Vascular Physiology Fluid pressure is force that drives any fluid (blood) forward. Fluid pressure is dependent on the available fluid energy. Bernoulli’s principle: When fluid flows, the total energy (E) remains constant (in the absence of frictional losses). As fluid flows into an increased area, the velocity must fall so that the volume flow remains constant (i.e. falling kinetic energy). This is offset slightly by a small rise in the pressure (and a slight ↑ Ep). Poiseuille’s law: The volume flow rate (laminar flow) is given by the pressure difference divided by resistance to flow. This describes the viscous (frictional) energy losses occurring in an ideal fluid (Newtonian) and ideal system (non-pulsatile, straight cylindrical) and estimates the minimum pressure gradient for flow. The inertial energy losses in arteries (acceleration–deceleration pulsations, changes in luminal diameter and turbulent flow patterns at branching vessels) will exceed the minimum pressure gradient. These effects are even greater in diseased vessels. However, the energy losses are to the fourth power of the radius; therefore, the change in vessel radius will have an exponential effect on fluid flow. This is the effect the pressure (energy) drop has on flow rates (akin to Poiseuille’s law) and is dependent on radius of the vessel (r4), length of vessel (L) and viscosity of fluid. The radius is the predominant factor influencing resistance (πr4) and the normal PVR occurs at the arterioles–capillaries (60–70%) and the medium-sized arteries (15–20%). In addition, the inertial effects of fluid (v2) increase as velocity increases, thereby also increasing resistance (important with turbulence of disease). Any stenosis will also increase the PVR. Atherosclerosis commonly affects arteries that are normally low resistance. Therefore, the haemodynamic effects will have a significant impact on the normal flow physiology. Arterial flow patterns are determined by arterial geometry, vessel wall properties and flow velocities, all of which are affected by atherosclerosis. In turbulent (non-laminar) flow, the random variations in pressure and velocity will cause significant energy losses (heat), which are reflected as ‘spectral broadening’ on Duplex due to the non-parabolic flow (i.e. not flowing as a uniform column). As flowing blood enters a stenosis, it undergoes a ‘contraction zone’ followed by an ‘expansion zone’ as it exits. Both zones are areas of large (kinetic) energy losses (especially the expansion zone) as the high velocity jet dissipates its energy (area of post-stenotic turbulence). Thus, the radius of the stenosis will have a proportionately greater effect on energy losses than the length. In addition, an abrupt radius change will have a greater effect than a gradually tapering stenosis. This is the degree of narrowing required to produce a significant reduction in distal pressure and flow (50% reduction in arterial diameter or 75% reduction in area). However, the exact narrowing also depends on the flow (i.e. it may be subcritical at rest, becoming significant during exercise when the flow velocities increase). Unlike arterial flow, venous flow is non-pulsatile. In addition, veins are thin-walled (little smooth muscle) displaying both elasticity and collapsibility. The combination of thin compliant walls with a larger lumen allows for accommodation of larger volumes of blood (65% of circulating volume is contained in the veins). In addition, venous flow must equal cardiac output!
Arterial Physiology
Fluid Pressure and Fluid Energy
Determinants of Arterial Pressure and Flow
Fluid Energy
Energy Conservation
Fluid Energy Losses in Blood
Peripheral Vascular Resistance (PVR)
Haemodynamics of Disease
Critical Arterial Stenosis
Venous Physiology
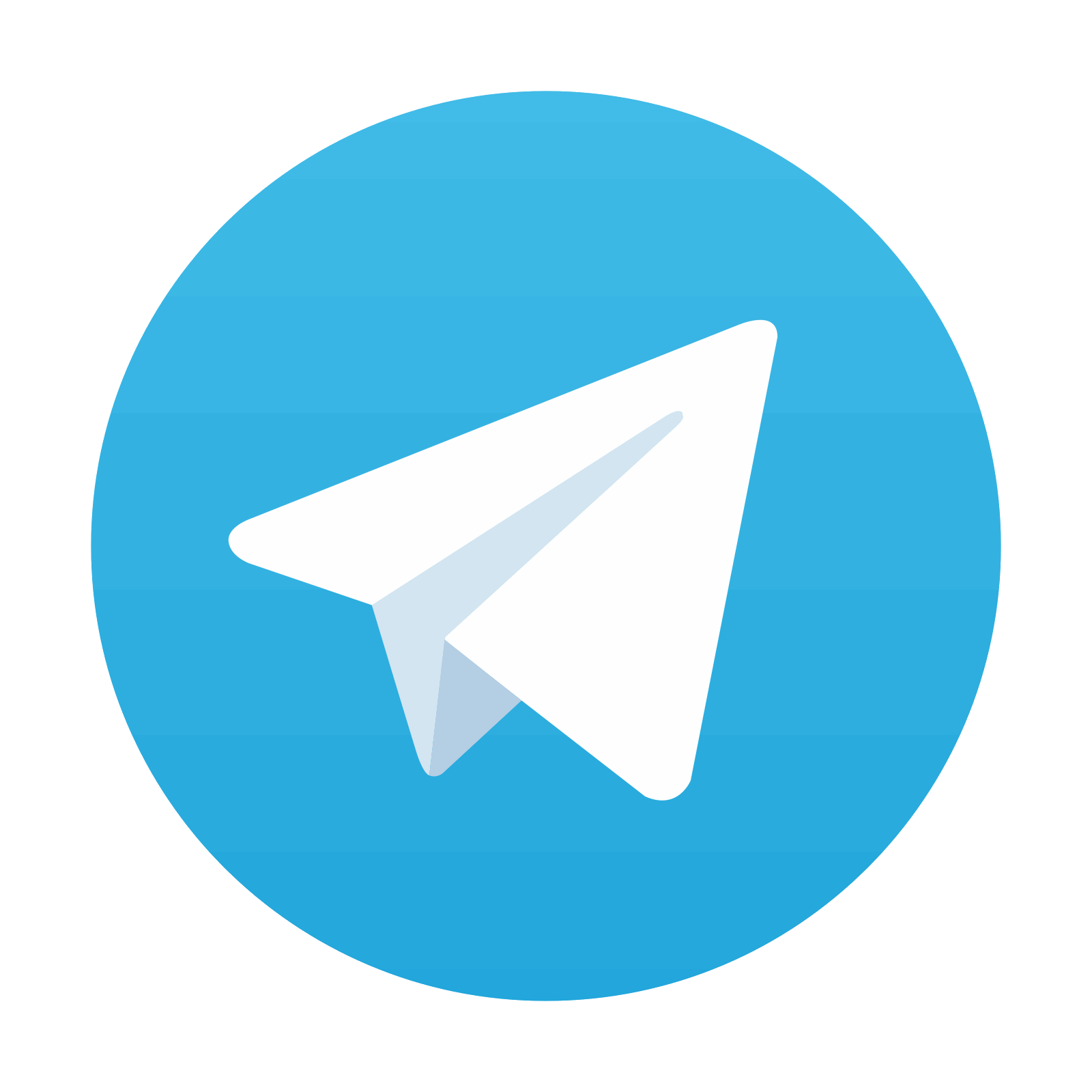