Fig. 9.1
Panel a: Morphological changes in the left ventricle in response to aortic valve stenosis . The initial response of concentric hypertrophy and heart failure with preserved ejection fraction gives way to a dilated cardiomyopathy, pulmonary hypertension, and reduced cardiac output. Panel b: Typical changes associated with the progressive aortic valve stenosis
The Aortic Valve
The normal aortic valve has three thin cusps that reflect from and are supported by the sinus of Valsalva. During systole, the valve is opened by the blood being ejected from the ventricle to the aorta, creating an orifice that has an effective cross-sectional area of 2.5–3.5 cm2 and trivial pressure gradient between the ventricle and aorta. During diastole, the cusps are pushed shut by the pressure gradient between the aorta and ventricle. When the cusps coapt together, the back-pressure load of the aortic blood causes the cusps to pull the sinus of Valsalva inward and the cusp tissue to stretch. Hence, a normal aortic valve is dynamic and that movement aids valve sealing in diastole.
Aortic Valve Stenosis
One to two percent of the population have a congenitally abnormal valve, the most common of which is a failure of the cusps to separate in fetal life. This leads to a bicuspid valve with one large (fused) cusp and one small (normal) cusp [1]. Bicuspid valves have turbulent blood flow during systole, leading to thickening and fibrosis and retraction of the valve tissue over time. Males are affected twice as often as females.
Patients with bicuspid valves typically present with severe aortic valve stenosis in their teens to 60s. As the valve orifice becomes fibrotic, it calcifies, adding further to the valve rigidity and obstruction of ventricular outflow. Progressive stenosis leads to a pressure gradient across the valve during systolic blood ejection. The restricted blood flow jet through a stenotic valve stimulates dilation of the aorta just above the sinus of Valsalva, presumably related to the high-velocity jet stimulating aortic growth.
The second major cause of aortic stenosis is a poorly understood degenerative process in which the valve tissue becomes fibrotic and calcified. Both atherosclerosis and inflammatory processes have been suggested as the inciting causes, but at present there is no convincing evidence for any etiology. The degenerative process is slow. The average reduction in effective orifice area (EOA) is 0.1 cm2/year. Most patients with severe aortic stenosis from a degenerative valve present in their 60s and beyond.
The third common cause, particularly in developing countries, is rheumatic heart disease, where a valvulitis leads to late fibrosis and calcification of the valve. Rheumatic disease of the aortic valve is usually accompanied by thickening of the mitral valve; the absence of mitral thickening makes a rheumatic etiology unlikely. Like bicuspid valvular disease, degenerative and rheumatic aortic valve disease leads to aortic dilation and a high-velocity jet across the valve, although the jet is usually seen with less intensity in bicuspid valves.
The increased pressure load on the ventricle imposed by valve restriction initiates a concentric hypertrophic process. Sarcomeres proliferate in parallel to each other, causing cardiomyocytes to grow in diameter and increasing the thickness of the ventricular wall. In later stages, the fibrous connective tissue between myocytes also proliferates. The hypertrophy causes the ventricular cavity to shrink in volume and the muscle to become less compliant. Longitudinal shortening of the ventricle all but disappears as the fibers rearrange their orientation, reducing the efficiency of cardiac contraction.
The hypertrophy and valvular obstruction initially cause heart failure with preserved ejection fraction. Stroke volume is diminished by the small left ventricle (LV) chamber size and reduced diastolic left ventricle filling due to reduced left ventricle compliance. High systolic intraventricular pressure creates increased pressure load on the mitral valve, potentiating functional mitral regurgitation. Higher diastolic left ventricle filling pressure leads to left atrial dilation and, eventually, to pulmonary hypertension and right heart failure. The elevated atrial pressure causes dilation of the mitral annulus, which in turn causes or augments the degree of mitral insufficiency.
This syndrome of hypertrophy-related diastolic abnormalities occurs most often in older women, many of whom have markedly reduced cardiac output in the face of normal left ventricle ejection fraction. In advanced stages, cardiac output declines, reducing renal perfusion and leading to fluid retention and congestive heart failure [2].
A contributing cause of elevated diastolic ventricular pressure is a combination of valve restriction and insufficiency . The same processes that cause stiffening of the valve cause tissue retraction and poor coaptation of the cusps. The resulting diastolic leak through the valve increases diastolic filling pressure and, in some patients, may be a major cause of symptoms.
As the pressure overload continues and the valve restriction becomes more severe, the ventricle may dilate, and systolic function, as measured by ejection fraction, declines. This often leads to functional mitral regurgitation, reduced cardiac output, atrial fibrillation from left atrial distension, and a spiral to severe heart failure and death.
Symptoms
The cardinal symptoms of aortic stenosis are chest pain, dyspnea, and syncope. Hypertrophy and high pressure-induced workload lead to myocardial subendocardial ischemia, reduced diastolic relaxation, and chest pain. The epicardial arteries feeding the muscle send penetrating branches through the thickened muscle. These branches are compressed, reducing availability of subendocardial blood flow. In addition, although the muscle mass increases significantly, the number of vascular channels does not, reducing the ratio of vascular cross-sectional area to muscle mass. Hypertrophy and subendocardial ischemia lead to dyspnea on exertion.
Of note, while many believe that syncope in patients with aortic stenosis results from vasodilation in the face of inadequate cardiac output, it usually results from reflexive dilation of the peripheral circulation in response to excessive intraventricular pressure [3]. This explains in part why it often occurs just after exercise and often disappears as the ventricle dilates and fails—and no longer generates very high pressures.
Diagnosis
The degree of valvular stenosis is usually defined by the mean systolic pressure gradient across the valve or the effective orifice area. Gradients from 10 to 40 mmHg imply moderate stenosis. Gradients over 40 mmHg imply severe stenosis. The problem with using pressure gradient as the measure of valve stenosis is that it is dependent on stroke volume. Patients with reduced stroke volume, whether from loss of left ventricle chamber dimension from concentric hypertrophy or from reduced systolic contraction, have a reduced systolic pressure gradient for a given level of stenosis. This can lead to some confusion about the severity of the stenosis because patients with severe aortic valve obstruction can have a low-pressure gradient due to a low stroke volume.
Effective orifice area, an engineering calculation to estimate the orifice of a rounded-edge pipe obstruction, theoretically reflects the degree of obstruction, independent of stroke volume. When the EOA falls below about 0.8 cm2/m2, a pressure gradient develops across the valve and the ventricle begins the hypertrophic process. As the valve disease progresses, the hypertrophy intensifies. The hypertrophy reduces ventricular compliance, leading to mild diastolic heart failure. Most patients report mild reduced exercise capacity during this phase. As the EOA falls below 1.0 cm2, symptoms of chest pain, syncope, and congestive heart failure develop. When the EOA falls below 0.5 cm2, the risk of sudden death increases and survival is markedly reduced.
The calculation of EOA requires measurement of the mean systolic pressure gradient and the stroke volume. These can be easily measured invasively in the cardiac catheterization laboratory, but precise measurements are increasingly less reliable in low cardiac output states. Similarly, echocardiographic estimates of cardiac output from the continuity equation and valve pressure gradient by Doppler methods are less reliable in conditions of low cardiac output and ventricular dilation. This leads to diagnostic uncertainty in patients with a relatively low transvalvular gradient but typical symptoms of aortic stenosis, particularly if there are other causes for heart failure (such as prior myocardial infarction).
Another parameter to assess the degree of aortic valve obstruction is the concept of valve impedance (Z) [4]. As in an electrical circuit, valve resistance is completely independent of blood flow. The concept is appealing because it characterizes the “load” on the ventricle during contraction. Unfortunately, this parameter is not often used in clinical practice or research reports.
Efforts to refine the contribution of aortic stenosis to the myopathic process have centered on assessments of valve gradient and EOA with inotropic stimulation. The most common test is a dobutamine infusion stress echo, where a low dose of dobutamine is infused intravenously as the valve gradient and cardiac output are measured [5]. Patients with viable myocardium and severe aortic stenosis have an increased stroke volume and valve gradient and a reduction in measured EOA. Those with a primarily myopathic process have no change in cardiac output or a rise in output but no change in EOA (◘ Fig. 9.2).


Fig. 9.2
Dobutamine infusion stress test to assess myocardial viability and the relative degree of aortic stenosis and ventricular failure in patients with “low-gradient” aortic stenosis. Panel a: Normal response to dobutamine (increase in cardiac output and aortic valve gradient). Panel b: Low-gradient mild aortic stenosis with small increase in valve gradient during dobutamine infusion. Panel c: No change in valve gradient, a reduction in cardiac output, and hypotension during dobutamine infusion in a patient with very severe aortic stenosis. This is an example of low cardiac output, low valve gradient, and severe, advanced, aortic valve stenosis. Source: Nishimura et al. Circulation. 2002;106(7):809–13
Medical Therapy of Aortic Stenosis
In general, medical therapy is used to palliate the symptoms of aortic stenosis but it has no effect on disease progression. Although counterintuitive and previously thought to be harmful, many patients both tolerate and improve with the use of nitrates and vasodilators. Many older patients with severe aortic stenosis have both a high transaortic valve pressure gradient and a high systolic blood pressure beyond the stenotic valve (due to reduced vascular compliance). The ventricle “feels” the total resistance to blood flow ejection, both valvular and postvalvular.
Reduction of systolic blood pressure can improve symptoms. The caveat is that some patients with severe aortic stenosis and limited cardiac output reserve can develop syncope if exposed to excessive vasodilation, particularly if they are volume depleted (such as upon awakening or from excessive diuretic therapy). Careful and judicious dosing of vasodilators is important.
In patients with severe aortic stenosis, reduced left ventricle systolic function, and severe congestive heart failure, cautious use of nitroprusside (in an intensive care unit setting) can increase cardiac output and alleviate congestion. In these patients, reduced systemic vascular resistance is compensated for by a rise in cardiac output.
Atrial fibrillation is a common “tipping point” for patients with severe aortic stenosis. Patients with new onset of congestive heart failure due to aortic stenosis can often see improvement from cardioversion. Atrial fibrillation tends to recur, however, and cardioversion should be seen as a temporary solution before valve surgery.
Surgical Aortic Valve Replacement
The definitive treatment for severe aortic valve stenosis is valve replacement. Prosthetic heart valves increase the EOA from less than 1.0 cm2 to about 1.5–2.5 cm2, depending on the valve type and size. Aortic valve replacement (AVR) usually leads to a remarkable improvement in symptoms and regression of much of the acquired ventricular adaptations. Hypertrophy regresses, ejection fraction improves, and functional mitral regurgitation lessens [6–8]. For patients with aortic stenosis, maximal regression of hypertrophy and improvement in left ventricle function take an average of 2 years. The ventricular fibrosis that occurs over time in patients with severe aortic stenosis and heart failure is associated with persistent left ventricle dysfunction after AVR [9]. Patients with more severe preoperative left ventricle systolic dysfunction or an aortic valve gradient <40 mm Hg (often indicating low stroke volume and more advanced disease) have a less complete recovery of left ventricle dimensions and symptoms [10]. Although markedly improved, mortality is still more than that of age-matched controls.
Of importance, the use of a small prosthetic valve (e.g., 21 mm or less), such that the postoperative valve area is less than 0.85 cm2/m2, often fails to eliminate the heart failure symptoms of aortic stenosis because the patient is still left with moderate to severe stenosis. This “patient-prosthesis mismatch” is associated with higher rates of death. In some smaller patients with severe calcification of the aortic valve base, aortic root transsection and revision may be needed to place an adequately sized valve and avoid late heart failure symptoms.
Transcatheter Aortic Valve Replacement
The recent development of transcatheter aortic valves has provided an opportunity to improve aortic valve orifice size using a biologic tissue valve mounted on a stent rather than a bulky sewing ring (which reduces orifice size). Initial results in higher-risk older patients (most with degenerative stenosis) suggest that these newer valves have favorable flow characteristics (average EOA 1.7 cm2) and a more laminar flow to the ascending aorta. Early clinical studies show faster regression of hypertrophy and return of systolic function in patients treated with a transcatheter compared to a surgical prosthesis.
Aortic Valve Regurgitation
Because the aortic valve is suspended from the sinus of Valsalva, aortic regurgitation can be caused by structural abnormalities of the aorta and sinus of Valsalva . The most common causes of acute aortic regurgitation are infective endocarditis, a type A dissection of the aorta, and a ruptured aortic valve with cusp prolapse (usually related to preexisting valve malformation such as a bicuspid or fenestrated valve, or blunt trauma) [11, 12]. Chronic aortic valve, regurgitation results from conditions leading to dilation of the aorta or fibrosis and retraction of the valve cusps. Conditions such as Marfan syndrome, ankylosing spondylitis, prolonged hypertension, and aortitis (e.g., syphilis, giant cell arteritis, Takayasu arteritis) lead to chronic, progressive dilation of the aortic “annulus,” lack of cusp coaptation, and, occasionally, diastolic prolapse of an aortic cusp. Valvulitis from radiation, rheumatic fever, autoimmune conditions, and certain drugs (e.g., fenfluramine/phentermine) cause valve fibrosis and, eventually, loss of mechanical valve structure and coaptation.
Aortic regurgitation (also referred to as insufficiency) results in a backflow of blood into the left ventricle during diastole. That causes a rapid increase in diastolic left ventricle pressure. When severe, premature closure of the mitral valve can occur (functional mitral stenosis) or even diastolic mitral regurgitation (i.e., reverse flow through the mitral valve in diastole).
Acute aortic regurgitation is poorly tolerated. Left ventricular diastolic, left atrial, pulmonary venous, and, eventually, pulmonary artery and right ventricular systolic pressures rise quickly. Patients develop rapid onset of heart failure, leading to pulmonary edema. The thin right ventricle (RV) is unprepared for the elevated pulmonary resistance, eventually leading to dilation and failure of the RV, and low cardiac output.
Aortic regurgitation that progresses more slowly leads to adaptive changes in the left ventricle and aorta (◘ Fig. 9.3). The regurgitant volume load that is shuttled between the aorta and ventricle with each cardiac cycle leads to dilation of both structures. The ventricle dilates and develops eccentric hypertrophy. With eccentric hypertrophy, cardiomyocytes lengthen much more than they increase in diameter.




Fig. 9.3
Panel a: Morphological changes in the left ventricle in response to aortic valve regurgitation. The chronic volume overload induces eccentric hypertrophy, a change in ventricular shape from elliptical to spheroid. Panel b: Typical changes associated with progressive aortic valve regurgitation. Untreated, the muscle begins to fibrose, leading to permanent ventricular dysfunction
Dilation of the ventricle greatly reduces myocardial efficiency. The wall stress that must be generated to produce a given intraventricular pressure rises directly with the radius of the ventricular cavity (the law of Laplace). In addition, the shape of the ventricle changes from a prolated ellipse to an almost spherical shape, which also reduces the mechanical advantage of contraction.
As the ventricle dilates, ventricular wall thickness remains relatively constant (usually less than 1.1 cm), but the left ventricle mass increases dramatically. Patients with advanced aortic regurgitation typically have the largest left ventricle mass of any cardiac condition, much larger than that induced by aortic stenosis where wall thickness increases but the left ventricle is much less dilated. As time progresses, a proliferation of fibrous connective tissue develops between the myocytes, causing the ventricular mass to become more fibrotic [13] (◘ Fig. 9.4).


Fig. 9.4
The relationship between ventricular fibrosis and recovery of ventricular function in patients with severe aortic valve regurgitation after valve replacement. Source: Villari et al. Circulation. 2009;120(23):2386–92. Residual myocardial stiffness (upper panel) increases and ejection fraction falls with the fibrotic content of the ventricle
To accommodate the regurgitant volume, the aorta also dilates markedly in patients with aortic regurgitation. Intrinsic aortic disease can augment the degree of dilation for a given regurgitant volume load, as can the presence of concomitant valvular stenosis, where the high-velocity jet ejected across the valve during systole creates high shear stresses on the aortic wall, causing aortic growth.
Assessment of Aortic Regurgitation
Evaluation of aortic regurgitation involves measurement of the amount of the blood regurgitating through the valve and the impact on the left ventricle and other cardiac structures. The initial method for assessing regurgitation was physical examination . A decrescendo diastolic murmur can be heard best at the left sternal border and over the precordium. The magnitude of regurgitation parallels the intensity and duration of the murmur, although in very severe, acute aortic regurgitation, the murmur may stop in mid-diastole due to equilibration of aortic and left ventricle pressure. In chronic aortic regurgitation, the left ventricle is markedly dilated, and the ventricular contraction palpated on the left chest wall is laterally displaced and very broad. In most patients with heart failure, a third heart sound is prominent and a mid-diastolic rumble of partial mitral inflow restriction is heard. The pulse pressure is usually quite wide due to the ejection of a large stroke volume into the aorta (high systolic pressure) and subsequent backflow into the left ventricle (low diastolic pressure).
The second method for assessing aortic regurgitation is injection of X-ray contrast media into the ascending aorta (an aortogram). Regurgitation is classified as 1+ to 4+ using the system described by Amplatz [14].
The extent of aortic regurgitation is most commonly assessed with Doppler echocardiography . While the extent and location of regurgitation can be visualized on color Doppler examination, certain parameters are useful in quantitating the magnitude of regurgitation (◘ Table 9.1). Echocardiography enables quantifying the degree of regurgitation and visualizing the impact of aortic regurgitation on cardiac size and function, as well as pulmonary artery pressure.
Table 9.1
Parameters of chronic aortic regurgitation
Mild AR | Moderate AR | Severe AR | Advanced AR | |
---|---|---|---|---|
LV ejection fraction | Normal | Normal-↓ | ↓-↓↓ | ↓↓-↓↓↓ |
LV size | Normal-↑ | ↑-↑↑ | ↑↑↑-↑↑↑↑ | ↑↑↑↑ |
LA size | Normal | ↑-↑↑ | ↑↑-↑↑↑ | ↑↑-↑↑↑ |
Cardiac output | Normal | Normal | Normal to ↓ | ↓-↓↓ |
PA pressure | Normal | Normal-↑ | ↑-↑↑ | ↑↑ |
Angiographic grade | 1+ | 2–3+ | 3–4+ | 3–4+ |
Regurgitant volume (mL) | <20 | 20–60 | >60 | >60 |
Regurgitant fraction (%) | <15 | 15–50 | >50 | >50 |
Effective regurgitant orifice (cm2) | <0.2 | <0.4 | >0.4 | >0.4 |
The degree and chronicity of regurgitation can be inferred from the structural changes to the heart. Acute regurgitation results in little change to ventricular volumes, but marked changes in function. These include premature closure of the mitral valve (and a corresponding Austin Flint diastolic murmur) and elevated pulmonary artery pressure. Chronic aortic regurgitation leads to the dilation and loss of systolic contraction described above. Acute or rapidly worsening aortic regurgitation and structural changes should prompt early surgical correction.
Medical Therapy of Aortic Regurgitation
Reduction of aortic pressure and afterload lessens the amount of regurgitation. Afterload reduction with nitroprusside can lead to a marked and rapid temporary improvement in acute aortic regurgitation, but is not practical for chronic treatment.
Angiotensin-converting enzyme (ACE) inhibitors, hydralazine, and other agents that reduce systemic vascular resistance can provide symptomatic relief in some patients with chronic aortic regurgitation [15]. Treatment with ACE inhibitors does not reduce the progression of ventricular dilation in patients with mild to moderate aortic regurgitation. Overall, medical therapy with afterload reduction for chronic aortic regurgitation has proven disappointing [16, 17]. For symptomatic patients, vasodilator therapy is generally relegated to a stabilizing role prior to valve replacement.
Beta-receptor antagonists can be used to prevent the decline in systolic ventricular function that occurs later in the disease and may improve survival [18].
Surgical Treatment
The primary surgical therapy is replacement of the aortic valve with a prosthetic valve. For most patients with aortic regurgitation, the aorta is also dilated, and surgery may involve replacing the dilated aortic root using a composite graft and reimplanting the coronary arteries. Some patients may benefit from a reconstruction of the valve and root [19], which has the potential advantage of a more durable result [20].
After valve replacement, the left ventricle slowly falls in size and transforms back toward a prolated ellipse [21]. Systolic contraction improves, but usually does not return to normal. Cardiomyocyte hypertrophy nearly normalizes but the fibrosis that characterizes advanced regurgitation does not recede. The result is persistent reduction of ventricular compliance due to reduced ventricular elasticity. The improvement in systolic function after valve replacement is inversely proportional to the degree of fibrosis at the time of valve replacement.
The lack of full ventricular recovery after aortic valve replacement for regurgitation has led many experts to recommend early valve replacement to avoid irreversible changes to ventricular structure. In most patients, valve replacement is indicated when the ventricle dilates significantly, or the ejection fraction falls. An end-systolic dimension >50 mm and a left ventricular ejection fraction <50 % are commonly accepted triggers for valve surgery. In patients without symptoms and/or with preserved left ventricular systolic pressure, marked dilation (end-diastolic dimension >65 mm) may also trigger surgical intervention.
Transcatheter valve replacement can be accomplished in some patients with pure aortic regurgitation and might be appropriate for a fraction of patients. One valve is approved for deployment in regurgitant aortic valves (JenaValve). This valve uses retention clips that prevent migration of the valve to the ventricle. The inability to treat the associated aortic dilation, the large size of the prosthetic valves required, and the inability to debride infected valves limits it applicability to present transcatheter approaches .
The Mitral Valve
The two mitral valve leaflets are normally supported by the mitral annulus at their bases and by chordae tendineae along the leaflet coaptation edges [22, 23]. The chordae are attached to one of two papillary muscles. The papillary muscles arise from the anterolateral and posterolateral ventricular myocardium, about two-thirds of the way down from the ventricular base to its apex.
During ventricular contraction , the rising pressure in the ventricle pushes the mitral leaflets toward the low-pressure atrium like a parachute. The mitral annulus contracts somewhat. The chordae tighten as the leaflet edges move toward the atrium and the attached papillary muscles and their underlying myocardium contract. This interaction limits mitral travel beyond the leaflet coaptation point (i.e., prevents valve prolapse) and improves ventricular contraction by giving the ventricle a tether to pull on to shorten. Hence, not only do the chordae tether the mitral leaflets, they also tether the ventricle and improve ventricular function. This interaction between the annulus, chordae, and ventricle improves mitral valve closure and left ventricular systolic performance. Preservation of the chordae apparatus during surgical replacement of the mitral valve results in better long-term ventricular function after surgery.
The normal mitral valve has a significant amount of redundant tissue—about twice that of the mitral cross-sectional area. In addition, the fibrous annulus supporting the base provides considerable structural support, as opposed to the aortic valve, which has no true annulus. This is needed because the mitral orifice is roughly two times that of the aortic cross-sectional area. In cattle, where the mitral annulus is even larger, mature cows actually ossify a portion of the annulus to bone, presumably to provide adequate support to the large valve circumference.
Functional Mitral Regurgitation
Functional mitral regurgitation refers to abnormal mitral valve function, predominantly regurgitation, due to changes in the structures surrounding and supporting the mitral valve [24]. Many cardiomyopathies caused by intrinsic cardiomyocyte disease (such as viral, inflammatory, or some genetic cardiomyopathies) and the more prevalent ischemic cardiomyopathies eventually lead to ventricular dilation and fibrosis.
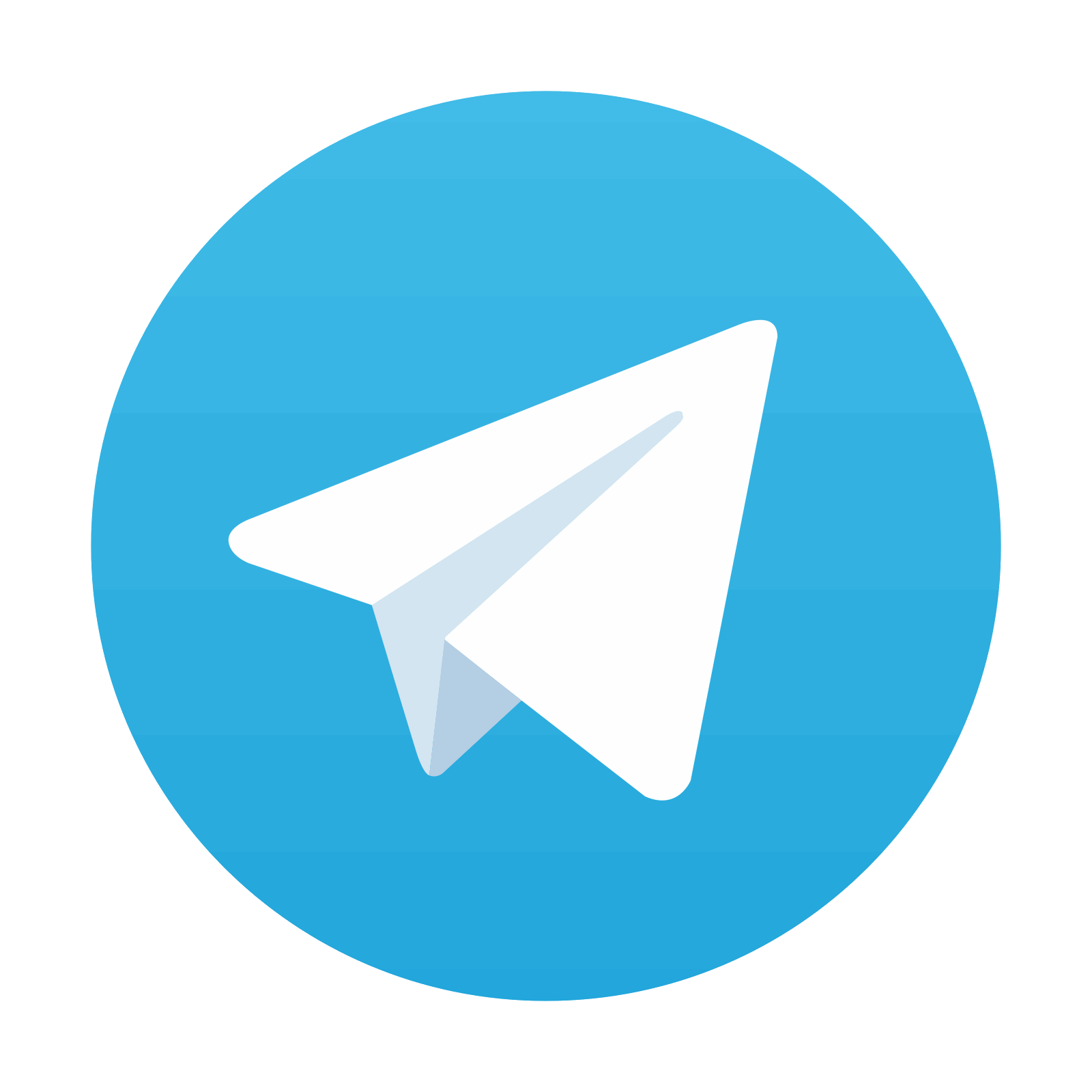
Stay updated, free articles. Join our Telegram channel

Full access? Get Clinical Tree
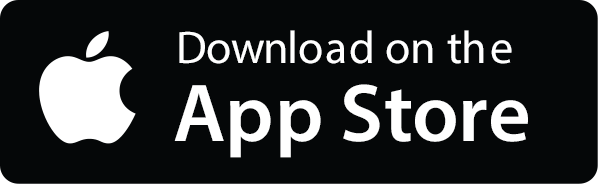
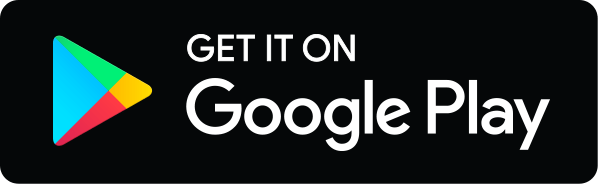