Vasodilators
Uri Elkayam
Fahed Bitar
Sarkis Kiramijyian
Parta Hatamizadeh
Philip F. Binkley
Carl V. Leier
The introduction of vasodilator therapy as a major intervention for heart failure occurred when Gould et al. (1) reported in 1969 that the α-adrenergic blocker phentolamine improved hemodynamics and the clinical status of patients with heart failure. Nitroprusside was later noted to augment hemodynamics and improve the clinical condition of patients with heart failure after myocardial infarction (2). The wide recognition of vasodilator therapy as a major intervention for congestive heart failure (CHF) was greatly affected by the introduction of the flow-directed pulmonary artery catheter (3). The report of the Veterans Heart Failure Trial (V-HeFT) in 1986 (4) showed the potential of chronic vasodilation therapy in improving long-term outcome, and recently the African-American Heart Failure Trial (A-HeFT) study (5) demonstrated a powerful effect of vasodilators given in addition to other heart failure therapies in African-Americans with chronic symptomatic CHF. This chapter reviews the principles of administration and the role of vasodilators in the treatment of heart failure.
Rationale for the use of Vasodilators in Congestive Heart Failure
Reduction of Afterload
Ventricular afterload (ventricular wall stress during systole) is raised in most patients with heart failure because of an increase in one or more of its determinants, including ventricular systolic volume, aortic impedance, and systemic vascular resistance. Excessive afterload has an unfavorable effect on ventricular function in heart failure (Fig. 27-1A); the magnitude of this effect is proportional to the degree of ventricular failure (6). Vasodilator therapy is therefore directed at reducing the excessive ventricular afterload or wall stress of the failing heart, which results in augmentation of ventricular systolic function, stroke volume, cardiac output, and overall cardiovascular performance (Fig. 27-1B).
Reduction of Preload
Ventricular preload (cardiomyocyte stretch at end-diastole) increases in most forms of CHF secondary to an expansion of intravascular (and therefore intracardiac) volume via fluid retention by the kidney, neurogenic and hormonal venoconstriction, and elevated afterload. An increase in preload or end-diastolic actin-myosin stretch is generally accompanied by an augmentation of ventricular systolic function (Fig. 27-2A), a phenomenon commonly referred to as the Frank-Starling relationship (7). In patients with severe decompensated ventricular dysfunction, the rise in preload does not elicit a sufficient augmentation of ventricular systolic function because of a flat systolic function-preload curve. For this reason, any increase in ventricular end-diastolic pressure or volume (i.e., preload) is accompanied by only modest augmentation of systolic function and stroke volume (Fig. 27-2B). The persistent depression of stroke work and volume and cardiac output perpetuates the mechanisms responsible for fluid retention, venoconstriction, and excessive afterload, such that preload increases further to high levels (Fig. 27-2C) with accompanying signs and symptoms of congestion.
Combined Reduction of Afterload and Preload
It shall be noted that although vasodilator drugs are traditionally separated into those that achieve mostly afterload reduction and those that are directed mostly at preload reduction, all agents with predominant afterload-reducing properties also reduce preload via a number of mechanisms, including enhancement of ventricular systolic emptying and, in many patients, reduction of valvular regurgitation. Conversely, the predominantly preload-reducing drugs can favorably affect afterload by reducing ventricular volume.
Nitroprusside
Nitroprusside is the sodium (or potassium) salt of a complex molecule made up of ferric cyanide (Fe2+ and five cyanide groups) and nitric acid. Its effect appears to be mediated largely by production of nitrosothiol in vasculature, which in turn generates cyclic guanosine monophosphate (cGMP) in vascular smooth muscle and evokes relaxation (8).
Nitroprusside has a rapid onset of action, with vasodilating effects detectable within 60 to 90 seconds of initiation of the infusion. Some of the administered nitroprusside decomposes after entering the bloodstream, with the release of cyanide into the circulation (10). Additional cyanide is produced when nitroprusside is metabolized by vascular tissue, which then is further metabolized by the liver to thiocyanate and is slowly cleared by the kidneys (about a 3- to 4-day half-life). Because of its long half-life, thiocyanate can accumulate during prolonged or high-dose nitroprusside infusions or in the setting of renal dysfunction, and can evoke signs and symptoms of thiocyanate toxicity.
Hemodynamic Effects
Nitroprusside reduces the excessively elevated ventricular filling pressures in patients with CHF by multiple mechanisms. The drug diminishes heightened venous tone and thereby increases venous capacitance, with a resultant peripheral shift of central blood volume. By reducing the afterload of both left and right ventricles, nitroprusside decreases ventricular systolic and diastolic volume via greater ventricular systolic emptying and less valvular regurgitation (3,9,10,11,12). Nitroprusside may also lower ventricular filling pressure by improving ventricular diastolic properties or negating the restraining effect of the pericardium (by lowering intracardiac pressures and volume) (13,14). Improved renal blood flow may lead to increased diuresis and this may further contribute to preload reduction mediated by nitroprusside (15).
The use of nitroprusside in patients with decompensated heart failure results in a significant fall in systemic blood pressure, right atrial pressure, pulmonary arterial pressure, pulmonary capillary wedge pressure (PCWP), and systemic and pulmonary vascular resistance (Fig. 27-3). These changes are associated with a significant increase in cardiac output and no change in heart rate (9,11).
In the setting of CHF, nitroprusside generally lowers myocardial oxygen consumption as it reduces systolic and diastolic wall stress (16,17). The effect of nitroprusside on coronary hemodynamics has not been extensively studied in human heart failure. The net effect of nitroprusside on coronary blood flow in patients with significant coronary obstructive disease, however, may be determined by multiple factors, including effect on myocardial oxygen demand, coronary vasodilatory effect, and effect on perfusion pressure as well as diastolic filling time (17,18,19). In addition, nitroprusside may induce a coronary steal phenomenon by producing vasodilation of nonobstructed coronary beds, thus directing blood flow away from the already maximally vasodilated beds downstream from the coronary artery lesions (20,21).
In the setting of human CHF, nitroprusside preferentially reduces limb vascular resistance, with an augmentation of limb blood flow comparable in degree to the rise in cardiac output (22). Renal vascular resistance is also reduced in the therapeutic range of nitroprusside; however, the resultant renal blood flow and renal function are heavily dependent on changes in systemic blood pressure and renal perfusion pressure (15,22). Hepatic-splanchnic vascular resistance and blood flow are not significantly altered during infusion of nitroprusside at therapeutic rates (22).
Neurohormonal Responses
The neurohormonal response to nitroprusside infusion in heart failure varies considerably. Olivari et al. (23) found
disparate responses of norepinephrine (NE) in a population of patients with CHF despite similar resting hemodynamics and comparable hemodynamic responses. One group (Group I) experienced an increase in plasma NE and the other group (Group II) experienced a decrease during the infusion. Group II patients appeared to be in a more advanced and severe stage of heart failure, with a higher mortality. Differences in baroreceptor and mechanoreceptor sensitivity and responsiveness have been suggested as a cause for disparate responses to NE in the two groups. A later study by Johnson et al. (24) examined the effect of diuretics and nitroprusside in 34 patients with decompensated heart failure. This therapy resulted in a significant increase in aldosterone levels and plasma renin activity. There was no significant change in NE levels, while endothelin and plasma atrial natriuretic levels showed a significant fall.
disparate responses of norepinephrine (NE) in a population of patients with CHF despite similar resting hemodynamics and comparable hemodynamic responses. One group (Group I) experienced an increase in plasma NE and the other group (Group II) experienced a decrease during the infusion. Group II patients appeared to be in a more advanced and severe stage of heart failure, with a higher mortality. Differences in baroreceptor and mechanoreceptor sensitivity and responsiveness have been suggested as a cause for disparate responses to NE in the two groups. A later study by Johnson et al. (24) examined the effect of diuretics and nitroprusside in 34 patients with decompensated heart failure. This therapy resulted in a significant increase in aldosterone levels and plasma renin activity. There was no significant change in NE levels, while endothelin and plasma atrial natriuretic levels showed a significant fall.
Clinical Application and Administration
Indications
Nitroprusside is most commonly employed in the treatment of acute decompensated heart failure (9,11,24,25), complicated acute myocardial infarction (MI) (26), and acute valvular insufficiency (27). It is also frequently encountered in patients who have undergone cardiopulmonary bypass or other cardiac surgery and it has been shown to be effective for chronic infusion in patients with end-stage CHF awaiting heart transplantation (28). Nitroprusside is also employed in the evaluation of potential recipients of heart transplantation to determine the reversibility of elevated pulmonary vascular resistance (29). A recent report demonstrated a significant improvement of functional class of patients with end-stage heart failure awaiting heart transplantation with chronic infusion of nitroprusside, which was safer and more effective than dobutmaine in relieving symptoms, facilitating unloading therapy, and improving survival (28). Khot et al. (30) examined the effect of nitroprusside in 25 patients with severe aortic stenosis and left ventricular (LV) dysfunction. This therapy, which had been previously considered contraindicated for this patient population, resulted
in a significant increase in cardiac output. Based on these results, it was suggested by the authors that nitroprusside may provide a safe and effective bridge to aortic valve replacement in patients with aortic stenosis presenting with severe CHF.
in a significant increase in cardiac output. Based on these results, it was suggested by the authors that nitroprusside may provide a safe and effective bridge to aortic valve replacement in patients with aortic stenosis presenting with severe CHF.
Nitroprusside can effectively augment the hemodynamic effects of other drugs such as dopamine, dobutamine, and similar agents (31,32). Combination pharmacologic support is occasionally employed in the intensive care setting to optimize hemodynamic and clinical responses in patients with severely depressed cardiac output and elevated ventricular filling pressures.
Administration
Nitroprusside is administered intravenously with an infusion pump or microdrip regulator system to ensure controlled, precise dosing. Because of its light-sensitivity, the infusion set should be shielded. In CHF, the initial dose is 0.10 to 0.20 μg/kg per minute; this is gradually advanced as needed to attain the clinical and hemodynamic objectives. The incidence of side effects and toxicity is directly related to the dose and duration of administration. Because of its potent dose-related hemodynamic effect and the difficulties in determining maximal effective dose, nitroprusside is optimally administered with hemodynamic monitoring consisting of pulmonary artery catheterization and a close monitoring of systemic blood pressure.
Potential Adverse Effects and Toxicity
The most commonly encountered adverse effect of nitroprusside administration is systemic hypotension (23,33). When accompanied by a fall in coronary perfusion pressure and a rise in heart rate, nitroprusside-induced hypotension can be detrimental in patients with myocardial ischemia and infarction (20). A worsening renal function has been noted in association with nitroprusside infusions, typically during periods of systemic hypotension or hypoperfusion. Some patients may experience hemodynamic rebound with symptomatic deterioration after the abrupt discontinuation of nitroprusside (34). Gradual discontinuation is therefore recommended in order to achieve a smoother withdrawal.
Nausea, disorientation, confusion, psychosis, weakness, muscle spasm, hyperreflexia, and convulsions are side effects of thiocyanate toxicity, which may occur as plasma thiocyanate concentrations rise above 6 mg (33,35). The early sign of cyanide toxicity is metabolic (lactic) acidosis. Thiocyanate can be removed with hemodialysis and cyanide toxicity has been successfully managed with infusions of thiosulfate, sodium nitrate, and hydroxycobalamin. Conversion of cyanide to prussic acid raises methemoglobin levels and thus lowers the oxygen-carrying capacity of the blood. Thiocyanate and cyanide toxicity are rare during the usual administration of nitroprusside in heart failure (≤3 μg/kg per minute for ≤72 hours).
Nitroprusside can lower systemic arterial oxygen content by causing or exacerbating a pulmonary ventilation-perfusion mismatch, probably via dilatation of pulmonary arterioles in nonventilated areas (36). However, for most patients receiving nitroprusside, oxygen delivery is still augmented during the infusion because of the rise in cardiac output.
Laboratory data suggest that nitroprusside is capable of diverting blood flow from ischemic or threatened myocardium to normal myocardium by dilating the arterioles in the normal region (20,21). This potential for so-called coronary steal suggests a preference of nitroglycerin (NTG) over nitroprusside in patients with occlusive coronary artery disease. Other, far less commonly encountered side effects of nitroprusside include reduced platelet number and function, hypothyroidism (thiocyanate impairs iodine transport), and vitamin B12 deficiency (37,38).
Organic Nitrates
Nitrates have been used for more than 100 years in clinical medicine, predominantly in the treatment of angina pectoris. Although not officially approved by the U.S. Food and Drug Administration (FDA) for use in CHF, nitrates have earned a role in the therapeutics of this condition during the past two decades (39,40).
Mechanism of Effect
Organic nitrates are prodrugs that undergo a complex metabolic biotransformation predominantly in the smooth muscle intracellular space (41). This biotransformation leads to the formation of nitric oxide (NO) or a related S-nitrosothiol, which stimulates the enzyme guanylate cyclase and leads to the formation of cyclic guanosine monophosphate (cGMP) in the vascular wall. GMP reduces intracellular calcium levels by decreasing its release from the cytoplasmic reticulum and by reducing its influx from the extracellular space. The decrease in intracellular calcium leads to a venous and arterial vasodilatation, which is the main cardiovascular effect of these drugs. Endothelial production and release of prostacylin may also contribute (42). Nitrates are cleared by extraction in the vasculature, hydrolysis in blood, and the action of glutathione-nitrate reductase in the liver (43).
Intravenous Nitroglycerin
Dose and Administration
Intravenous NTG is available as 5- and 10-mg/mL solutions that are diluted with normal saline or 5% dextrose solutions to provide infusions of 100 mg NTG/250 mL (44). Onset of action is immediate, as is offset when the infusion is stopped. Glass bottles and nonpolyvinyl chloride plastic tubing must be used to avoid a loss of the active drug via absorption onto plastics (45).
Infusion rates are usually initiated at 10 to 20 μg per minute and titrated upward in a stepwise fashion using 10 to 60 μg per minute increments to predetermined endpoints, such as improvement of symptoms, development of the drug-related side effects, change in a systolic blood
pressure or pulmonary artery wedge pressure, or a maximum dose of 200 to 500 μg per minute (46,47).
pressure or pulmonary artery wedge pressure, or a maximum dose of 200 to 500 μg per minute (46,47).
Therapeutic Effects of Nitroglycerin in Patients with Heart Failure
Hemodynamic Effects
The potential hemodynamic effects of a therapeutic dose of NTG in patients with CHF include a substantial reduction in right and left ventricular filling pressure, systemic and pulmonary vascular resistance, and systemic blood pressure (Fig. 27-4). There is little or no change in heart rate, while cardiac output usually increases (46,47). The mechanisms for increased cardiac output include left ventricular and right ventricular afterload reduction, improvement in myocardial ischemia, and reduction in the degree of mitral regurgitation (44,48).
Effect on Mitral Regurgitation
Regurgitation of the mitral valve is common in patients with CHF, usually due to severe dilatation of the left ventricle and the mitral valve annulus. Use of intravenous (IV) NTG in a group of patients with chronic, nonischemic mitral regurgitation resulted in a reduction in LV and diastolic volume and mitral valve regurgitant area as well as a marked improvement in the severity of mitral regurgitation and the hemodynamic profile (48,49).
Neurohormonal Effects
The effect of acute and sustained IV NTG on hormone secretion was studied in nine patients with CHF by Webster et al. (Fig. 27-5) (50). NTG was administered at a dose uptitrated to achieve a 30% to 50% reduction in PCWP (50 to 245 μg per minute) and was associated with a substantial reduction in mean right atrial pressure and PCWP, a mild but significant reduction in systemic blood pressure (85 mm Hg to 78 mm Hg, p = 0.04), and no change in heart rate. These hemodynamic changes were associated with a reduction in plasma atrial natriuretic peptide, but also a significant increase in plasma aldosterone, cortisol, and epinephrine levels with a small and statistically significant increase in plasma NE or plasma renin activity.
The effect of IV NTG on arginine-vasopressin, plasma renin activity, aldosterone, and atrial natriuretic peptide was also evaluated by Dupuis et al. (47) in 13 men hospitalized with severe CHF (NYHA Class IV). The hemodynamic changes induced by NTG were accompanied by an increase in arterial epinephrine and plasma renin activity and a decrease in atrial natriuretic peptide. In contrast, there was no change in arterial NE, aldosterone, or arginine-vasopressin. By 6 hours of continuous infusion, arterial epinephrine levels returned to baseline values, possibly due to less-pronounced hypotension; however, plasma renin activity remained elevated and atrial natriuretic peptide remained decreased. Dakak et al. (51) also evaluated the hemodynamic and neurohormonal effects of 12 patients with severe heart failure. NTG dose was 276 ± 100 μg per minute, which resulted in considerable hemodynamic effects that were significantly attenuated due to nitrate tolerance after several hours of continuous infusion. NTG infusion was associated with a substantial increase in plasma renin activity and serum aldosterone, while atrial natriuretic peptide showed a marked but transient decrease in keeping with the development of hemodynamic tolerance.
Effect on Coronary Circulation
No information is available on the effect of IV NTG on coronary blood flow in patients with heart failure. In an unpublished study performed by our group, intracoronary administration of 200 μg NTG in a group of 25 patients with heart failure secondary to idiopathic dilated cardiomyopathy resulted in a significant dilatation of the epicardial coronary artery diameter by 8% ± 4%, as well as an increase in coronary blood flow by 42% ± 12% (44). These data suggest a combined effect of NTG on both the epicardial conductance as well as the resistance of coronary arteries in patients with heart failure due to idiopathic cardiomyopathy.
Effect on Renal Circulation
The effect of IV NTG on the renal circulation in patients with acute decompensated heart failure (ADHF) has not been studied. Infusion of NTG at a rate calculated to achieve blood concentration of 10-7, 10-6, 10-5 mol/L into
the renal artery in patients with heart failure resulted in a significant dilatation of the main renal artery with no significant effect on renal blood flow (52). These findings suggest a significant vasodilatory effect of NTG on large-conductance renal arteries but not on small-resistance vessels.
the renal artery in patients with heart failure resulted in a significant dilatation of the main renal artery with no significant effect on renal blood flow (52). These findings suggest a significant vasodilatory effect of NTG on large-conductance renal arteries but not on small-resistance vessels.
Potential Limitations of Intravenous Nitroglycerin in the Treatment of Acute Decompensated Heart Failure
Side Effects
Table 27-1 shows the reported side effects of IV NTG in 216 patients with ADHF, enrolled in the Vasodilatation in the Management of Acute CHF (VMAC) study (53). The most common adverse effect was headache, which was seen in 20% of the patients, followed by asymptomatic hypotension (8%), and nausea (6%).
Nitrate Resistance
A decreased vasodilatatory response and attenuated hemodynamic effect of nitrates have been reported in patients with heart failure. A recent study by Katz et al. (54) showed a twofold increase in femoral artery blood flow velocity with
intra-arterial infusion of NTG at a concentration of 10-7 mol/L in normal subjects. This response, however, was markedly attenuated in patients with heart failure and could be overcome only by increasing the dose to 10-5 mol/L. Potential mechanisms for vascular resistance to NTG include an increase in sodium and water within the vascular wall or an increased mechanical compression caused by accumulation of subcutaneous fluid (55), sulfhydryl group deficiency (56), and neurohormonal stimulation leading to activation of vasoconstrictive mechanisms including catecholamines, angiotensin II, endothelin, and vasopressin, which may attenuate the vasodilatory effect of the drug (57).
intra-arterial infusion of NTG at a concentration of 10-7 mol/L in normal subjects. This response, however, was markedly attenuated in patients with heart failure and could be overcome only by increasing the dose to 10-5 mol/L. Potential mechanisms for vascular resistance to NTG include an increase in sodium and water within the vascular wall or an increased mechanical compression caused by accumulation of subcutaneous fluid (55), sulfhydryl group deficiency (56), and neurohormonal stimulation leading to activation of vasoconstrictive mechanisms including catecholamines, angiotensin II, endothelin, and vasopressin, which may attenuate the vasodilatory effect of the drug (57).
Table 27-1 Adverse Effects of Nitroglycerin During First 24 Hours After the Start of Therapy in 216 Patients in VMAC Study | ||||||||||||||||||||||||||||||||||||||||||||||||
---|---|---|---|---|---|---|---|---|---|---|---|---|---|---|---|---|---|---|---|---|---|---|---|---|---|---|---|---|---|---|---|---|---|---|---|---|---|---|---|---|---|---|---|---|---|---|---|---|
|
Nitrate Tolerance
Early development of tolerance and marked attenuation of initial hemodynamic effects of IV NTG in hospitalized patients with heart failure have been demonstrated by a number of investigators. Elkayam et al. (46), in a randomized, double-blind, placebo-controlled study, documented the early development of tolerance in 31 hospitalized patients with heart failure, which resulted in a significant attenuation of hemodynamic effects (Fig. 27-6). Analysis of individual data showed the development of early tolerance in approximately half of the patients, which could not be predicted by baseline hemodynamic and neurohormonal values. Similar attenuation of hemodynamic effects of IV NTG within several hours of initiation of therapy in hospitalized patients with heart failure has been shown by other investigators (47). Potential mechanisms for the development of early tolerance to NTG effect include the activation of neurohormonal mechanisms (47).
Oral Nitrates
Hemodynamic Effects
Oral nitrates result in a substantial reduction in resting RV and LV filling pressures, systemic vascular resistance, and systemic blood pressure (56,58). There is little or no change in heart rate, and cardiac output is usually increased because of the reduction in LV afterload, decrease in pulmonary vascular resistance, improvement in myocardial ischemia, and reduction in the degree of mitral regurgitation (59).
The hemodynamic benefit of organic nitrates in patients with chronic CHF is also seen during both dynamic and isometric exercise. Hecht et al. (60) showed a significant decrease in PCWP, mean pulmonary arterial pressure, mean systemic arterial pressure, heart rate, systemic vascular resistance, and pulmonary vascular resistance, along with an increase in cardiac index, stroke volume index, and stroke work index during dynamic exercise in patients with chronic CHF. Elkayam et al. (61) studied the hemodynamic effect of nitrate therapy during isometric exercise in a similar patient population and showed nitrate-mediated prevention of unfavorable hemodynamic changes, including an increase in RV and LV filling pressure, pulmonary pressures, and systemic vascular resistance with a reduction in stroke volume and stroke work index.
Effect on Mitral Regurgitation
Regurgitation of the mitral valve is found in a large number of patients with CHF because of severe dilation of the left ventricle and the mitral valve annulus. Studies have shown a favorable effect of nitrates in patients with mitral regurgitation, with reduction in LV end-diastolic volume and mitral valve regurgitation area as well as marked improvement in the severity of mitral regurgitation and the hemodynamic profile (62). Similar nitrate-mediated hemodynamic improvement in patients with mitral regurgitation was shown during isometric exercise (61).
Hamilton et al. (62) showed a significant reduction in mitral and tricuspid valvular regurgitation after approximately 3 days of intensive vasodilator therapy, mainly with angiotensin-converting enzyme (ACE) inhibitors and oral isosorbide dinitrate (ISDN). Mitral and tricuspid regurgitation were significantly reduced as determined by two-dimensional and Doppler echocardiography with color-flow imaging in 14 patients with advanced heart failure secondary to dilated cardiomyopathy. This reduction was sustained after an average follow-up of 6 ± 2 months. In addition, mean left and right atrial volumes were reduced with initial therapy and showed a further decrease at 6 months.
Effect on Exercise Tolerance
Two early studies evaluated, in a randomized, double-blind fashion, the effect of a 3-month therapy that used oral ISDN on both maximal exercise time (63,64) and oxygen consumption in patients with chronic CHF, and showed a significant favorable effect of therapy. More recently, Elkayam et al. (65) published the results of a study regarding the effect of high-dose (50 to 100 mg), intermittent (12 hours per day) transdermal NTG in patients with chronic CHF, already treated by standard therapy that included ACE inhibitors, and showed a significant and sustained effect of therapy on maximal treadmill exercise time.
Potential Limitations of Oral Nitrate Therapy in Heart Failure
Side Effects
In a recent study (65), 46% of patients with moderate CHF developed headache during treatment with large-dose (2 to 4 mg per hour) transdermal NTG. Only 13% of the patients, however, discontinued therapy because of this side effect.
Nitrate Resistance
In a previous hemodynamic evaluation of the commonly used single ISDN dose (40 mg) in 99 consecutive patients with moderate and severe CHF (66), lack of hemodynamic response was found in almost half of the patients. A significantly higher mean right atrial pressure was found in nonresponders compared with responders. A dose increase to 80 or 120 mg in the nonresponders overcame resistance in 42% of the patients. Overall, almost half of the patients did not respond to the commonly recommended dose of 40 mg of ISDN and almost 25% did not respond at all, even to doses as high as 120 mg.
Nitrate Tolerance
The temporary nature of nitrate-mediated hemodynamic effects with a marked attenuation occurring within the first 24 hours of therapy has been shown by numerous investigators (67). One study showed early development of tolerance with the use of frequent dosing (every 4 to 6 hours) of oral ISDN given to patients with chronic heart failure (68).
Hydralazine
Hydralazine (HYD), a hydrazinophthalazine and a potent dilator of arterioles, was introduced as an antihypertensive agent more than 40 years ago. Its vascular effects have earned it a role in the management of CHF.
Basic Pharmacology, Metabolism, and Pharmacokinetics
HYD likely produces vasodilation through a modulating effect on intracellular calcium kinetics, and a HYD-induced elevation of cyclic adenosine monophosphate (cAMP) or cGMP has been proposed (69). In addition, indirect mechanisms, including HYD-induced alterations in sympathetic nervous system tone, release of prostanoids, and inhibition of thromboxane A2 biosynthesis have also been suggested (70,71,72). HYD is rapidly and almost totally absorbed from the gastrointestinal tract, with peak plasma concentration occurring 30 to 60 minutes after ingestion (69,72,73). HYD undergoes significant first-pass metabolism by the liver, which accounts for a bioavailability of 10% to 35% (69,74). More than 90% of administered HYD is cleared by the liver and the remainder by renal excretion. The HYD molecule is metabolized by acetylation, oxidative reactions, ring hydroxylation, and glucuronide conjugation (69,74). In genetically slow acetylators, higher plasma concentrations are achieved and much of the metabolism of HYD is shifted from acetylation to primary oxidative reactions and hydroxylation (69,73,74). Eighty to ninety percent of circulating HYD is protein-bound. Elimination half-life is 0.5 to 2.0 hours and the clearance rate can range from 30 to 150 mL/kg per minute. Whether CHF influences the pharmacokinetics of HYD has not been convincingly resolved (73,74,75).
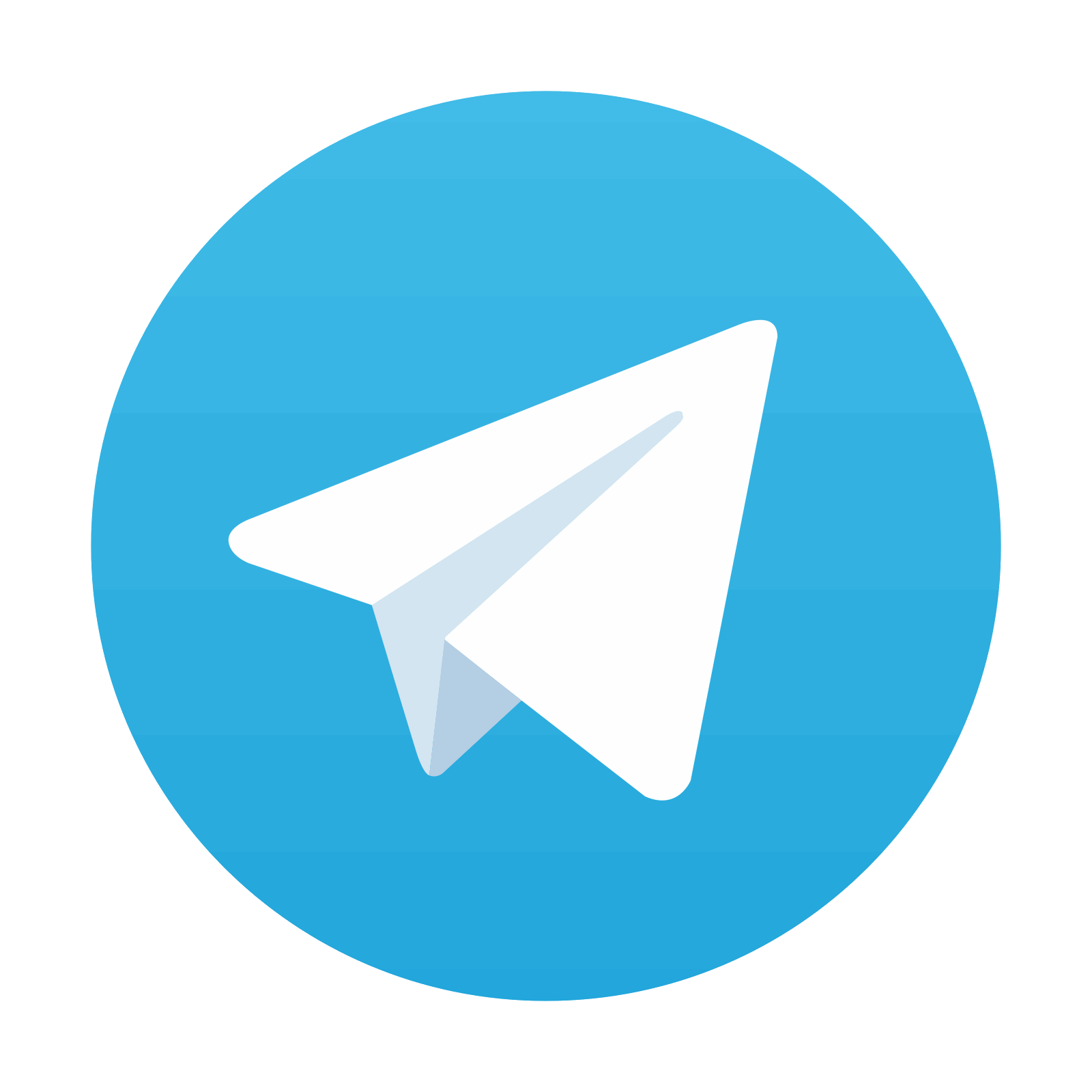
Stay updated, free articles. Join our Telegram channel

Full access? Get Clinical Tree
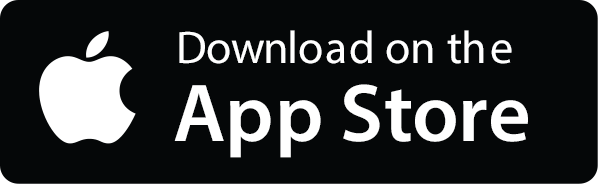
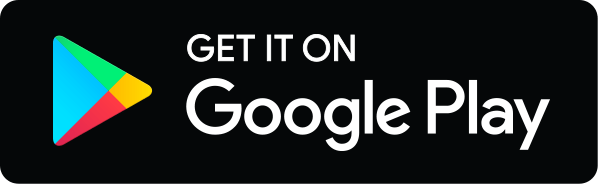