The cardiac valves permit efficient forward blood flow while preventing backflow, in turn creating unidirectional forward cardiac output. Valve disease leads either to reduction in orifice area (stenosis), impeding forward flow, or to incompetence, permitting either regurgitant flow or a combination of these effects. Valve stenosis exerts a pressure overload on the ventricle behind the stenotic valve because that chamber must generate increased pressure to drive the bloodstream past the narrowed orifice. Valve incompetence exerts a volume overload on the affected ventricle, which must enlarge to compensate for the forward flow that is lost to regurgitation. The effects of these overloads on ventricular function and geometry are dealt with in subsequent chapters. This chapter focuses on the evaluation of valve lesion severity and the effects of valve disease on hemodynamics.
Usually in early diastole, there is a small gradient across the mitral valve that initiates filling and then rapidly dissipates (Fig. 8-1A).1 Indeed, the normally opened mitral orifice is 4 to 5 cm2, which creates a functionally single chamber of left atrium and left ventricle (LV) during diastole; thus, pressures in both chambers are equal. Mitral stenosis, which is usually caused by rheumatic heart disease, reduces orifice area, in turn causing a gradient between left atrium and LV. Mitral stenosis eventually leads to pronounced hemodynamic effects, although little hemodynamic disturbance develops until orifice area is compromised to less than half its normal size. As stenosis worsens, the pressure gradient between left atrium and LV becomes larger and longer in duration (Fig. 8-1B). This gradient is added to normal left atrial pressure, causing left atrial hypertension, eventually leading to pulmonary congestion. As mitral stenosis worsens further, reduced valve aperture impairs LV filling, limiting cardiac output. Thus, despite usually normal LV muscle function, the hemodynamics are those of LV failure. Although left atrial contraction assists late atrial emptying, most transmitral flow occurs early in diastole, and much of the propellant force creating the pressure driving blood across the valve ultimately is derived from the right ventricle. Therefore, it is this chamber that is pressure overloaded by the disease. Still further stenosis of the mitral valve causes secondary pulmonary vasoconstriction, reinforcing and worsening pulmonary hypertension and right ventricular pressure overload.
FIGURE 8-1
A. Simultaneous normal left ventricular (LV), aortic (Ao), and pulmonary capillary wedge pressure (PCW) tracings are shown. DFP, diastolic filling period; ECG, electrocardiogram; SEP, systolic ejection period. B. Simultaneous LV and PCW tracings from a patient with mitral stenosis are shown. The shaded area represents the transmitral gradient. (Reproduced with permission from Carabello BA, Grossman W. Calculation of stenotic valve orifice area. In: Baim DS, Grossman W, eds. Grossman’s Cardiac Catheterization, Angiography, and Intervention. 6th ed. Philadelphia, PA: Lippincott Williams & Wilkins; 2000:193–209.)

Because transvalvular gradient is flow dependent, a measure of stenosis severity that incorporates both flow and gradient is helpful in assessing stenosis severity. Valve area has become the most widely used of these measures.2 Flow (F) is the product of orifice area (A) and stream velocity (V) (F = A × V). Rearranging the terms, A = F/V. When using Doppler echocardiography to assess orifice area, velocity is measured directly. In using invasive hemodynamics to assess stenosis severity, the transvalvular pressure gradient is measured and converted to velocity using the formula , where g is the acceleration as a result of gravity (980 m/s) and h is the mean pressure gradient. Thus, valve area equals
. Because flow normally tends to stream through the center of an orifice, physiologic valve area is smaller than actual anatomic valve area. This property is corrected using a constant of orifice contraction, Cc. Because not all of the pressure gradient is converted to flow (some energy is lost to friction), another constant, that of velocity loss (Cv ), is used. In 1951, Richard Gorlin and his father2 proposed the following equation for calculation of valve area:
Flow is expressed as cardiac output during the time in diastole that the mitral valve is open (diastolic filling period, dfp). Thus the final formula is
where MVA, is mitral valve area, CO equals cardiac output in L/min, and HR is heart rate.
The Gorlins were unable to develop the constants for the equation. Instead, they compared their calculated results to actual valve areas from autopsy and surgical specimens and applied an empiric constant of 0.85 to the denominator. By doing so, the recalculated valve areas came to within 0.2 cm2 of the actual valve areas. Even as newer noninvasive methods have become available for quantifying mitral valve area, the Gorlin formula has remained a gold standard for valve area determination.
As noted previously, several noninvasive methods have been developed for the determination of mitral valve area. The two most commonly used are the pressure–half time method and direct planimetry.3,4 In general, these methods are adequate for clinical decision making. However, in some cases, there is a discrepancy between clinical and laboratory assessment of stenosis severity; in such cases, the additional information from invasive evaluation is helpful in making a final decision regarding the severity of a given patient’s mitral stenosis and its management. The usual cause of discordance between clinical and noninvasive assessment of the severity of mitral stenosis occurs in the patient who manifests ambiguous symptoms of heart failure while valve area is calculated to be in the mild to moderate range. Here, an invasive evaluation of hemodynamics can settle the issue. Evaluation of left atrial pressure (pulmonary capillary wedge pressure [PCWP]), cardiac output, and pulmonary artery pressure at rest and during exercise is usually very illuminating. Regardless of calculated valve area, if wedge pressure and transvalvular gradient are either significantly increased at rest or more likely provoked to increase with exercise, the hemodynamics explain the patient’s symptoms and suggest that mechanical relief of obstruction will lead to clinical improvement. If exercise does not provoke significant hemodynamic abnormalities, causes other than the patient’s mitral stenosis should be sought to explain the patient’s symptoms. Unfortunately, in today’s busy interventional catheter laboratories, careful assessment of hemodynamics, especially with exercise, has become a rarity.5
In the absence of transseptal puncture, left atrial pressure is estimated from the PCWP. There is much debate concerning whether this is a reliable left atrial pressure surrogate.6,7 In fact, the PCWP is or is not reliable, depending on the technique used to measure it. Apart from noting that the catheter appears to be wedged and has (in sinus rhythm) typical a and v waves, it is crucial to confirm that the catheter is truly wedged by removing highly oxygen-saturated (>90%) blood from the catheter while in the wedged position.1,7 Failure to perform this confirmatory step often results in recording a pressure that is hybrid between PCWP and pulmonary artery pressure, in turn overestimating both the PCWP and the transvalvular gradient, often by as much as 5 mm Hg. In addition, the transducers measuring both the LV pressure and PCWP must be properly calibrated and zeroed. Although recording device internal standards for calibration have improved over the years, there is no substitute for mercury calibration, considering that errors of just a few mm Hg may have an impact on assessment of stenosis severity of the mitral valve, where a gradient of just 5 mm Hg is often consistent with severe disease.
The transmitral flow period used in calculating the valve area is the entire time the valve is open in diastole. This period is measured (see Fig. 8-1B) from the time the LV pressure tracing falls below PCWP in early diastole (valve opening) to where LV pressure exceeds PCWP in early systole (valve closing). However, the use of the PCWP to measure left atrial pressure causes an 80-millisecond delay from the actual pressure event in the left atrium and its recording as PCWP. Because the LV pressure is recorded directly, the 2 pressures become nonsimultaneous. This problem is corrected by tracing the PCWP recording on paper or electronically reimposing it on the LV tracing using the correct timing of the actual pressure events. Failure to make this maneuver leads to substantial overestimation of the transvalvular gradient and inaccurate measurement of the diastolic filling pressure.
Mitral regurgitation adds a second error in assessing valve area. The pressure gradient is produced by the total flow crossing the valve. The cardiac output determination measures only forward flow, thus underestimating total flow and, as a result, underestimating mitral valve area.
Finally and obviously, the cardiac output must be measured accurately. Although the gold standard for this determination is the Fick method, thermodilution is often substituted.8,9 Although this technique is usually accurate, accuracy diminishes in the presence of tricuspid regurgitation and low cardiac output, conditions commonly present in patients with mitral stenosis. In some catheterization laboratories, a “pseudo-Fick” approach is used, in which oxygen consumption is assumed from tables of normals adjusted for height and weight. Because patients with mitral stenosis are hardly normal with respect to cardiac physiology, this technique is fraught with error and should be avoided.10
As with mitral stenosis, little hemodynamic disturbance occurs from aortic stenosis until the valve orifice area is reduced to less than half its normal 3.0 to 4.0 cm2. Serious clinical consequences usually do not occur until aortic valve area is reduced to less than 1.0 cm2 when the classic symptoms of angina, syncope, and heart failure develop, presaging sudden death.11,12 As with mitral stenosis, aortic stenosis severity is usually assessed accurately noninvasively. An abundance of comparison studies show remarkable concordance between gradient measured invasively and gradient measured using Doppler echocardiography. Likewise, there is excellent concordance between invasively derived valve areas and those derived noninvasively using the continuity equation.13,14 Thus, in many catheterization laboratories, only coronary arteriography is performed prior to contemplated aortic valve replacement with stenosis severity assessed noninvasively prior to catheterization.
Invasive assessment of aortic stenosis, like that of mitral stenosis, uses the Gorlin formula. Systolic ejection period is substituted for diastolic filling period (see Fig. 8-1A).1 However, unlike mitral stenosis, the Gorlins had no data to compare calculated to actual valve area because, at the time, it was considered malpractice to cross the aortic valve with a catheter. Thus, an empiric constant was never developed for the aortic valve area calculation.15 Both the invasively derived and the noninvasively derived areas for the aortic valve are flow dependent, varying directly with flow.16,17 Two explanations are usually given for this phenomenon. First, it may be that as flow through the valve is increased, the orifice truly opens wider. Second, there is speculation that the absence of knowing the discharge coefficient (or empiric constant) affects the calculation. In fact, there are data to support either point of view.18,19
The current American College of Cardiology/American Heart Association guidelines for the treatment of valvular heart disease define severe aortic stenosis as present when peak echo jet velocity is ≥4.0 m/s, when mean transvalvular gradient is ≥40 mm Hg, or when aortic valve area is ≤1.0 cm2.20 Unfortunately, many patients show discordance among these 3 criteria and also between the clinical and objective assessment of disease severity.21 Invasive assessment of aortic stenosis can be helpful in resolving these discrepancies in several situations. First, invasive assessment is useful when the clinical impression of the patient’s symptoms and lesion severity does not agree with the noninvasive data; for example, in a patient with angina and a physical exam consistent with severe disease but an echocardiographic Doppler exam that shows a gradient of only 20 mm Hg, it is likely that off-axis imaging of the aortic jet velocity has caused its underestimation. Second, invasive evaluation is helpful when features of the noninvasive exam are inconsistent with one another; that is, the aortic valve opens well, yet there appears to be a large transvalvular gradient. Third, the invasive approach is often helpful in assessing the very difficult patient with low output and low gradient. Some patients in this group have impaired systolic function and reduced ejection fraction, while others have normal ejection fraction but severe concentric hypertrophy that has reduced end-diastolic volume and concomitantly reduced stroke volume. In the group with reduced ejection fraction, there is far-advanced LV dysfunction, leading to both high operative and late mortality rates (Fig. 8-2).22,23 However, patients in this group who augment their gradient and forward output during dobutamine stress show inotropic reserve and have a relatively good outcome following aortic valve replacement, whereas patients who fail to augment their gradient and forward output during dobutamine stress have a poor prognosis (Fig. 8-3).24,25 Although dobutamine is often administered in the echo lab, administration during catheterization allows preanalysis of the coronary anatomy. This is potentially important, because patients with severe coronary disease might fail to augment during dobutamine infusion, not because they lack inotropic reserve, but because dobutamine-induced ischemia from coronary disease precludes an increase in cardiac output. In the group with low gradient and normal ejection fraction, inotropic reserve can be used to calculate gradient and valve area at a higher cardiac output, confirming that truly severe aortic stenosis is present.
FIGURE 8-2
A. Kaplan-Meier survival curve for 52 patients with aortic stenosis, decreased LV function, and low transvalvular mean gradient (MG) compared with expected survival. B. Kaplan-Meier survival curve for patients with aortic stenosis, decreased LV function, and low MG (<30 mm Hg) compared with patients with aortic stenosis, decreased LV function, and MG ≥30 mm Hg. Operative risk was 21%. (Reproduced with permission from Connolly HM, Oh JK, Schaff HV, et al. Severe aortic stenosis with low transvalvular gradient and severe left ventricular dysfunction: result of aortic valve replacement in 52 patients. Circulation. 2000;101:1940–1946.)
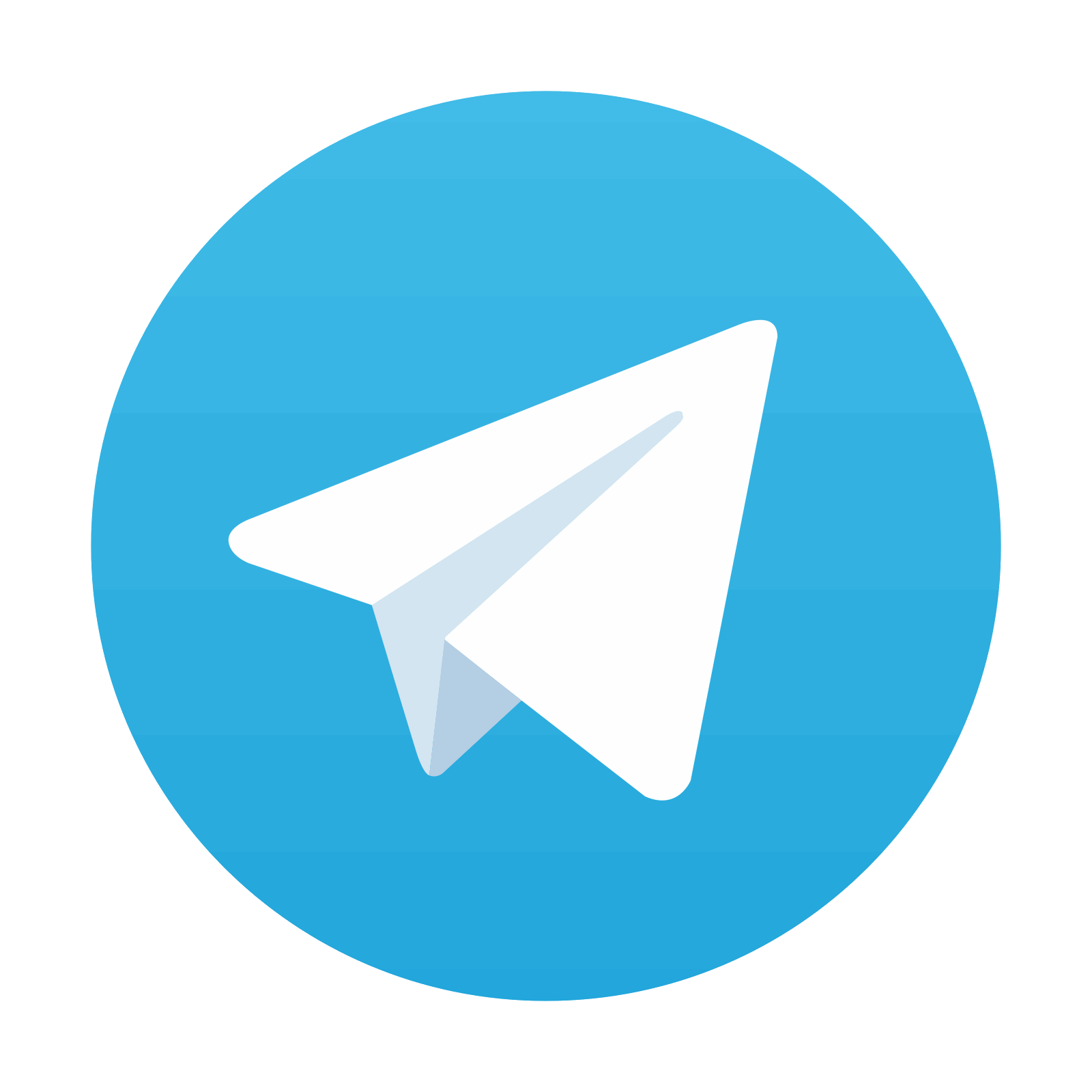
Stay updated, free articles. Join our Telegram channel

Full access? Get Clinical Tree
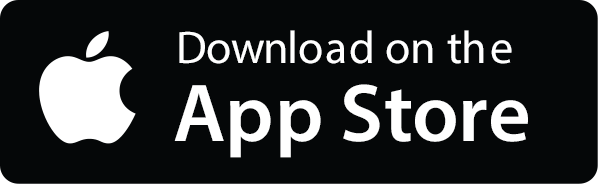
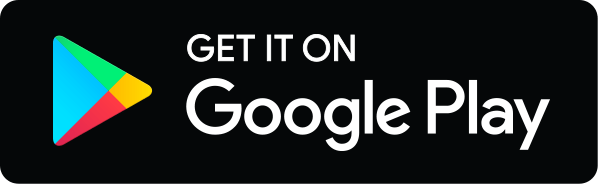
