Protein
Varicose veina
Vein wall collagen
Increased
Vein wall elastin
Decreased
Vein wall laminin
Increased
Vein wall tenascin
Increased
Vein wall fibronectin
Similar
SMC collagen type I
Increased
SMC collagen type III
Decreased
SMC collagen type IV
Similar
SMC fibronectin
Decreased
Vein Wall Inflammation, SMC Dysfunction, MMP Expression, and Venous Dilation
A significant finding in varicose veins is the role of inflammation as a consequence of inflammatory cell infiltrate. Several studies have evaluated inflammatory cells and activation in varicose veins and normal control veins. In human saphenous vein specimens from patients with CVD, an increased number of monocytes/macrophage infiltration in the venous wall and valves was demonstrated. The inflammatory invasion is an important step in the events leading to inflammation, cytokine and proteinase production, and structural changes in the vein wall architecture. Elevated intercellular adhesion molecule-1 (ICAM-1), a marker of activation of leukocytes to adhere to the endothelium, has also been detected in CVD vein specimens, but not in normal veins [7]. This would suggest that certain predisposing factors and/or stimuli cause the vein endothelium to express ICAM-1, leading to leukocyte activation and infiltration, with further inflammatory responses that initiate the release of cytokines and MMPs. In fact, a study evaluating patients with venous hypertension demonstrated that there was sequestration of activated neutrophils and monocytes in the microcirculation. This persisted even after elevating the limbs and decreasing the venous hypertension, which indicated that leukocytes were adhering to the endothelium [8]. In another interesting study, plasma collected from patients with CVD caused significant granulocyte activation, which was more prominent in advanced stages of CVD (skin changes and ulcer). In addition, there was increased hydrogen peroxide production from activated granulocytes in the patient’s plasma than the control patient plasma [9]. These data suggested the presence of a circulating activating factor in the plasma of patients with CVD, which could activate the ICAM-1 on endothelium, initiating the early events that may be important in the pathophysiology of CVD and varicose veins.
Immunohistochemical studies of SMC cultured from varicose veins were found to have a decreased number of cells staining for collagen type III and fibronectin compared to control veins, although the transcriptional products (same amount of mRNA product) of these two proteins were not dissimilar in varicose veins versus control vein. In addition, the synthesis and deposition of collagen type III but not type I were significantly lower in varicose veins. When matrix metalloproteinases (MMPs) -1, -2, and -9 and the natural tissue inhibitors of MMPs (TIMPs) -1 and -2 were analyzed from the supernatant of confluent SMC, no differences were observed. These data suggested that the regulation as well as changes for both collagen type III and fibronectin in SMC was a post-transcriptional event [10]. Although there was no difference in MMP and TIMP in the supernatant tested, this did not exclude the possibility that altered expression, activity and other types of MMPs exist in whole tissues including TIMP. Further work in this area demonstrated that varicose greater saphenous vein had a smaller spiraled collagen distribution specifically in the intima and media. To investigate the latter findings, the same investigators demonstrated that inhibition of MMP with marimastat (BB-2516, non-selective MMP inhibitor) resulted in partial restoration in the production of collagen type III in smooth muscle cells from varicose veins. In addition, MMP-3, which degrades fibronectin, was elevated in both its transcription product and protein expression. It was concluded that the mechanism involved in collagen type III and fibronectin degradation in the smooth muscle cells cultured from varicose veins is likely linked to the expression of MMP-3 and its proteinase activity [11]. The important properties of type III collagen in blood vessels is the ability to provide elasticity and distensibility. The abnormal production of type III collagen in both SMC cultured from varicose veins and fibroblasts cultured from dermal biopsies of patients with CVD raises the possibility that varicose veins’ pathology may arise from abnormal matrix collagen deposition and is likely a systemic disease [12]. Furthermore, to identify factors involved in abnormal elasticity and distensibility in varicose veins, a study evaluated the content of hydroxyproline and quantified collagen types I, III, and V. It was found that in both SMC and fibroblast of patients with varicose veins, as compared to control, had an increase in hydroxyproline content, indicating increased collagen; however, the proportion of collagen type III was significantly reduced despite normal mRNA transcript. These data were consistent with previous reports and offered an explanation for the loss of distensibility and elasticity in varicose veins; they suggested that the defect is generalized, supporting a genetic basis for the alterations observed in patients’ varicose veins [13]. Taken together, these findings suggest that at least in cultured SMC from varicose vein, there is an imbalance of collagen production with dysregulation and increased type I collagen but reduced type III collagen production. Because of normal expression of mRNA for type III collagen, the reduction in synthesis is related to post-transcriptional events. The inhibition of type III collagen synthesis could be a result of degradation/inhibition by MMP-3 and may explain changes in the mechanical properties of the vein wall leading to inappropriate elasticity and distensibility, which results in varicose vein formation. Finally, the similar abnormalities found in dermal cultured fibroblasts indicate that the problem of varicose veins is a systemic disease, likely with genetic factors involved.
Localization of MMPs and Significance, Models of Venous Hypertension and MMP Activation, MMP Modulation by Flavonoids
A possible explanation for venous dilation and tortuosity may be the influences of MMPs and TIMPs, which lead to venous wall remodeling and subsequent dilatation and valve incompetence. Several authors have found an increased expression, localization, and activity of MMPs in the venous segments of varicose veins and in veins with thrombophlebitis compared to control veins [3, 14, 15]. It is unclear whether MMPs are present because of a secondary process due to cellular inflammatory infiltration and wall remodeling or directly involved in the formation of varicose veins. The question is how MMPs cause venous dilation and varicose vein formation. The role of MMPs in varicose vein has largely been attributed to their proteolytic effects on extracellular matrix (ECM), degradation of the valve leaflets, and weakening of vein wall structure [16, 17]. The localization of MMPs in the varicose vein wall adventitia and fibroblast is consistent with a role in ECM degradation [15]. However, in varicose veins, MMPs have also been localized in the vicinity of the endothelium and SMC [15, 18], raising the possibility of additional effects of MMPs on these cell types. Other studies have investigated the ratio of TIMP-1/MMP-2 and found a threefold ratio increase in varicose veins compared to normal veins, concluding that proteolytic inhibition and ECM accumulation may account for the pathogenesis of varicose veins [19].
Animal models of venous hypertension utilizing a femoral artery and vein fistula in the rat have demonstrated an increased sustained venous pressure above 90 mmHg with significant abnormal structural changes in the vein valve and wall. In addition, there was significant expression of MMP-2 and MMP-9 at 6 weeks [20]. In a similar animal model, untreated veins developed venous hypertension with reflux and morphologic changes in the vein wall and valve, but in veins treated with the flavonoid Dalfon, which reduces inflammation by modulating inflammatory cells, there were reduced physiologic and anatomic changes in the vein wall and valves as a response to venous hypertension [21]. Importantly, these two latter studies demonstrated the feasibility of a venous hypertensive model, the implications of MMPs, and how certain drugs may alter the effects of inflammation on the vein wall to maintain valve function and reduced venous wall destruction.
MMPs and Effects on Endothelial and SMC Venous Function
It is possible that MMPs may have acute and chronic effects on the vein wall. Early changes may cause functional and metabolic changes to the vein wall, while the later effects of MMPs may alter wall matrix composition to such an extent that dilation and tortuousity becomes the prominent morphological feature [2]. In recent studies, evidence for how MMPs acutely influence venous wall dilation was determined. In a rat inferior vena cava (IVC) model where exogenous MMP-2 was added, changes in vein wall contraction resulting in relaxation was recorded. MMP-2 caused time-dependent venous relaxation in phenylephrine-contracted IVC. However, MMP-2-induced venous relaxation was essentially abolished in 96 mmol/l KCl depolarizing solution, which prevents outward movement of K+ ions from the cell through K+ channels, which is necessary during venous relaxation in hyperpolarized vascular tissue. In order to define which K+ channels were involved, the investigators tested the effects of K+ channel agonists and antagonists on MMP-2-induced venous relaxation. MMP-2 caused further relaxation of vein segments in the presence of activators of the ATP-sensitive potassium (KATP) channel, indicating that MMP-2 was not working through the KATP channel during cell hyperpolarization (a condition of negative membrane potential caused by outward potassium ion movement leading to smooth muscle cell relaxation and resulting in venous relaxation). In contrast, blockade of the large conductance Ca2+-dependent K+ channels (BKCa) with iberiotoxin significantly inhibited the MMP-2 effect on venous relaxation, suggesting that MMP-2 actions in part involve hyperpolarization by activation of BKCa. MMP-2 induced activation of K+ channels likely causes SMC hyperpolarization and leads to decreased Ca2+ influx through voltage-gated channels [22]. In addition, it was determined that nitric oxide (NO) was abundant in acetylcholine-stimulated IVC. However, in the presence of MMP-2, there was no measurable increase of NO, indicating that the NO-cGMP pathway was not stimulated by MMP-2-induced relaxation. In addition, using inhibitors of the NO-cGMP (L-NAME) and PGI2-cAMP (indomethicin) pathways did not cause inhibition of MMP-2-induced relaxation of phenylephrine-contracted IVC, indicating that the MMP-2 mechanism likely involves hyperpolarization, resulting in venous dilation [22, 23]. Taken together, these data demonstrated a novel effect of MMPs on venous tissue function and suggest that protracted MMP-2 induced venous relaxation could lead to progressive venous dilatation, possibly influencing the venous wall before changes in the valve occur, and leading to varicose vein formation [22].
In the same rat IVC and venous isometric contraction apparatus, it was determined that MMP-2 attenuates [Ca2+]e-dependent vascular smooth muscle (VSM) contraction (by inhibiting Ca2+ entry into the smooth muscle) without affecting Ca2+ release from intracellular Ca2+ stores. In addition, in an effort to determine the mechanism of MMP-2 induced vasorelaxation, it was found that MMP-2 induced VSM relaxation does not involve the generation of RGD or activation of αvβ3 integrin receptor (RGD contains the Arg-Gly-Asp tripeptide known to activate integrin receptors and lead to membrane hyperpolarization). From this study, it was concluded that MMP-2-induced inhibition of the Ca2+ entry mechanism of VSM contraction may play a role in the venous dilation associated with varicose vein formation [24].
< div class='tao-gold-member'>
Only gold members can continue reading. Log In or Register a > to continue
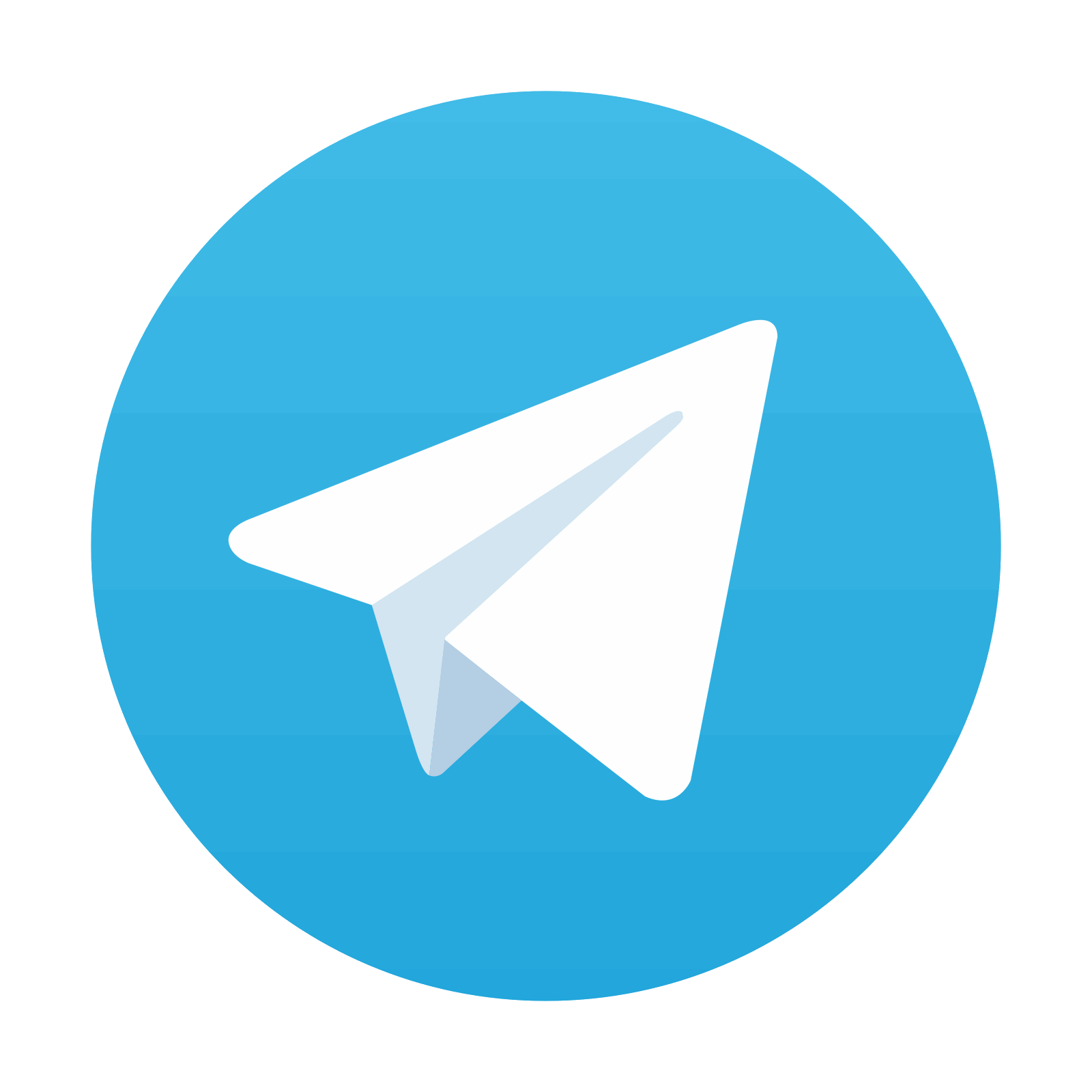
Stay updated, free articles. Join our Telegram channel

Full access? Get Clinical Tree
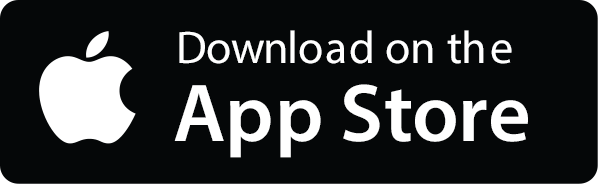
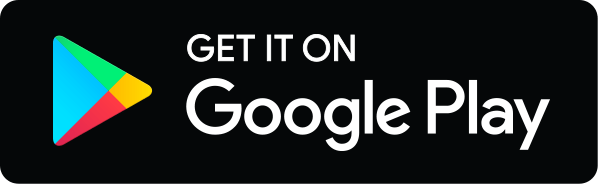