, Rohit Arora3, 4, Nicholas L. DePace5 and Aaron I. Vinik6
(1)
Autonomic Laboratory Department of Cardiology, Drexel University College of Medicine, Philadelphia, PA, USA
(2)
ANSAR Medical Technologies, Inc., Philadelphia, PA, USA
(3)
Department of Medicine, Captain James A. Lovell Federal Health Care Center, North Chicago, IL, USA
(4)
Department of Cardiology, The Chicago Medical School, North Chicago, IL, USA
(5)
Department of Cardiology, Hahnemann Hospital Drexel University College of Medicine, Philadelphia, PA, USA
(6)
Department of Medicine, Eastern Virginia Medical School Strelitz Diabetes Research Center, Norfolk, VA, USA
Validation
In academic review articles describing morbidity [1] and mortality [2] risk in patients with diabetes mellitus, Vinik et al. include P&S monitoring in their list of “commercially available computer programs … employed to assess autonomic nerve function.” From an autonomic perspective, the cascade of secondary symptoms typically attributed to diabetes as defined by Vinik et al. and the many cardiovascular risk factors as discussed by DePace et al. [3, 4] are effects of progressively accelerating autonomic dysfunction. This has led DePace et al., in another academic review article, to conclude that “It is unequivocally established that CAN is associated with increased cardiac morbidity and mortality. Identifying and addressing CAN early, especially in a subclinical cardiac patient, will further differentiate which asymptomatic patients require more aggressive therapy. The results from P&S testing documenting CAN may be used as a baseline. One should view these test results as a guide towards more individualized treatment. A more specific selection of medications and dosing based on these results is possible. P&S test results represent objective data which are useful in guiding pharmacological and lifestyle changes. In addition to normalization and improvement of CAN independent, simultaneous P&S testing providing unambiguous parasympathetic and sympathetic activity levels, may guide the physician towards the type and dosing of pharmacological agents necessary to achieve an objective clinical target or outcome. The pharmacopeia includes adrenergic (beta-blockers, anti-hypertensives, bronchodilators and vasopressors) and cholinergic, (anti-depressants, anxiolytics, and anti-psychotics) agents. This would eliminate arbitrarily dosing medications without a clear target outside of HR and BP. Also, the threshold for implanting of prophylactic devices such as cardiac defibrillators may be better defined by assessing and following P&S dysfunction. While further studies are indicated, the clinical and epidemiological data is too compelling not to test for, diagnose, and aggressively treat CAN with abnormal SB to guard the patient’s well being, not only in diabetics, but in all patients with risk factors for heart disease.”
A 2008 vasopressor outcome study [5] compares HRV-alone analyses (both time and frequency domain) with P&S monitoring and demonstrated a greater ability in describing patient outcomes than any of the HRV-alone results. In 184 consecutive, arrhythmia-free, insulin-dependent diabetic patients with previously diagnosed orthostatic hypotension, with persistent dizziness, P&S monitoring demonstrated persistent autonomic dysfunction (sympathetic withdrawal upon standing) and more accurately described the known hemodynamic effects pre- and post-dosing with an alpha-1 adrenergic agonist. Arora et al. conclude, “independent, simultaneous assessment of progressive parasympathetic and sympathetic dysfunction, autonomic imbalance, and the responses of the two ANS branches to therapy enables early detection and early intervention. Orthostatic dysfunction, by way of example, illustrates that frequent, sensitive assessments of both ANS branches can improve the negative outcomes associated with CAN.”
A recently concluded study compares P&S monitoring to HRV-alone monitoring in 2,745 patients (41.5 % female) diagnosed with autonomic neuropathy. The results from both monitoring methods are correlated with: known normals, symptoms of advanced autonomic dysfunction (or DAN in diabetics), symptoms of CAN, age, body mass index (BMI), stage of autonomic decline, and level of autonomic risk as defined by P&S monitoring, HRV-alone monitoring, resting HR, resting BP, and clinical trending. All measures are well correlated (p < 0.05) with age, BMI, resting HR, and resting BP. While different HRV-alone parameters are individually well correlated with known normals (E/I ratio, p = 0.040), symptoms of advanced autonomic dysfunction or DAN (LFnu and HFnu, p < 0.050), symptoms of CAN (sdNN, p = 0.050), stage of autonomic decline (sdNN, p = 0.089), and level of risk (TSP, p = 0.079), there is a lack of consistency. All P&S monitoring parameters are well correlated with all factors (p < 0.020). In measures of specificity, P&S monitoring consistently demonstrates significantly greater positive and negative predictive rates with fewer false-positive and false-negative rates.
Another recently concluded study compares P&S monitoring to HRV-alone monitoring in 2,745 patients (41.5 % female) diagnosed with autonomic neuropathy, including treatment for orthostatic dysfunction. The results from both monitoring methods are correlated with the prevalence of orthostatic dysfunction, including orthostatic hypotension (OH, an abnormal drop in BP upon standing), postural orthostatic tachycardia syndrome (POTS, an excessive increase in HR upon standing), postural orthostatic bradycardia syndrome (POTS, an abnormal decrease in HR upon standing), and postural orthostatic hypertension syndrome (POHS, an excessive increase in BP upon standing). For comparison, orthostatic dysfunction is defined both in terms of hemodynamics alone and hemodynamics with autonomic function (sympathetic withdrawal or SW). Total orthostatic dysfunction patients (2,408) are more than the total SW patients (1,127). The discrepancy is because not all patients are neurogenic; some have only vascular dysfunction. Assuming any decrease in BP with upright posture is abnormal, 2,175 patients demonstrate a decrease in BP of more than 5/0 mmHg (clinical and preclinical OH). The P&S method identifies 71.9 % with a false-negative and false-positive rate of 12.3 and 10.1 %, respectively (p = 0.0076). The HRV-alone method identifies 22.3 % with a false-negative and false-positive rate of 26.8 and 21.4 %, respectively (p = 0.0144). POTS is diagnosed in 0.9 % of the patients. The P&S method identifies 48.0 % with a false-negative and false-positive rate of 1.5 and 0.3 %, respectively (p = 0.0187). The HRV-alone method identifies 28.0 % with a false-negative and false-positive rate of 2.6 and 0.4 %, respectively (p = 0.0838). POBS is diagnosed in 3.5% of the patients. The P&S method identifies 45.4 % with a false-negative and false-positive rate of 6.3 and 0.04 %, respectively (p = 0.0251). The HRV-alone method does not identify any POBS. POHS is diagnosed in 4.2 % of the patients. The P&S method identifies 70.0 % with a false-negative and false-positive rate of 21.6 and 0.5 %, respectively (p = 0.0036). The HRV-alone method identifies 11.8 % with a false-negative and false-positive rate of 64.1 and 2.8 %, respectively (p = 0.0853).
Akselrod and the original researchers from MIT and Harvard validated P&S monitoring and its applications throughout many fields of medicine [6–38]. These articles demonstrate specificity, sensitivity, reliability, and repeatability of the method. The HRV standard articles [39, 40] reference the MIT/Harvard work, recognizing its validity. The CPT code 95943 is written based on the MIT/Harvard method.
P&S Monitoring Versus HRV Alone: Deep Breathing
Differences between the P&S monitoring and HRV-alone monitoring are highlighted in the patient example below (adapted from Aysin and Aysin [41]). A slice of the time-frequency analysis of DB is presented in Fig. 4.1. The upper left graph in Fig. 4.1 is the cardiogram (instantaneous HR plot) in response to six slow, relaxed, deep breaths over 60 s. The upper right plot is the corresponding wavelet analysis results with time on the abscissa, frequency on the ordinate, and frequency (or spectral) amplitude in gray scale (black is zero and white is maximum). The second row presents similar plots for the RA and corresponding wavelet analysis. At each time slice in the RA time-frequency spectrum (a sample is presented in the third row of Fig. 4.1), the FRF is determined and transferred to the HR spectrum (the corresponding sample is presented in the bottom row of Fig. 4.1). Given the FRF, the RFa area is determined (again, the area under the HR spectral curve within the parasympathetic activity window [42], represented in blue, centered on the FRF). The remaining portion of the LF region then represents sympathetic activity. For comparison, the LF and HF regions [39, 40] are also represented on the HR spectrum plot in this example. The apparent significant discrepancies are tabulated; see Table 4.2 [41 (Table 1)]. The region corresponding to parasympathetic modulation (RFa) is missed in HRV-alone analysis. Of course, the isolation of P from S in this example is clear and easy for illustrative purposes. However, in typical, clinical situations, the discrepancies are often more subtle and are the reason for much of the lack of general clinical acceptance.


Fig. 4.1
A comparison of patients’ deep breathing responses as assessed with P&S monitoring and HRV-alone methods
Table 4.1
Comparison of mean and standard deviation of low and high frequency power of HRV data estimated from traditional HRV (tHRV) and eHRV analysis over 17 patients
tHRVa | eHRV | p | |||
---|---|---|---|---|---|
NB | LF | 4.46 ± 3.18 | LFa | 2.66 ± 1.85 | 0.0003b |
HF | 3.08 ± 2.03 | RFa | 2.72 ± 1.81 | 0.0059b | |
LF/HF | 1.58 ± 0.71 | LFa/RFa | 1.12 ± 0.55 | 0.0004b | |
DB | LF | 50.66 ± 33.59 | LFa | 2.07 ± 2.32 | 0.0003b |
HF | 6.94 ± 6.12 | RFa | 46.11 ± 30.15 | 0.0003b | |
LF/HF | 9.22 ± 3.64 | LFa/RFa | 0.11 ± 0.26 | 0.0003b |
Table 4.2
Comparison of different HRV analysis methods during orthostatic challenge
Method | Variable | Baseline | Standing | p |
---|---|---|---|---|
Hemodynamics | HR | 76 ± 11 | 87 ± 13 | <0.005 |
MAP | 84.35 ± 9.52 | 88.03 ± 9.37 | 0.012 | |
P&S monitoring | LFa | 3.95 ± 3.51 | 6.04 ± 5.91 | 0.023 |
RFa | 5.28 ± 7.23 | 2.66 ± 4.18 | <0.005 | |
LFa/RFa | 1.56 ± 2.36 | 4.92 ± 7.04 | <0.005 | |
HRV-alone frequency domain analysis | LF | 7.15 ± 7. 22 | 8.69 ± 8.52 | 0.242 |
HF | 4.88 ± 5.62 | 2.32 ± 2.12 | <0.005 | |
LF/HF | 1.99 ± 1.47 | 4.84 ± 4.20 | <0.005 | |
HRV-alone time domain analysis | sdNN | 48.04 ± 23 | 46.47 ± 20.60 | 0.747 |
rmsSD | 35.25 ± 21.84 | 21.06 ± 10.76 | <0.005 | |
pNN50 | 14.97 ± 16.62 | 4.52 ± 6.27 | <0.005 | |
HRV-alone statistical analysis | Range HR | 25 ± 10 | 33 ± 13 | <0.005 |
The results from patients (Table 4.2) show that the HRV-alone method (tHRV) is neither sufficient nor accurate for assessing P&S activity. Table 4.2 data show that during normal breathing (NB), the HF measure is poorly associated (p > 0.050). The data also show that during deep breathing (DB), the HRV-alone LF term increases significantly, suggesting that the LF term captures the expected parasympathetic response. Typically, this would be interpreted as a sympathetic response, whereas the RFa term (from P&S monitoring) captures the parasympathetic activity. Aysin and Aysin [41] conclude, “it has been well established that HRV analysis with and without respiratory [activity] information provides different results” (citing Triedman, Perrott, Cohen, and Saul [14]). Aysin and Aysin continue by stating “Although the differences were more obvious with low breathing rates, using [the P&S method] in general would provide more accurate results, due to accurate localization of parasympathetic activity in the HRV signal” [41].
P&S Monitoring Versus HRV Alone: Postural Change
Aysin et al. also compare the HRV-alone and P&S monitoring methods during orthostatic challenge [43]. In this study, Aysin et al. included in the comparison both spectral analysis and time domain ratio measures from HRV alone (see Table 4.1). One hundred healthy volunteers participated in this study. The methods included 5 min of rest, in a relaxed seated position with sturdy back support, and then a quick PC to standing, followed by 5 min of quiet standing. As documented in Bloomfield et al. [44], this active standing maneuver results in P&S responses that are “nearly identical to the changes in autonomic balance that occurs during the first 5 min of quiet standing.” Their results suggest “nearly identical” is approximately 96.3 %. These results were repeated, using hemodynamic measures as biomarkers [45].
As expected, Aysin et al. [43] find a statistically significant decrease in the parasympathetic activity as measured by P&S monitoring (RFa, p < 0.005) when the patient assumes a head-up posture. A similar result is also found with the HRV-alone measures that are considered to represent parasympathetic activity (i.e., HF, rmsSD, pNN50; p < 0.005). The P&S monitoring measure, SB (=LFa/RFa), and the HRV-alone measure, LF/HF, significantly increase from baseline to standing (p < 0.005), as does the P&S monitoring sympathetic activity measure (LFa, p = 0.023). However, the changes in the HRV-alone measures, which are assumed to represent sympathetic activity (sdNN and LF), were not statistically significant (p > 0.050). Aysin et al. observe that this “result is important in that significant changes in sympathetic activity is properly identified only by using HRV Spectral analysis with RA analysis [the P&S method]. Although sympathetic activity is not directly related to respiration, parasympathetic activity is, as its measure is computed based on respiration. Therefore, using RA analysis in HRV analysis should make a difference. The reason that LF was not significantly different between baseline and stand is explained by the fact that the LF area includes both P and S modulations resulting from varying respiratory rates. If an individual has low respiratory rates, Vagal (parasympathetic) activity will contribute to the LF area and power in LF area will be mixed. Therefore, change in sympathetic activity from sitting (baseline) to stand will not be reflected properly. In clinical settings, detection of significant changes is very important in determining treatment [43]. Aysin et al. [43] conclude that “even under normal breathing rates, [P&S Monitoring] seems to provide the most accurate estimates of both ANS branches, and therefore would be a better clinical tool in diagnosing and treating medical conditions resulting from autonomic dysfunction.”
Aysin and Aysin [41] also presented a second example with a breathing rate that is >0.15 Hz and satisfies the HRV-alone spectral analysis criterion for respiratory frequency [39, 40]. In this example (see Fig. 4.2), the respiratory frequency while the subject is standing is 0.26 Hz (15.6 breaths per minute). The top two plots in Fig. 4.2 present the independently and simultaneously captured HBI (cardiogram) and respiratory activity (“RA”) signals, respectively. A higher respiratory rate is obvious and reflected in the RSA in the cardiogram (HR plot, top). The next two plots are the HR and RA time-frequency results from the two independent and simultaneous wavelet analyses, respectively. The higher frequency content in both signals is apparent. The bottom two plots are single slices from the RA and HR spectra, respectively. As depicted, the FRF is detected from the RA spectrum and transferred to the HR spectrum. Then from the HR spectrum, parasympathetic activity is computed from the area under the HR spectrum within the blue-shaded area (RFa). Notice this RFa is completely contained within the HF. The more narrow RFa is a more accurate parasympathetic measure than the broader HF measure. From Figs. 4.1 and 4.2, both RFa and LFa computations result in an increased specificity over the HRV-alone methodology.


Fig. 4.2
P&S monitoring analysis and results from a healthy subject in response to a PC challenge
P&S assessment defines independent, simultaneous measure of P and S activity. So what?!? Examples will be demonstrated throughout the rest of this book. In general, independent, simultaneous assessment of P&S activity enables physicians to quantify and document patients’ responses to therapy, disease, lifestyle, and history. It also simultaneously measures parasympathetic activity (RFa) and sympathetic activity (LFa) without extraneous signal content (noise). Specific, independent, simultaneous measures of P&S activity enable a true measure of autonomic balance (SB = LFa/RFa). Wavelets enable P&S measures for arrhythmia patients through the ability to analyze the transient nature of arrhythmia [46]. Wavelet spectral analysis resolves the shortcomings of the Fourier transform (FFT), enabling customized and individualized interventions. The results are reduced morbidity and mortality, reduced medication load and hospitalizations and reduced healthcare costs, and improved patient outcomes.
P&S Monitoring Versus HRV Alone: Clinical Example
In HRV-alone, the Parasympathetic response is often lost in the Low Frequency measure, inflating the assumed Sympathetic Response, masking orthostatic dysfunction, and causing false positive indications of Sympathetic Excess.
The next section is composed of excerpts from a manuscript first published as an abstract entitled “Enhanced frequency domain analysis replaces older heart rate variability methods.” The abstract was accepted at the Fourth Annual Diabetes Technology Meeting, Philadelphia, PA, 28–30 October 2004, and presented as a poster [47]. This manuscript details the differences between P&S monitoring and HRV alone for DB and Valsalva over the adult age range. The original data (as presented below) are based on a cohort of 389 patients (161 females, 354 type 2 diabetics and 35 type 1 diabetics). This is a subpopulation from a patient cohort of 511 patients diagnosed with type 2 diabetes (248 females, age = 63.4 ± 13.1, range 25–96) [48].
Background
P&S monitoring completes older HRV-alone methodologies to allow proper dissection of P from S activity based on HRV-alone analysis, which provides more information, to further differential diagnostics and quantify individual patient response to therapy. Poor P&S results have been correlated with poor outcomes, and normal P&S results have been correlated with healthy outcomes [49, 50]. For example, orthostatic hypotension (OH) is common in diabetes and perhaps the most debilitating comorbidity [1, 2]. OH is a failure of the SNS, but it could also be (partially) caused by an overdrive of the PSNS. Either way it is not an ANS structural deficit, it is a functional deficit. Identifying this difference facilitates diagnosis and therapy.
Methods
Two or more autonomic (P&S monitoring) assessments were performed on 389 adults diagnosed with type 2 diabetes. The average age of the cohort is 63.2 (range is from 25 to 96), with 161 females (41.4 %). See Chap. 5 of this compendium for a complete methodology describing the autonomic (P and S) assessment study. The Autonomic (P and S) Assessment section includes a copy of the original figure, depicting a model of the P&S monitoring method. P&S monitoring reproducibility studies resulted in 11.4 % ± 1.3 variability from test to test within a short period of time (<6 weeks). From these data, the patient’s HRV-alone (LF, HF, and L/H) and P&S monitoring (LFa, RFa, and LFa/RFa) parameters were computed in response to clinical challenges and at rest. Patients presenting with SW (suggesting section “Orthostatic dysfunction) were analyzed by considering L/H and LFa. According to the standard articles [39, 40], the HRV-alone parameter L/H is considered a better representative of sympathetic response to upright posture. Also, patients presenting with stand PE were analyzed by considering HF and RFa.
Results
Of the 389 patients, 43.7 % presented with clinical OH symptoms, with positive studies for vascular abnormalities, including “lazy walls” and dysfunctional valves. Another 67.3 % presented with clinical symptoms of PE. Comparisons between HRV-alone parameters and P&S monitoring parameters from OH patients showed that LFa positively identified more patients than L/H (73 % vs. 29 %, p < 0.001). Similar comparisons between PE patients showed that RFa positively identified more patients than HF (94 % vs. 18 %, p < 0.001). More on OH will be said in an outcome study [5] presented later in this compendium (see section “Orthostatic dysfunction”).
The resting (baseline) P&S monitoring results are plotted in Fig. 4.3. The thicker solid lines with squares represent the resting sympathetic activity (Bx LFa), and those with asterisks represent the initial baseline (resting) parasympathetic activity (Bx RFa). The normal ranges for both resting parameters are 1.0–10.0 bpm2, with a borderline region from 0.5 to 1.0 bpm2. In the case of this cohort, the test 1 results (the left hand series of data points in Fig. 4.3: LFa = 1.01 bpm2 and RFa = 0.97 bpm2) are near the low end of the normal range. Test 2 results (the right-hand series of data points in Fig. 4.3) show that, overall, the patients’ ANS results are weakening: sympathetic activity (LFa) decreases to 0.65 bpm2 and parasympathetic activity (RFa) decreases to 0.70 bpm2.


Fig. 4.3
Average resting (baseline) P&S monitoring and time domain ratio responses from two consecutive (serial) tests: pre- and post-therapy modification for orthostatic hypotension
The dynamic P&S monitoring responses to challenge are a sensitive, and possibly earlier, indicator of systemic P and S dysfunction. Figures 4.4 and 4.5 compare the RFa and HF responses to DB and LFa and LF responses to Valsalva challenge, respectively. The frequency domain HRV-alone results, by age, from the two tests are depicted by broken lines in the two figures (triangles for test 1 and “x” for test 2). The P&S monitoring (RFa and LFa, respectively) results, by age, from the two tests are depicted by solid lines in the two figures (diamonds for test 1 and squares for test 2). The thin solid line with no data markers is the published, age-matched low end of the normal range for the DB and Valsalva challenges [51]. The upper end of the age-matched normal range is omitted for clarity.



Fig. 4.4
Dynamic P&S monitoring and HRV-alone responses to deep breathing over age, compared with normals

Fig. 4.5
Dynamic P&S monitoring and HRV-alone responses to Valsalva over age, compared with normals
Assuming that the effects of diabetes accelerate the aging effects on the ANS, the decline in the patient’s P&S responses to challenge (RFa to DB and LFa to Valsalva) would be faster than that of age-matched normals. This assumption is borne out. The younger patients’ results are well below the upper range of the age-matched normal data. As seen in Fig. 4.4 for RFa and Fig. 4.5 for LFa, the P&S results for the younger patients start out above the low end of normal, but the older patients are at or below the low end of normal.
The HRV-alone HF data also start a downward trend that is accelerating with age, but in both tests, the trend reverses after age 65 (see Fig. 4.4). This is not the case for the HRV-alone LF data (see Fig. 4.5). The LF trend is flat for a while and then around age 50 declines significantly and consistently thereafter. In the younger population (theoretically the earlier stages of the disease), P&S monitoring provides earlier indications of AD, while the HRV-alone parameters are still relatively flat.
Orthostatic Dysfunction
Orthostatic dysfunction is a significant and debilitating secondary symptom in diabetes and is commonly found in many chronic diseases. Orthostatic dysfunction may also be a symptom secondary to autonomic dysfunction induced by the primary disease. HRV-alone analysis utilizes the LF/HF ratio (L/H) as the indicator of a change in sympathetic activity in response to a challenge. P&S monitoring uses the changes in the LFa as the sympathetic activity indicator. SW upon assuming a head-up posture (standing) is a physiologic definition of orthostatic dysfunction. A depiction of SW is presented in the top graph of Fig. 4.6. Sympathetic activity is presented on the abscissa (the red portion of the curve), starting under “A” (the resting response) and ending at “F” (the S stand response). Following a parasympathetic decrease, the blue portion of the curve, a sympathetic increase or surge into the gray normal area is expected (see bottom graph of Fig. 4.6). Anything to the left of the normal area is defined as SW. The expected sympathetic surge is between 120 and 500 % [52]. Data suggest that SW is a fall-risk indicator in geriatrics and may contribute to elevated or high BP [5]. Recent clinical results have shown that dehydration is a significant factor in SW. Therapy, starting with recommending proper daily hydration (approximately six to eight glasses of water spread throughout the day, in place of caffeine, sugar, or alcoholic drinks), has corrected SW in approximately 40 % of the cases. Corrected SW is demonstrated as a normal sympathetic surge with standing in the 3- to 12-month follow-up tests.


Fig. 4.6
Examples of an abnormal PC (stand) response, indicating SW (top) and a normal PC response (bottom)
A comparison of L/H and LFa from HRV-alone and P&S monitoring, respectively, was made within patients at risk for orthostatic dysfunction. The assumption is that a drop in either parameter in response to a PC indicates SW. Of these patients, 170 (43.7 %) presented to their physicians with clinical symptoms of OH. LFa identified more patients with orthostatic dysfunction than L/H (73 % vs. 29 %, p < 0.001). LFa identified more patients than physician diagnosis (73 % vs. 18 %, p < 0.01) and combination of diagnosis and symptoms (73 % vs. 35 %, p < 0.002). L/H identified more patients than physician diagnosis (25 % vs. 18 %, p < 0.01), but less compared to combination of diagnosis and symptoms (25 % vs. 35 %, p < 0.001). LFa identified OH more frequently than any decrease in BP (73 % vs. 56 %) and more often than either a 20 mmHg or 10 mmHg decrease in systolic or diastolic BP, respectively (73 % vs. 16 %). Overall, the P&S monitoring parameter, LFa, identified OH (as defined by SW with any drop in BP upon standing) more often than either the HRV-alone method or even the patient’s own physician. In fact, the data suggests that the HRV-alone method would result in a 44 % false-negative rate. These results are similar to those of Stoupakis et al. [53].
Parasympathetic Excess
While Valsalva and PC (standing) are essentially sympathetic challenges, it is expected that a decrease in the parasympathetics will also occur. This makes possible a (dynamic) parasympathetic excess (PE) which is only identified through independent, simultaneous measures of P&S activity (for a broader review of PE, see the section of this compendium labeled section “Parasympathetic excess: diagnosis and therapy”) [54]. PE is defined as an abnormal increase in parasympathetic activity in response to a sympathetic stimulus (Valsalva or PC, see Fig. 4.7). For PC (standing), PE is defined as any increase in parasympathetic activity in response to the PC (only a decrease is expected). For Valsalva, since a quick breath is necessary to initiate the Valsalva maneuver, a 400 % increase or less (including a decrease) in parasympathetic activity in response to Valsalva challenge is acceptable. A change of more than 600 % is considered abnormal. The 400–600 % range is considered borderline.


Fig. 4.7
The graphical definition of (dynamic) parasympathetic excess. The gray area is normal. Above the green line is abnormal. In between is borderline
A comparison of HF and RFa was made within this patient population assuming an increase in either parameter in response to the Valsalva challenge or PC indicates PE. Within this patient population, 262 (67.3 %) patients presented to their physicians with more than four of the clinical symptoms of PE [54]. Valsalva or PC RFa identified more patients with PE than Valsalva or PC HF (94 % vs. 18 %, p < 0.001). In fact, the data suggests that the HRV-alone method would result in a 76 % false-negative rate.
Discussion
P&S monitoring uses the heart and lungs as a window to noninvasively quantify P and S activity. The ANS is known as one of the great mediators of all of the body’s metabolic needs on a moment-by-moment basis. Since the body only has one heart and one set of lungs, the PSNS and SNS are forced into a continual compromise that requires a single HR and a single respiratory rate to suffice for all. By monitoring the compromise from the point of view of respirations and HR, an overall, systemic measure is made. The data suggest that the resting levels of P&S activity are more sensitive than HRV-alone measures. Although both indicators are low and the HRV-alone parameters suggest that average patient in the cohort is near or in DAN, the HRV-alone measures show little or no sensitivity to continuing ANS decline as compared to P&S monitoring measures.
Assuming that the data in Figs. 4.4 and 4.5 represent the trend for a single average patient, these data suggest that autonomic decline is detected more sensitively and specifically. In fact, the delay in the HRV-alone LF decline may be a false indication of better health or stasis of P and S decline, leading both physician and patient to be less aggressive regarding the disease. Furthermore, the steeper slope of the HRV-alone, sympathetic responses to Valsalva, from age 55 through 75, suggests that a small change in age consistently causes a significant change in sympathetic activity. This also suggests that there is no apparent effect of therapy. The differences between the HRV-alone techniques and the P&S monitoring-based technique are the reasons for the high false-negative rates. The HRV-alone LF and LF/HF are mixed measures of the P and S branches. The HF is sometimes considered a parasympathetic measure when the breathing frequency is high and in the HF frequency range. Otherwise, the HF term is merely a noise measure. The HF range is a relatively broad, fixed frequency range, including additional content that is not a parasympathetic activity. The L/H ratio, by inclusion, also suffers from the same problems as the LF and HF terms. When the breathing frequency is not in the HF range, what do HF and L/H mean?
P&S monitoring seems to explain the discrepancies between the monitoring methods. For DB responses, the HF responses do not demonstrate a consistent trend as indicated by the age-matched normal curves. The HF responses are relatively flat over the ages. This may be explained by the fact that the breathing frequency (6 breathes per minute = 0.1 Hz) for DB is in the low-frequency range (LF: 0.04–0.15 Hz) of the HRV spectrum, not in the HF range (HF: 0.15–0.40 Hz). From P&S monitoring, this means that most of the parasympathetic response to DB (RFa, in this case, 0.04–0.16 Hz) is in the LF range. HF captures very little of the parasympathetic response (0.15–0.16 Hz). The rest of what HF captures (0.16–0.040 Hz) is unknown. For Valsalva responses, the decrease in LF is delayed and rapidly declines and eventually parallels the LFa responses. The delay seems to be a result of the mix of P and S activity in the LF response. As the patients age, the parasympathetic activity decreases along with the sympathetic activity resulting in the steep portion of the LF response curves. Eventually, the patients’ parasympathetic response is relatively small compared with the sympathetic response resulting in nearly identical responses between LF and LFa (remember, if there is no parasympathetic activity in the LF region, LFa should equal LF).
P&S monitoring uses RA analysis to identify cardiovagal outflow, which specifies parasympathetic influence on the heart and therefore HRV, without extraneous frequency content and regardless of the breathing frequency. With a more specific measure of parasympathetic activity, a more specific measure of sympathetic activity is computed. Thus, the P&S monitoring respiratory frequency area (RFa) and low-frequency area (LFa) terms are more pure measures and more highly correlated with P&S activity, respectively. When applied clinically, these terms allow proper dissection of P from S to further diagnostics. The examples presented include orthostatic dysfunction and PE. Heretofore, PE could not be quantified and has only been hinted at through confusing and conflicting symptoms. Now PE is clearly identified. Clinically, PE is associated with difficult-to-control BP, blood sugars, or hormone (e.g., thyroid or estrogen) levels and is associated with symptoms of depression (including depression-anxiety disorders), fatigue, exercise intolerance, sleep disturbances, GI upset, or frequent headache or migraine [54]. Further, stand PE may mask orthostatic dysfunction, in terms of a drop in BP; however, it may not mask SW [54]. P&S monitoring enables clear observations of underlying physiology. For both OH and PE, P&S monitoring provides indications of dysfunction with many fewer false negatives than HRV alone. Further, perhaps due to their sensitivity and the fact that the ANS controls organs and tissues, P&S monitoring detects functional changes earlier, changes that may lead to early clinical diagnoses and intervention.
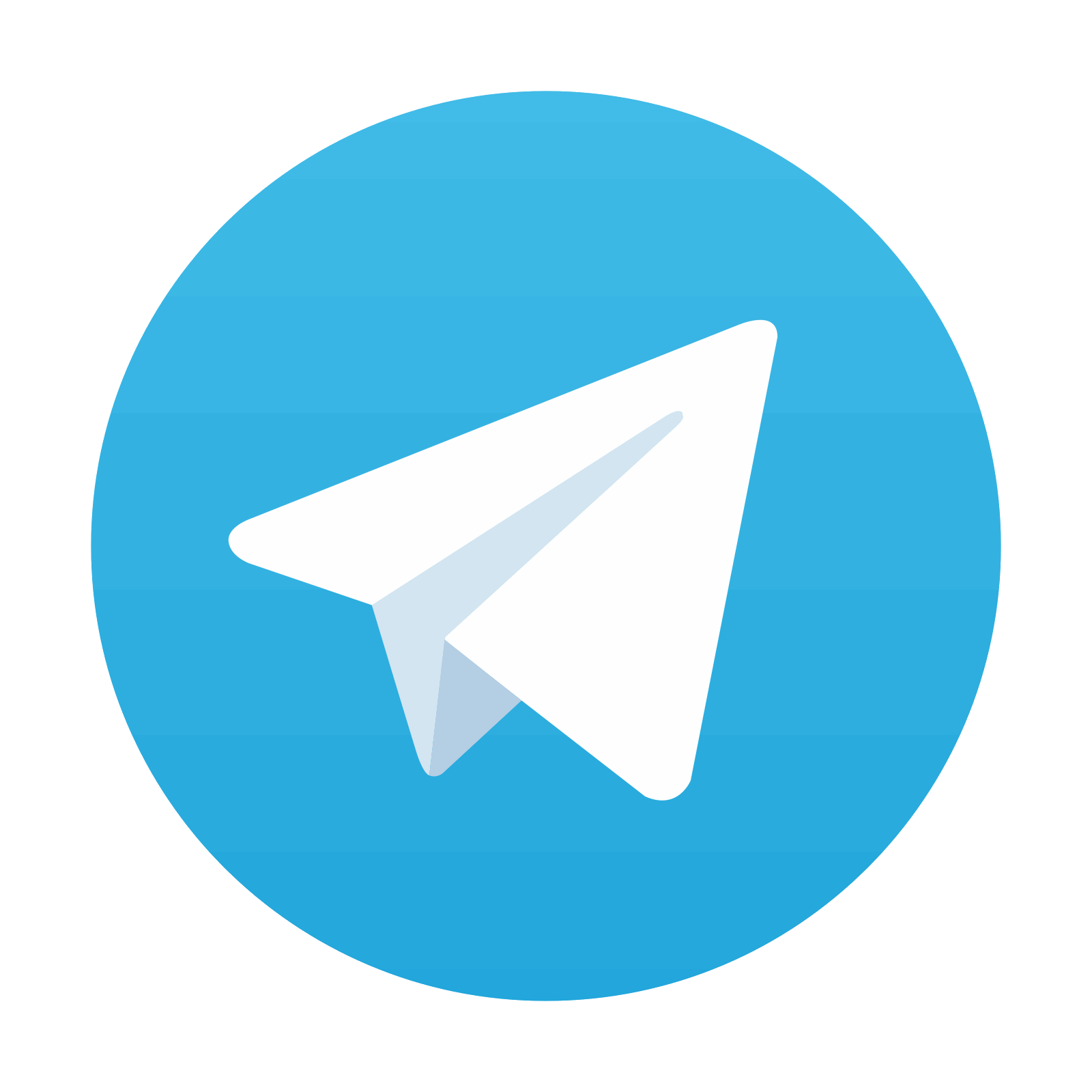
Stay updated, free articles. Join our Telegram channel

Full access? Get Clinical Tree
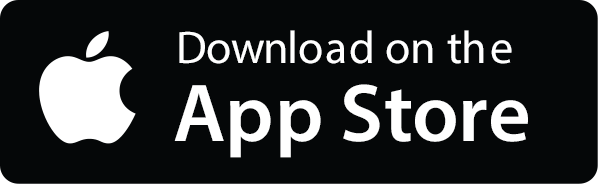
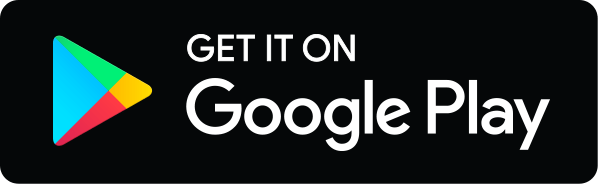