillation in addition to right atrial tachycardia. In the presence of atrial fibrillation or left atrial reentry tachycardia, or in patients with significant left-sided atrioventricular valve regurgitation, the left atrial Cox-maze IV procedure is performed in addition to the modified right atrial maze (see Fig. 12.6 ). When identified preoperatively, additional arrhythmia surgery for atrioventricular nodal reentry tachycardia, accessory connections, or ventricular aneurysm producing ventricular tachycardia may be needed. Implantation of an epicardial dual-chamber antitachycardia pacing system is performed to achieve atrial pacing with intact atrioventricular conduction and avoid ventricular pacing. Insertion of ventricular leads has been performed to enable atrial tachycardia detection algorithms, and to avoid reoperation in the setting of later development of atrioventricular block; multisite ventricular leads (resynchronization) or epicardial ventricular defibrillator leads may also be required ( Fig. 12.9 ).


The survival to adulthood of patients with single-ventricle physiology and an inexorable decline in circulatory dynamics has resulted in an increased population referred for cardiac transplantation, which some consider to be the fourth stage of Fontan surgery. Among adults undergoing heart transplantation, only 2% have congenital heart disease, of whom 36% to 44% carry a diagnosis of single ventricle, indicating the magnitude of the challenge for long-term care of these patients.
Fontan Cardiac Physiology
The systemic venous circulation in the Fontan circulation is comprised of three distinct channels: superior vena caval flow, inferior vena caval flow, and splanchnic flow. Elevated pressures in the superior vena cava impairs lymphatic resorption, which may contribute to increased pulmonary vascular resistance, development of collateral flow, and uncommonly, plastic bronchitis (fibrinous rubber casts in the tracheobronchial tree producing cough and wheezing). Elevated pressure in the inferior vena cava results in chronic hepatic congestion. Splanchnic flow channels venous blood from the intestine and spleen to the portal vein, and has venous pressure that is up to three times higher than that present in the inferior vena caval flow draining the kidneys, pelvis, and lower extremities. The elevated splanchnic pressure results in lymphatic hypertension and the loss of protein including immunoglobulins via the intestines, which may result in PLE presenting as ascites with hypoalbuminemia.
In a “Fontan circulation” the systemic venous return is connected to the PAs without a prepulmonary pump ( Fig. 12.10 ). The residual postcapillary energy is not allowed to run off to the systemic venous atrium, but is used to push blood through the lungs. Advantages of a Fontan circulation on single-ventricle physiology include near-normalization of the arterial oxygen saturation and abolishment of the chronic volume overload on the single ventricle. However, because pulmonary impedance hampers venous return through the pulmonary vasculature, this connection creates, like any dam, upstream congestion and downstream decreased flow. These two features of the Fontan circulation, upstream venous congestion and downstream decreased output, are the basic cause of the majority of the physiologic impairments of this circulation. De Leval has termed this state the “paradox of the Fontan circulation”: the imposition of caval hypertension and pulmonary arterial hypotension as conditions of success.

Flow through the Fontan circulation will depend on the resistance of a series of locations: the surgical connection, central and peripheral PAs, pulmonary vascular resistance (precapillary sphincters, pulmonary capillaries, and veins), and the pressure gradient across the bottleneck (systemic venous pressure–ventricular filling pressure). The Glenn and Fontan connections themselves create abnormal pulmonary flow conditions: flow differential to the branch PAs, mild desaturation, increased collateral flow, suboptimal mixing of inferior and superior caval flow, and resultant endothelial dysfunction. Any increase in the pulmonary venous atrial pressure, such as from arrhythmia, atrioventricular valve regurgitation, or elevated end-diastolic pressure, will further decrease transpulmonary flow, resulting in a continuously declining cardiac output. The body tolerates only a small range of increased pressures in the systemic veins (between 12 and 20 mm Hg) and a small range of ventricular filling pressures; this leaves the impedance of the neoportal system as the major determinant of output.
The single ventricle has endured variable years of intense cyanosis and hypertrophy from volume overload prior to Fontan surgery and has developed increased mass and fibrosis, which may be ongoing in the setting of aortic stiffness or obstruction. The ventricle, which is the typical bottleneck in a biventricular circulation, no longer controls cardiac output and cannot decrease the degree of systemic congestion. However, the single ventricle can make the circulation worse: any increase in filling pressure will result in more systemic venous congestion and less cardiac output.
Ventricular systolic function has been shown to remain relatively stable in adulthood in the single-ventricle population, in the absence of the development of atrial tachycardia or significant atrioventricular valve pathology. However, ventricular diastolic dysfunction progresses with age, with gradual increase in filling pressures. Hypertension or ventricular outflow tract obstruction results in increased ventricular afterload, ventricular hypertrophy, decreased compliance, and ventricular hypertension. Decreased ventricular compliance is associated with increased end-diastolic pressure and diastolic dysfunction, which have a negative back-pressure effect on the Fontan dam, leading to the cascade of progressive Fontan circulatory dysfunction. Obesity contributes significantly to decreased pulmonary compliance as well as increased systemic resistance and ventricular hypertrophy, and is directly detrimental to Fontan hemodynamics. Finally, the gradual increase in pulmonary resistance with normal aging contributes to compromised Fontan circulation with age. To mitigate these competing negative circulatory interactions, the future mechanical support of Fontan patients would lower caval pressure and produce increased pulmonary arterial pressure with pulsatile flow. In the meantime, the clinician is challenged to monitor the potential effects of this circulation and improve flow dynamics as feasible, with particular attention to each component of the circuit.
Clinical Status and Monitoring of the Adult Fontan Patient
The management of adult Fontan patients has, as its goal, the optimization of the circulation to prolong the satisfactory longevity of the unique Fontan physiology. Early anatomic and surgical characteristics, such as ventricular morphology , heterotaxy , prior PA or aortic arch reconstruction , older age at primary Fontan , atrioventricular valve regurgitation or repair , and prolonged postoperative pleural effusions are important predictors of late Fontan adverse outcomes, but obviously cannot be modified for the adult. A stepwise approach to the assessment of the adult Fontan patient is needed, both for optimization of hemodynamic status and for delineation of causes of so-called “failing Fontan” circulation.
To understand the anticipated challenges of the Fontan patient, it is important to understand the many anatomic and surgical variables of the individual patient, as well as the changes in physiology with age ( Table 12.1 ). Among current adults with Fontan circulation, approximately 50% to 75% have a single LV, 30% to 45% have a single RV, and biventricular complex anatomy including heterotaxy syndrome affects up to 15% of patients. The most common forms of Fontan surgeries encountered in current adults are atriopulmonary anastomoses in 20% to 60% of patients, lateral tunnel repairs in 25% to 45% of patients, and extracardiac total cavopulmonary connections in 11% to 20% of patients. The age of the adult patient is an indicator of the more likely form of prior Fontan surgery because the atriopulmonary anastomosis was performed between 1968 and 1995, the lateral tunnel repair was introduced in 1988, and the extracardiac conduit became widely used in the mid-1990s. See Table 12.2 for outcomes reported with adult Fontan populations.
Variable | Anatomy, Surgery, Age | Incidence(%) | Considerations |
---|---|---|---|
Single left ventricle | 50-75 | Ventricular dilatation, overall improved systolic function compared with single right ventricle | |
Tricuspid atresia | 22-40 | — | |
Double inlet left ventricle | 18-26 | Subaortic outflow obstruction | |
Pulmonary atresia; hypoplastic right heart | 3-8 | — | |
Single right ventricle | 30-45 | Increased incidence of systolic ventricular dysfunction, AV valve regurgitation | |
Double outlet right ventricle | 15 | — | |
Hypoplastic left heart, mitral atresia | 2-12 | Risk factor for long-term survival | |
Biventricular morphology | 5-15 | — | |
Unbalanced AV septal defect | 10-14 | — | |
Heterotaxy syndrome | 4-15 | Atrial isomerism: increased incidence of SVT; decreased survival | |
cc-TGA, TGA with straddling tricuspid valve | — | Presence of two ventricular pumping chambers may improve cardiac output; ccTGA increased risk of complete AV block | |
Staging Procedures | |||
Systemic-to-pulmonary shunts | 63-82 | Potential distortion of branch pulmonary arteries | |
Pulmonary artery banding | 11-26 | — | |
Pulmonary artery reconstruction | 11-15 | Risk for long-term pulmonary artery distortion/obstruction | |
Aortic arch repair, excluding HLHS | 7-22 | Imposition of prosthetic patch on aorta or residual narrowing may increase ventricular afterload | |
Classic Glenn to LPA | — | Pulmonary arteriovenous malformations, cyanosis | |
Bidirectional cavopulmonary anastomosis | 15-80 | Improved long-term outcomes? | |
Age at Fontan repair | 4-7 years among current adults | — | Age >7 years may be risk factor for survival |
Complication | Incidence(%) | Considerations |
---|---|---|
Reoperation | 1-18 | Anastomotic obstruction, pulmonary artery distortion, subaortic obstruction, atrioventricular valve regurgitation, aortic arch obstruction; pacemaker implantation |
Catheter interventions | 6-30 | Fenestration closure; conduit or pulmonary artery stents; coil occlusion of collaterals; ablation |
Cyanosis | Progressive | Right-to-left shunting: intrapulmonary- or atrial-level/fenestration; venovenous collaterals to pulmonary veins; coronary sinus drainage to left atrium; hepatopulmonary syndrome |
Protein losing enteropathy | 2-9 | Endothelial protein-losing disorder: Hypoalbuminemia, ascites, elevated fecal alpha 1 antitrypsin; increased susceptibility to proinflammatory cytokines Elevated splanchnic pressure; decreased cardiac output |
Plastic bronchitis | 1-3 | Lymphatic hypertension; decreased lymphatic resorption |
Thromboembolism Stroke Pulmonary embolus Renal infarct | 5-10 1.5-6 1-4 <1 | Procoagulant state: abnormalities of protein C, protein S, antithrombin III; increased platelet reactivity; venous stasis, atrial thrombosis |
Anemia | 15-48 | Low iron stores, associated with diuretic, warfarin usage |
Thrombocytopenia | 30-36 | Splenic sequestration |
Endocarditis | 2 | Uncommon; sepsis reported as cause of death in 3%-18%, related to intestinal immunoglobin loss |
Liver disease | Late liver failure <10 | Common findings: hepatomegaly, mild elevation of bilirubin and gamma glutamyl transferase Increased risk of hepatocellular carcinoma; requires surveillance with imaging and alpha fetoprotein levels |
Sinus bradycardia | >70 | Almost uniformly present; junctional rhythm and escape-capture bigeminy frequently noted; chronotropic incompetence with exercise |
SVT | 10-70 | Increases with time, increased among AP/LT repairs Atrial reentry/flutter 75%, atrial fibrillation 40%, focal 10%-15% |
VT | 3-12 | Nonsustained VT noted with Holter or pacemaker monitoring |
Pacemakers | 9-23 | Sinus node dysfunction common; atrioventricular block more common in double inlet left ventricle or L-looped ventricle Atrial pacing preferred to single chamber ventricular pacing Transvenous approach limited, associated with atrial lead thrombosis; epicardial implantation usually required. |
Defibrillators | 2 | Sudden death considerations: arrhythmia, stroke, aneurysm rupture |
Fontan conversion surgery | 1-37 | Atriopulmonary Fontan patients; extracardiac repairs using aortic homografts |
Cardiac transplantation | 1-4 | Indications: Intractable arrhythmias, progressive exercise intolerance, cyanosis, protein-losing enteropathy, plastic bronchitis. Increased early mortality compared with other forms of congenital heart disease. |
Sudden death | 9-19 | Potential causes: arrhythmia, pulmonary embolus, stroke, vessel rupture |
Physical Findings
See Table 12.3 . In general, Fontan patients are slightly shorter than average adult height, with similar prevalence of overweight and obesity. Recent data suggest increased morbidity and mortality in Fontan patients with elevated body mass index (BMI), likely related to decreased pulmonary compliance, ventricular hypertrophy, diastolic dysfunction, and elevated systemic vascular resistance associated with obesity. Many older Fontan patients have progressive cyanosis, which may be more pronounced with exertion. Central cyanosis may be due to atrial-level fenestrations, intrapulmonary shunting (arteriovenous pulmonary malformations, ventilation-perfusion mismatch), or venovenous collaterals often to the LA, which develop as “pop-offs” due to elevated central venous pressure. Hepatomegaly is generally present, frequently with splenomegaly. Abdominal fullness or ascites may be present. Lower extremity venous insufficiency is present in as many as 60% of Fontan adults, manifests as discoloration, brawny induration, or significant varicosities, and may be related to prior catheterizations and deep venous thrombosis. The findings of obesity, resting desaturation, ascites, or advanced lower extremity venous changes are of significant concern and should prompt efforts to improve cardiovascular status.
Body habitus | Short stature Thin extremities | Overweight: similar to adult population Musculoskeletal wasting of arms: advanced cachexia |
Head | Facial plethora Jugular venous distention marked in supine position | Resting oxygen saturations usually >94% |
Chest | Sternal concavity; sternotomy scars | Restrictive lung physiology |
Cardiac Ventricular impulse First and second heart sounds | Bradycardia or premature beats Increased Single first and second heart sounds common | Presence of a murmur is abnormal and suggests AV valve insufficiency, outflow tract obstruction, aortic or pulmonic insufficiency |
Abdomen | Hepatomegaly typically present Central adiposity Ascites | Lack of hepatomegaly may indicate advanced liver disease/atrophy |
Extremities | Mild clubbing common Lower legs: Venous stasis/brawny discoloration/varicosities | Leg edema is an advanced finding of heart failure Advanced changes associated with poor outcomes |
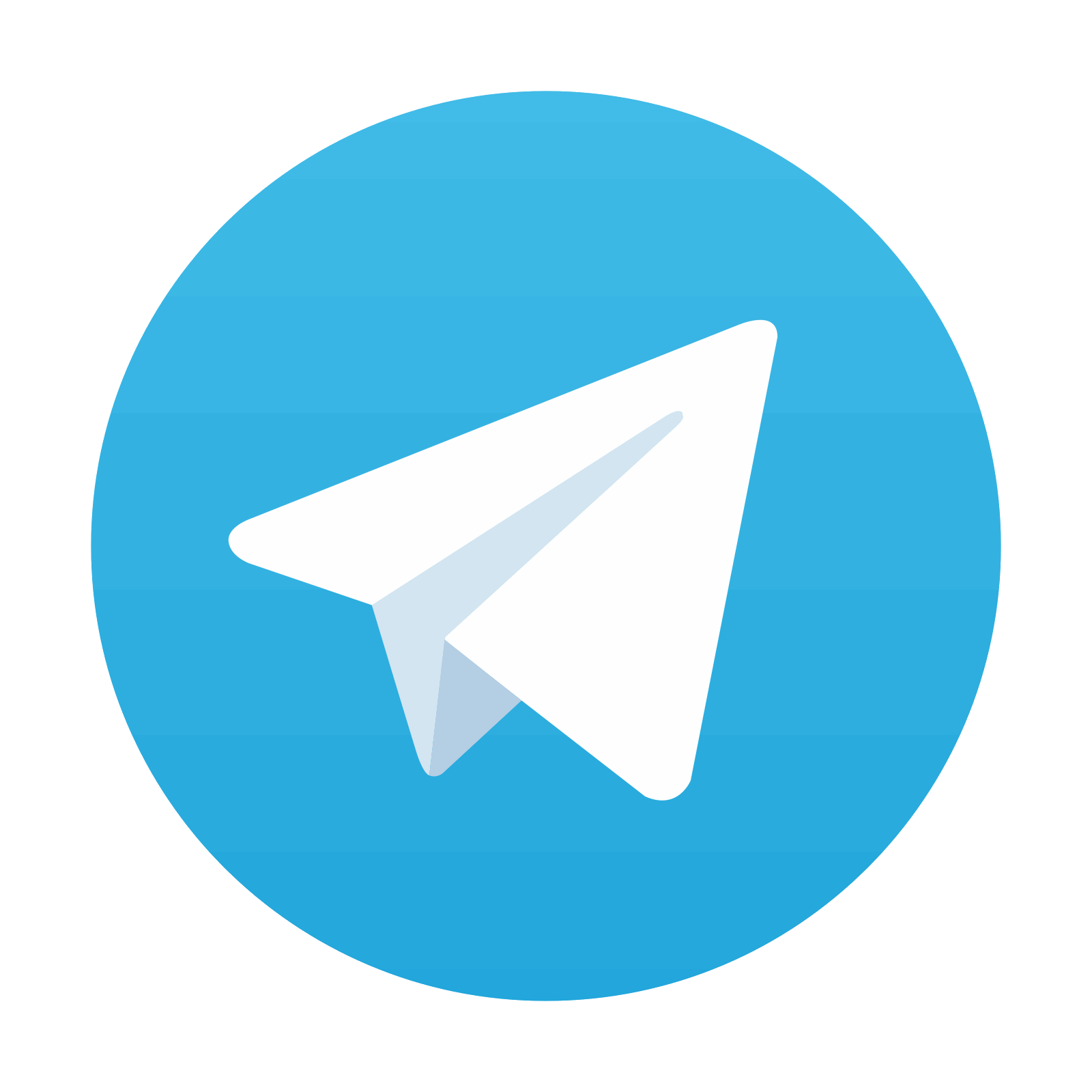
Stay updated, free articles. Join our Telegram channel

Full access? Get Clinical Tree
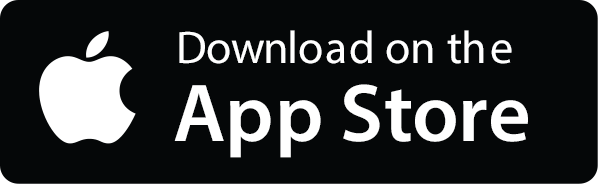
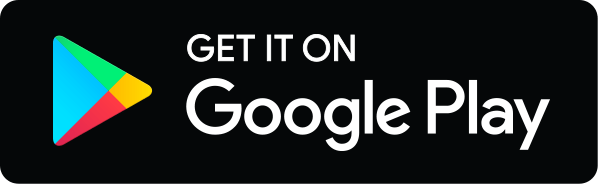
