We investigated the clinical value of three-dimensional (3D) transesophageal echocardiography (TEE) color flow Doppler (TEE-CFD) for percutaneous transcatheter closure of mitral valve prosthesis paravalvular leaks (PVLs) compared to two-dimensional (2D) TEE. The number, location, and size of the mitral valve prosthesis PVLs were determined in 8 patients using 2D and 3D TEE-CFD. We also evaluated 2D and 3D TEE-CFD for identifying the canalization of the target PVL during the intervention and assessing the change in the PVL effective orifice after the endovascular procedure. We visualized 12 PVLs using 2D TEE-CFD and 15 PVLs using 3D TEE-CFD. No substantial disagreement was found between 2D and 3D TEE-CFD for the location for each of the PVLs. No difference was found in the vena contracta short axis width obtained by 2D TEE-CFD and 3D TEE-CFD (5.7 ± 1.4 mm vs 5.5 ± 1.3 mm, respectively, p = 0.09). However, only 3D TEE-CFD demonstrated the effective circumferential orifice length of the PVL (12.2 ± 8.5 mm). A closure device was deployed in 6 cases. In 1 case, the canalization of a nontarget PVL, visualized only on 3D TEE-CFD, led to an appropriate change in the treatment strategy. The reduction in the mean PVL vena contracta width demonstrated using 2D TEE-CFD and 3D TEE-CFD was similar (2.2 ± 0.7 mm vs 2.1 ± 1.1 mm, respectively, p = 0.69). However, only 3D TEE-CFD verified the reduction of the effective orifice circumferential length of the PVL by 10.5 ± 5.6 mm. In conclusion, 3D TEE-CFD provided unique and additive information in patients with mitral valve prosthesis PVLs. This new technology has the potential to improve the procedural success of percutaneous transcatheter closure of PVLs.
A recently introduced technology for real-time 3-dimensional (3D) transesophageal echocardiography (TEE) has provided unlimited image plane orientation and unique en face visualization of paravalvular defects ( Figure 1 ). In addition 3D-CFD enables direct visualization and qualitative assessment of the mitral valve effective regurgitant orifice. Although Kronzon et al recently reported the use of real-time 3D TEE for the evaluation of postoperative mitral annuloplasty ring and prosthetic valve dehiscence, no direct comparison between conventional 2D-TEE color flow Doppler (2D TEE-CFD) and 3D TEE-CFD has been reported for the assessment and endovascular treatment of patients with mitral valve prosthesis paravalvular leaks (PVLs). We hypothesized that this new 3D TEE-CFD technology would have additive value for transcatheter closure of mitral valve prosthesis PVLs. Therefore, the objective of our study was to compare 2D and 3D TEE-CFD in the planning, guiding, and evaluation of transcatheter closure of mitral valve prosthesis PVLs.
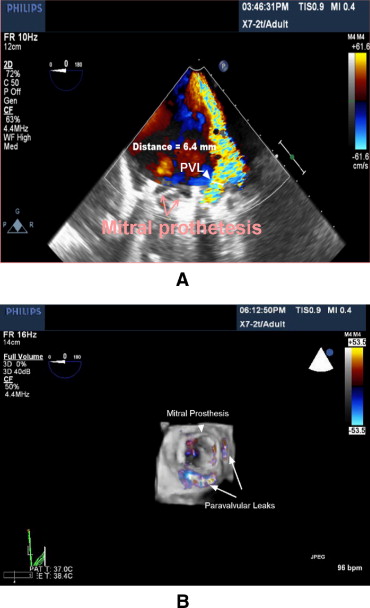
Methods
From April 2008 to February 2009, 8 consecutive patients were referred because of advanced congestive heart failure and/or hemolytic anemia associated with severe mitral valve prosthesis PVL. These patients were deemed to be at high operative risk. Therefore, the focus of the assessment was to determine the appropriateness of transcatheter PVL closure.
All imaging modalities were performed with the iE33 ultrasound system (Phillips Medical Systems, Andover, Massachusetts) equipped with a fully sampled 3D matrix array TEE transducer.
The initial assessment of the PVL was performed by routine 2D TEE-CFD using previously described methods. The spatial extension of the paravalvular jet was evaluated in the standard mid-esophageal view. The sewing ring was fully screened by 180° rotation of the imaging plane using consecutive 10° increments.
The 3D TEE CFD images were obtained by acquiring electrocardiographic-triggered images. This tool acquires 7 sequential, thin-scan volumes and creates a pyramid-shaped full volume. If it was not possible to visualize the entire circumference of the prosthetic ring and all paravalvular jets in a single 3D-CFD full volume image, 2 volumes of the medial and lateral sections of the sewing ring and adjacent paravalvular regions were acquired separately, with some overlap.
The utility of 2D TEE-CFD and 3D TEE-CFD was assessed in 8 patients using screening echocardiography before transcatheter intervention. The aim of the evaluation was to determine 3 parameters: (1) the total number of PVLs, with particular emphasis on identification of the target lesion for intervention, (2) the location of the PVLs, and (3) the size of the PVL effective orifices.
To facilitate communication between the invasive cardiologists and echocardiographic cardiologists and to enhance integration of new technology in the procedural environment, preprocedural 2D-TEE and 3D-TEE images were collectively reviewed by both subspecialists off-line. All measurements were performed using an image management system (Vericis, Emageon, Birmingham, Alabama). The criteria for the identification of PVLs using 2D and 3D TEE-CFD required clear visualization of the PVL origin and the regurgitant jet extending into the left atrium. To distinguish between a single leak and multiple isolated leaks originating from the same paravalvular region, it was necessary to demonstrate the presence of paravalvular tissue separating 2 adjacent PVLs.
The location of the paravalvular lesions was identified using a previously published diagram that related the mitral valve prosthesis and 2D transesophageal echocardiographic image plane angle orientation ( Figure 2 ). The clock face of the sewing ring was divided into 6 circumferential regions of 60° (or 2 hours) each (ie, 12 to 2 o’clock, 2 to 4 o’clock, and so forth). The mitral valve anatomy was oriented in the surgical perspective by flipping the diagram around a vertical axis and rotating the image counterclockwise to bring the aortic valve to the 12-o’clock position ( Figure 2 ). The 3D TEE-CFD data set was also oriented to the surgical view for visualization of the mitral anatomy ( Figure 3 ). The locations of all regurgitant defects were then separately plotted on the reference diagram for each modality ( Figure 1 ).
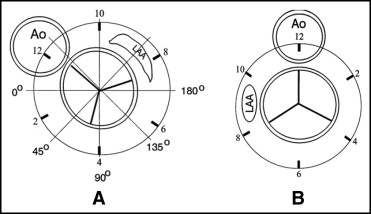
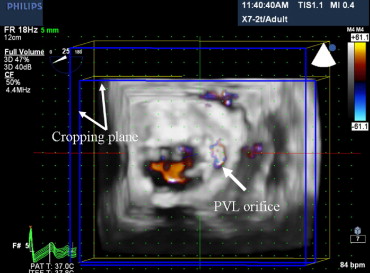
The vena contracta width was obtained on 2D TEE-CFD images ( Figure 1 ). The 3D images were oriented to the en face surgical view of the mitral valve from the left atrial perspective for the quantitative assessments. The 3D TEE-CFD data were manually cropped to visualize the effective orifice of the PVL ( Figure 3 ). The cropping plane was oriented perpendicularly to the jet direction and was moved from the left atrium toward the mitral annulus until the smallest jet cross-sectional area was obtained. This was defined as the effective regurgitant orifice at the level of the vena contracta, which was visualized approximately 5 mm above the defect. The distance measurement tool was then calibrated using calibration markings on the 3D TEE-CFD image grid, and the width and circumferential length of the PVL effective orifices were then obtained ( Figure 4 ). We compared the vena contracta width and the location of the effective orifice using 2D TEE-CFD and 3D TEE-CFD before the procedure. In addition, we assessed the magnitude of the postprocedural changes of the PVL effective orifices using 2D and 3D TEE-CFD.
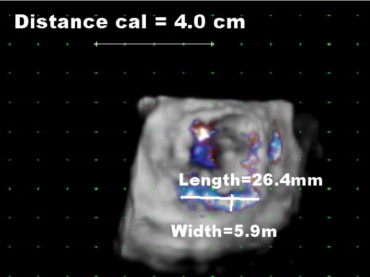
The utility of 3D TEE-CFD for endovascular closure of a PVL was evaluated during intraprocedural echocardiography in 6 of 8 patients who underwent intervention. In each patient, closure of the largest PVL was performed if multiple leaks were present. Two patients ( Table 1 , patients 2 and 5) were not suitable for transcatheter intervention: one because of coexistent mitral valve vegetation and the second because of extensive dehiscence of approximately 1/3 of the circumference of the mitral valve prosthesis sewing ring. Because the focus of the present study was to evaluate the usefulness of 3D TEE-CFD to identify proper canalization of the target PVL, 2D TEE-CFD and 3D TEE-CFD images were obtained after advancement of the guiding catheter across the PVL.
Pt. No. | PVL | 2D Vena Contracta | 3D Effective Orifice | ||||||
---|---|---|---|---|---|---|---|---|---|
Clock Face Location | Dimension (mm) | Clock Face Location | Width (mm) | Length (mm) | |||||
Before | After | Before | After | Before | After | ||||
1 | |||||||||
1 | 1–3 | 6 | 3 | 1–2 | 6 | 2 | 10 | 2 | |
2 | 8–11 | 5 | 10–11 | 5 | 6 | ||||
3 | 9–10 | 2 | 3 | ||||||
4 | 8–9 | 3 | 6 | ||||||
2 | |||||||||
1 | 2–6 | 8 | 3–6 | 8 | 27 | ||||
3 | |||||||||
1 | 6–7 | 7 | 6 | 6–9 | 6 | 5 | 27 | 7 | |
2 | 2–5 | 5 | 3–5 | 5 | 10 | ||||
4 | |||||||||
1 | 12–4 | 6 | 4 | 12–2 | 6 | 4 | 17 | 6 | |
2 | 2–4 | 3 | 6 | ||||||
5 | |||||||||
1 | 4–7 | 8 | 4–7 | 7 | 23 | ||||
6 | |||||||||
1 | 1–3 | 5 | 3 | 1–3 | 6 | 3 | 22 | 7 | |
2 | 8–9 | 5 | 8–9 | 5 | 6 | ||||
7 | |||||||||
1 | 3–6 | 5 | 4 | 4–6 | 5 | 4 | 5 | 3 | |
8 | |||||||||
1 | 5–8 | 6 | 3 | 5–8 | 5 | 2 | 10 | 3 | |
2 | 2–3 | 3 | 3–4 | 3 | 5 |
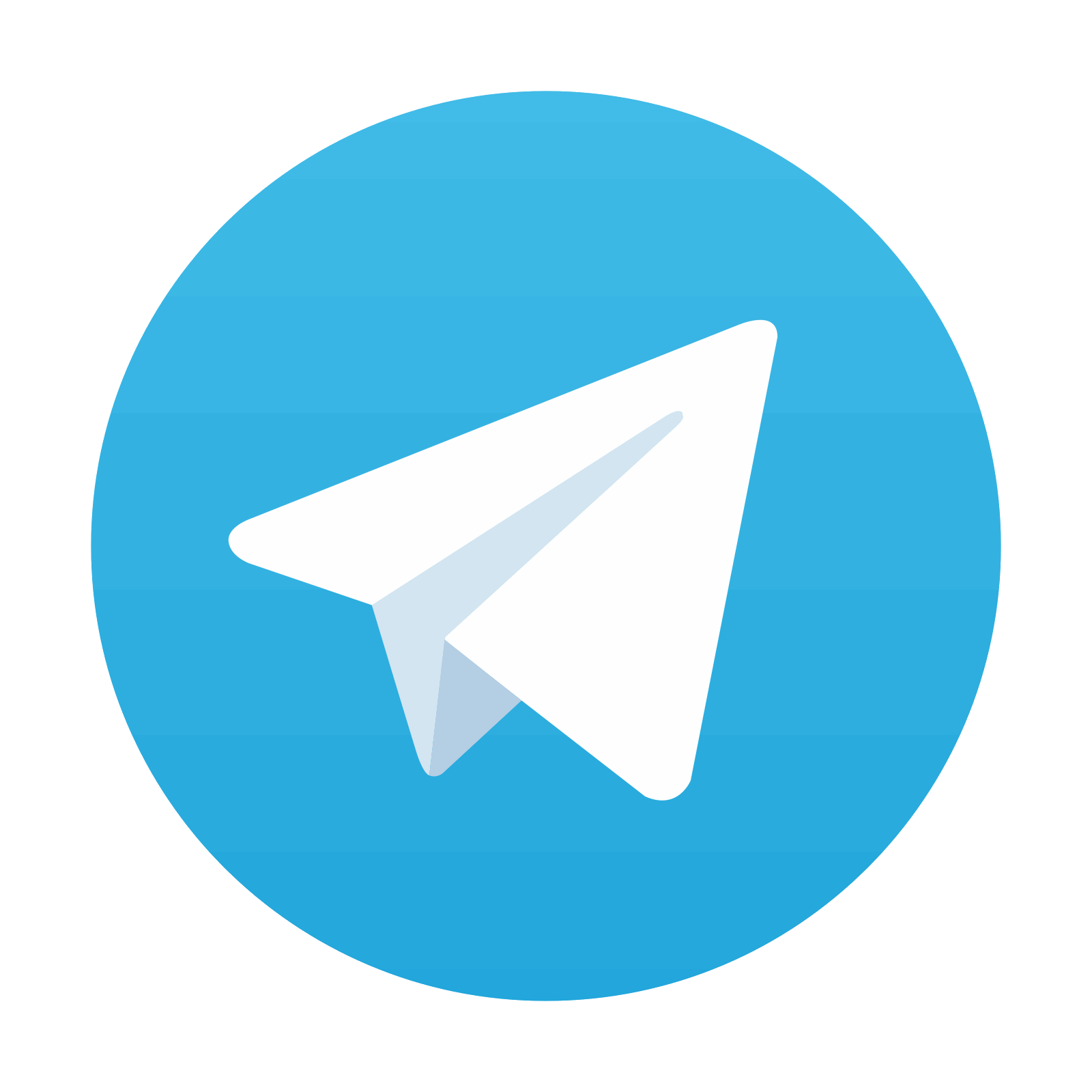
Stay updated, free articles. Join our Telegram channel

Full access? Get Clinical Tree
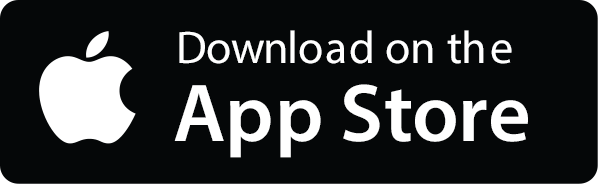
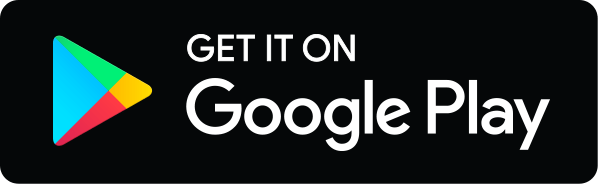
