and Muzammil H. Musani2
(1)
Department of Radiology, Stony Brook University Hospital, 100 Nicolls road, Stony Brook, NY, USA
(2)
Department of Medicine and Radiology, Stony Brook University Hospital, Stony Brook, NY, USA
The objective of this chapter is to outline basic epidemiology, pathophysiology, clinical presentation, and imaging findings of common structural heart disease. Structural heart disease can result from a variety of processes effecting the myocardium, pericardium, vasculature, or cardiac valves. This chapter will outline disease processes effecting the aforementioned and highlight imaging findings that can aid in diagnosis and patient management.
5.1 Myocardial Infarction
Myocardial infarction (MI) results from the occlusion of coronary arteries most commonly from atherosclerotic plaque rupture followed by thrombosis leading to ischemia and myocyte damage [1–5]. Patients generally present with chest pain which may radiate to the jaw or arm, dyspnea, hemodynamic instability, etc. [1–5]. Patient evaluation varies within regions and hospitals, generally depending on the expertise available within the institution. In most cases, initial evaluation generally involves obtaining an electrocardiogram and cardiac enzymes [2]. Mortality in acute MI is approximately 20 % with 6 % in hospital mortality [1–3]. Given this, urgent revascularization is stressed in setting of acute MI. Patients with positive initial findings (elevated cardiac enzymes, ECG changes, etc.) are taken for interventional or surgical revascularization. If the aforementioned are unremarkable for ST-elevation MI (STEMI), patients may be observed with serial cardiac enzymes, get stress myocardial perfusion exam, or get a CCTA [1, 2]. In such cases, ECG-gated CCTA evaluation can demonstrate the degree of vessel occlusion (coronary artery filling defect with or without adjacent calcific plaque), myocardial hypoattenuation, and wall-motion abnormalities (hypokinetic, akinetic, or dyskinetic) in acute setting. CCTA can also detect presence of valvular dysfunction, septal or papillary muscle rupture in massive MI [1–3]. Secondary signs of an MI including pericarditis, pericardial effusion, CHF (pulmonary vascular congestion, pleural effusions, etc.) can also be detected. In chronic stages, myocardial thinning, aneurysmal changes, and dystrophic calcifications can be seen [2–4]. Echocardiogram can show wall-motion abnormalities, septal and papillary muscle involvement, and presence of pericardial effusion, however, not to the degree of anatomic detail seen on CCTA. Additionally, the degree of vessel occlusion and extracardiac findings are not seen on echocardiography. MRI can demonstrate findings seen on CCTA. One of the key benefits of MRI is that it provides a precise assessment of myocardial stunning and identifying viable myocardium, which aids in determining interventional management (CABG versus stenting) [6, 7]. Additionally, MRI is the most accurate means of assessing cardiac output and the extent of myocardial involvement [6, 7]. One important limitation of MRI at present is motion artifact (cardiac and respiratory) which can limit evaluation of coronary arteries. These limitations limit the utility of MRI in an acute setting, as accurate knowledge of degree of coronary artery occlusion is the base of patient management. Therefore, at present, MRI is generally used in subacute and chronic settings [2–4, 6, 7].
5.2 Left Atrial and Left Atrial Appendage Thrombus
Thrombus formation in the left atrial body (LAB) and/or the left atrial appendage (LAA) is generally seen in setting of atrial fibrillation or mitral valve stenosis [8–10]. These conditions result in slow blood flow in the LAB and LAA which leads to formation of a thrombus. The thrombus, especially if mobile, is at increased risk of embolization [8, 10–13]. Echocardiography is generally the initial imaging modality used, and it can reveal an echogenic clot in the body of the left atrium or in the left atrial appendage [11–13]. Chronic thrombus (especially if broad based) can develop neovascularity which can lead to presence of Doppler signal, thus can mimic an atrial neoplasm [11–13]. Thrombus on ECG-gated CCTA appears as a hypoattenuated filling defect in LAB and/or LAA. If there is heterogeneity in IV contrast distribution especially in the LAA, then a one-minute delayed CCTA scan can be obtained. Persistence of LAB/LAA filling defect is highly suggestive of a thrombus. If LAA is completely contrast opacified, then the filling defect seen on initial images is likely slow-flow artifact. MRI can also detect LAB/LAA thrombus [8, 11–13]. There are variable appearance of the clot on T1 and T2 sequences depending on the level of hemoglobin (oxygenated, deoxygenated) and hemosiderin present. LAA/LAB thrombus on postcontrast MRI will appear as a filling defect that persists on delayed images [10–13]. Anticoagulation reduces the risk of embolic events [10–13].
5.3 Dilated Cardiomyopathy
Dilated cardiomyopathy (DCM) refers to enlargement of cardiac chambers and contractile dysfunction resulting in heart failure (ejection fraction <40 %) [14, 15]. It usually is biventricular and can be subacute to chronic, resulting from a multitude of causes. The most common cause is ischemic cardiomyopathy in the US and is most prevalent in the elderly usually with risk factors of coronary artery disease [14, 15]. Overall prognosis depends on degree of left ventricular dysfunction [14, 15, 18]. Additionally, older age, male gender, and race (African American) statistically have worse prognosis. Subacute and chronic causes of DCM include myocarditis (infectious, autoimmune conditions), peripartum cardiomyopathy, and drug toxicity (chemotherapy agents, cocaine), metabolic (hypophosphatemia, uremia, hypocalcemia), and endocrinopathies (thyroid dysfunction, Cushing disease, etc.) [14–18]. Idiopathic DCM is the most common cause worldwide and familial DCM accounts for 25 % of these cases [19, 20]. Differential considerations for DCM include restrictive cardiomyopathy, hypertrophic cardiomyopathy, and valvular heart disease [14–18]. Patients usually present with signs and symptoms of heart failure (gradual development of dyspnea on exertion, orthopnea, paroxysmal nocturnal dyspnea, peripheral edema, etc.) [14, 15].
On chest radiographs, the diagnosis can be suggested by widened cardiac silhouette occupying greater than 50 % of the thorax width on posterior-anterior (PA) view. Secondary signs of heart failure can be seen including pulmonary vascular congestion, dilated azygous vein, and pleural effusions [14–17]. Usually if there are signs and symptoms of dilated cardiomyopathies, transthoracic echocardiography (TEE) is the most common initial modality used to evaluate dilated cardiomyopathy. TTE can reveal wall-motion abnormalities, valvular dysfunction, and cardiac chamber volumes; however, given the lack of specificity and variability in ejection fraction, additional modalities are used to evaluate DCM [14, 15, 17].
Cardiac CTA (CCTA) can be used to rule out the most common cause of DCM, i.e., ischemia with high degree of sensitivity and specificity. Ventricular dimensions and volume can be obtained accurately. Newer volume rendered multiphase techniques can help obtain estimated ejection fractions. CCTA is useful in acute setting as the scan can be performed quickly and yields fairly accurate results. Additionally, newer techniques are allowing for assessment of myocardial perfusion with accuracy comparable to those seen in MRI and cardiac nuclear medicine tests [22, 23].
Magnetic resonance imaging (MRI) is the most accurate method for assessing dilated cardiomyopathy as it can give accurate assessment of cardiac chamber size and ventricular ejection fractions [14, 15, 21]. Ventricular dimensions, volume, and ejection fractions can be obtained accurately. In addition, detailed cardiac anatomy can be obtained [21]. However, MRI is limited in evaluating coronary arteries, limiting its utility in acute setting. Thus, CCTA is the preferred modality in evaluating patients in acute to subacute settings to exclude ischemic cardiomyopathy, and MRI can be used for problem solving in equivocal cases.
5.4 Left Ventricular Aneurysm
Left ventricular aneurysm (LVA) refers to a thin fibrotic segment of left ventricular myocardium which may be dyskinetic or akinetic [24, 25]. It most commonly results from prior transmural myocardial infarction and is most commonly seen in anterior and apical segments secondary to left anterior descending artery occlusion [26]. Infrequently, inferior-basal wall aneurysms may be seen after right coronary artery territory transmural infarcts [26]. It develops in approximately eight-to-fifteen percent of patients with ST-elevation myocardial infarction (STEMI) [24–26]. Most LVAs are asymptomatic but can lead to systolic dysfunction [24–26]. Large-sized LVA can have slow blood flow that can lead to the formation of thrombus which can subsequently embolize [24–26]. Additionally, LVA can serve as foci of arrhythmias that can be fatal. Overall, patients with STEMI and resultant LVA have worse outcomes than those without LVA [24–26].
LVA can be seen on echocardiography, CCTA, and MRI. Unenhanced cardiac CT can reveal aneurysmal remodeling of the left ventricle with or without calcification in the infarcted myocardium and/or mural thrombus [27, 28]. CCTA reveals thin fibrotic myocardium with wall-motion abnormalities. Occasionally, filling defect can be seen, which represents thrombus in the LVA [27, 28]. The aforementioned findings can be seen on MRI and echocardiography. In addition, MRI can reveal transmural delayed hyperenhancement in the scarred myocardium of the LVA [24, 28, 29]. Treatment generally involves medical management of modifiable cardiovascular risk factors and revascularization in cases where there is viable myocardium present. Antiarrhythmics are used in setting of ventricular arrhythmias, and implantable cardiac defibrillators are used in patients with high risk of sudden cardiac death [24, 26, 29].
5.5 Left Ventricular Thrombus
Left ventricular thrombus (LVT) is generally seen in patients with transmural myocardial infarction with flow disturbance resulting from wall-motion abnormality and/or LV aneurysm [30–33]. Rarely, it can be secondary to tumor thrombus, Takotsubo cardiomyopathy, and chemotherapy [30, 31]. The thrombus can persist and overtime may develop dystrophic calcification. There is increased risk of embolization if the thrombus is large and mobile.
LVT can be seen on echocardiography as an echogenic mass which may be mobile, pedunculated, or adherent to myocardial wall [33–35]. On CCTA, LVT presents as a filling defect in the left ventricle that persists on delayed imaging. Associated wall-motion abnormalities and coronary artery disease can also be seen [32–34]. Chronic thrombus may contain calcifications, which present as hyperdense foci within the aneurysm. On noncontrast images, the thrombus appears slightly lower in attenuation as compared to normal myocardium [32–34]. Thrombus generally appears hyperintense in acute-to-subacute stages on spin-echo sequences and gradually becomes hypointense in chronic stages [30, 31, 33, 36]. Important to note that there can be reactive thrombus that may form around intraventricular mass. There is no postcontrast enhancement seen in a fresh thrombus [30, 31, 33, 36]. Chronic broad-based thrombus may demonstrate minimal postcontrast enhancement due to neovascularity [30, 31, 33, 36]. In such cases, a biopsy may be performed to exclude intracardiac neoplasm. Treatment generally involves rate and rhythm control plus anticoagulation to reduce risk of embolization [36].
5.6 Hypertrophic Obstructive Cardiomyopathy (HOCM)
Hypertrophic obstructive cardiomyopathy (HOCM) is a primary myocardial disorder affecting the sarcomeres resulting in hypertrophy of the left ventricle (LV) [37, 38, 46]. Additional reversible causes of LV hypertrophy should be ruled out including hypertension or aortic valve stenosis [37, 38]. Clinically, patients can be asymptomatic or present with arrhythmias, sudden cardiac arrest (from ventricular fibrillation or sustained ventricular tachycardia), chest pain, and/or signs of decreased cardiac output (e.g., syncope, orthopnea, paroxysmal nocturnal dyspnea) [37, 38]. It is the leading cause of sudden cardiac arrest in young, otherwise healthy, individuals including athletes [37, 38]. Diagnostic criteria include asymmetric LV thickness of greater than 15 mm; however, functional obstruction has been reported where LV thickness was less than 15 mm [37, 39–42]. Ratio of asymmetric septal hypertrophy can also be used to aid in diagnosis and is defined by the ratio of the septal wall thickness to a nonhypertrophied segment greater than 1.3 [39–42]. The most common location is the basal septum which may or may not result in LV outflow obstruction. Additional areas of involvement include the LV mid-cavity and/or apex [37, 39–42]. Classic cases of HOCM are autosomal dominant with incomplete penetrance and variable expressivity resulting in a wide spectrum of clinical and imaging spectrum [38, 46]. Histopathologic evaluation reveals hypertrophied myocytes arranged in disorganized bundles surrounded by expanded interstitial compartment containing variable degree of collagen deposition (fibrosis) [38].
Echocardiography, generally first diagnostic imaging modality to be used, can show nondilated and/or hyperdynamic left ventricle with variable degree of hypertrophic changes which can result in left ventricular outflow tract obstruction [43]. In severe cases of HOCM, mitral valve regurgitation and/or systolic anterior motion of the mitral valve leaflet can be seen [37, 43]. CCTA and MR imaging can show all the echocardiography finding more precisely and yield more reliable/reproducible measurements [40]. Additionally, MRI can reveal areas of increased fibrosis (delayed hyperenhancement on postcontrast images) which can serve as arrhythmogenic foci and the total volume of delayed hyperenhancement on MRI correlates with risk for sudden cardiac arrest [37, 39–44]. Treatment generally revolves around optimizing cardiac function, which can be done by using beta-blockers and calcium channel blockers to improve diastolic relaxation which results in improved ventricular filling and also reduces risk of arrhythmias. Interventional therapies include permanent pacemaker placement, septal myomectomy, or septal ablation [43, 44]. Automatic implantable defibrillators are used in patients with high risk for sudden cardiac death [43, 44].
5.7 Myocardial Bridging
Myocardial bridging refers to congenital variance in the course of coronary artery which instead of taking its normal epicardial course, takes an intramyocardial course [45, 46]. The degree of bridging is variable generally involving a small segment of the vessel and most patients are asymptomatic [45, 46]. Symptoms, when they occur, are generally during time of maximal systole; myocardial contraction constricts intramyocardial segment of the coronary artery resulting in ischemia and rarely infarction [45]. Severity of symptoms varies depending on the coronary artery involved and the degree of resultant stenosis. Most patients have isolated myocardial bridging; however, in some instances, multiple segments and/or multiple vessels can be involved [45, 46]. The most common location is the mid left anterior descending artery (LAD), followed by left circumflex artery (LCx), and right coronary artery (RCA) [45, 46]. Increased sheer stress in arterial segment proximal to the myocardial bridge predisposes to atherosclerosis [45, 46]. Interestingly, a higher incidence is noted in patients with hypertrophic cardiomyopathy, for reasons poorly understood. Complications include vasospasm, ventricular septal rupture, arrhythmias, myocardial stunning, and sudden cardiac arrest [45, 47].
Coronary computed tomographic angiography (CCTA) is the best imaging modality which depicts intramyocardial locations of coronary artery [45–47]. Echocardiography has limited role in diagnosis of myocardial bridging. MRI can show myocardial imaging; however, motion artifact limits detection of small segment lesions [45–47]. Treatment generally involves rhythm and rate control and in certain cases interventions including percutaneous coronary artery stenting and coronary artery bypass can be performed [45–47].
5.8 Fatty Infiltration of RV (ARVC)
Fatty infiltration of right ventricle, also referred to as arrhythmogenic right ventricular cardiomyopathy (ARVC), is typified by fibrofatty degeneration of the right ventricle which can lead to dysrhythmias and potentially sudden cardiac death [48–51]. It generally presents with syncope, ventricular arrhythmias, and sudden cardiac death [48–51]. Histopathologic evaluation reveals fibrofatty infiltration of the right ventricular myocardium, most commonly involving the anterior wall. ARVC can have a patchy involvement of the myocardium, thus, can result in false negative biopsy. Diagnosis is confirmed with presence of greater than 3 % fibrous tissue and greater than 49 % fat, with the process beginning in the subepicardium and extending endocardially. Autopsy studies have demonstrated involvement of left ventricle as well (40–76 %) [48–51].
Diagnosis is made using combination of clinical and imaging findings. Diagnosis is suggested by fulfilling two major criteria, one major and two minor criteria or four minor criteria [48–52]. Major criteria include RV global dilatation and RV aneurysm, which can be seen on various imaging modalities. Family history, if biopsy proven, is considered major criteria. Minor criteria include ECG abnormalities, regional RV wall-motion abnormalities, and family history of ARVD without pathology proven diagnosis is considered minor criteria [48–52].
Various imaging modalities can aid in diagnosing ARVC, including echocardiography, coronary computed tomographic angiography (CCTA), and magnetic resonance imaging (MRI) [48–51]. Diagnosis of ARVC on imaging is suggested by dilated right ventricle, right ventricular aneurysm, and/or thickened trabeculae. CCTA can reveal dilated right ventricle, reduced systolic function, and RV aneurysm [48–51]. CCTA can also demonstrate intramyocardial macroscopic fat; however, sensitivity and specificity of this is not well established and is under clinical investigation [48–51]. MRI can demonstrate variable degree of fatty infiltration of the myocardium, which is considered major criteria. The extent of myocardial involvement can be appreciated on T1 pre- and post-contrast fat suppressed sequences where the myocardial fat is suppressed by the MR pulse signal thus appearing as hypointense focus within the myocardium [48–51]. The degree of myocardial fibrosis is variable and can have a patchy distribution. These changes can be seen on MRI as areas of delayed hyperenhancement. Wall-motion abnormalities can be appreciated on MRI and echocardiography [48–51]. Treatment usually involves avoiding rigorous exercise or activities that would result in increased sympathetic tone of the heart. Beta-blockers are used in the absence of arrhythmias and/or implantable cardioverter defibrillator. Antiarrhythmics are used in cases with dysrhythmias [52].
5.9 Noncompacted Cardiomyopathy
Noncompacted cardiomyopathy (NCM) is associated with several deep trabeculations of ventricular wall that communicate with ventricular chamber resulting in abnormal ventricular motion and diminished cardiac output [53, 54]. It most commonly involves the left ventricle and referred to as left ventricular noncompaction (LVNC) [53, 54]. Patients generally present with signs and symptoms of heart failure including dyspnea, orthopnea, hemodynamic instability, etc. [55]. Tachyarrhythmias and embolic complications can be seen [55]. LVNC can be an isolated finding or be seen with congenital heart disease. Prognostic indicators include number of effected segments, diastolic dysfunction, and heart failure at presentation [53–55].
On echocardiography, CCTA, and MRI, there is visualization of distinct two-layered appearance of trabeculated (noncompacted) myocardium and compact myocardium generally involving the left ventricular apex and/or mid and distal segments of inferior and lateral walls [53–56]. Echocardiography is generally the first modality used. CCTA and/or MRI is used to gain more precise anatomic and functional information, which is especially helpful in surgical planning [53–56]. Treatment revolves around management of heart failure, tachyarrhythmia, and/or embolic disease [53–56].
5.10 Infectious Endocarditis
Infectious endocarditis, as the name implies, involves infection of the heart most commonly the valves with infrequent involvement of the cardiac chambers [57–60]. Common infectious agents include Staphylococcus and Streptococcus species and rarely can be secondary to tuberculosis, fungal organisms, and form HACEK organisms (Hemophilus, Actinobacillus, Cardiobacterium, Eikenella, Kingella) [57–60]. Diagnosis is made on combination of clinical, laboratory, and imaging findings using modified Duke Criteria (major and minor). Major criteria include positive blood cultures and positive echocardiography findings (vegetation, new regurgitant valve). Minor criteria include fever (>38 °C), risk factors (IV-drug abuse or predisposing cardiac conditions, e.g., valvular disease, prosthetic valve, pacemaker), vascular involvement (arterial embolic to lung, brain, liver, kidney, etc.), and immunological findings (e.g., osler nodes, positive rheumatoid factor). Need to fulfill two major or one major and three minor criteria to confirm diagnosis of endocarditis [57–59, 62]. Note, however, given early treatment and delayed complications, the modified Duke criteria may not be present on initial presentation [57–59, 62]. Complications including septic emboli, valvular cusp perforation, perivalvular abscess, pseudoaneurysm, and/or fistula portend poor prognosis with reported mortality up to 40 % [57–59, 62]. Risk of embolic disease is increased in patients with mobile vegetations and/or vegetation size greater than 1 cm [57–59, 62].
Initial imaging modality is usually transthoracic echocardiography (TEE) which may demonstrate vegetations, which appear as oscillating irregular-shaped mass adherent to the valve and/or endocardium [57–59, 62, 66, 67]. Complications including regurgitation, abscess, and pseudoaneurysm may be seen on TEE. However, due to the poor sensitivity and specificity of TEE, transthoracic echocardiography is used which yields modest improvement in sensitivity and specificity. However, given TEE’s invasive nature and use of anesthesia, alternative diagnostic modalities can be used especially in more critically ill patients whose clinical course may get complicated with an invasive procedure and/or patients who may not tolerate anesthesia/intubation [57–59, 62, 66, 67]. ECG-gated cardiac computed tomographic angiography (CCTA) can detect valvular vegetations, perivalvular or aortic root abscess, and pseudoaneurysm with a similar sensitivity and specificity as compared to TEE [60–67]. Pseudoaneurysm presents as contrast filled space in communication with the cardiac chamber and/or aortic root. Leaflet perforation, though difficult to detect, presents as discontinuity in a cusp [61–67]. Fistula can vary in size and is represented by communication between cardiac chambers [61–67]. The benefits of CCTA is that it is noninvasive, rapidly performed, provides better anatomic detail, which can aid in surgical planning, if needed. Additionally, CCTA can provide information on involvement of the coronary arteries and septic pulmonary emboli. MRI can reveal cardiac involvement of infectious endocarditis with slightly improved sensitivity and specificity; however, given the critical state of the patients and long duration of the MRI exam, CCTA should be considered [61–67]. Also, CCTA is the preferred modality to detect pulmonary involvement. Treatment involves long-term intravenous antibiotic. Surgical treatment may be required in complications including large vegetations, severe valvular regurgitation, pseudoaneurysm, abscess, and fistulae [57–59, 65, 67].
5.11 Aortic Root Abscess
Aortic root abscess is not an uncommon complication of infectious endocarditis and is seen in approximately 30–40 % [67–69]. Patients usually present with persistent bacteremia and develop new conduction defects in spite of appropriate course of antibiotics [67, 69, 70]. Aortic valve and aortic annulus are more prone to abscess formation for reasons unclear and the abscess can further extend into adjacent myocardium (especially when involves the right and noncoronary cusps), destroying conductive tissue resulting in heart block [68–70]. The aortic root abscess can also rarely extend up to and occlude the coronary arteries resulting in myocardial ischemia/infarction [70]. In infectious endocarditis, aortic root/perivalvular abscess portends poor prognosis with mortality rates twice as high rates of mortality, usually secondary to systemic embolization [67, 68].
Transthoracic echocardiography (TEE), despite its low sensitive (28 %) is usually the first study used to evaluate potential aortic root abscess [67, 69, 71]. Transesophageal echocardiography (TEE) is the second modality used with sensitivity and specificity of 87 and 95 %, respectively [67, 69, 71]. Although TEE is more sensitive, it still can miss more than one in ten patients with aortic root abscess [67, 69, 71].
ECG-gated coronary computed tomographic angiography (CCTA) can be used to evaluate aortic root abscess with high sensitivity and specificity [72]. The noninvasive nature of the technique, with great anatomic detail, makes it a viable option for evaluating patients with suspicion of aortic root abscess, especially those who are too sick to undergo general anesthesia required for TEE [72]. In addition to localization of the abscess, CCTA can evaluate extent of the abscess and exclude involvement of coronary arteries, which the TEE/TTE is unable to do [72].
5.12 Pericardial Effusion, Pericardial Calcification, and Constrictive Pericarditis
Pericardial calcifications can result from prior trauma, pericarditis (infectious, autoimmune, uremic, radiation, etc.), postsurgical, or be idiopathic [73–80]. The distribution or appearance of pericardial calcification is nonspecific, and diagnosis is generally made based on clinical findings [73–77, 80]. Constrictive pericarditis is a key diagnosis one must exclude when evaluating patients with pericardial calcifications as it can result in hemodynamic changes, chest pain, and congestive heart failure [80]. Echocardiography is used as primary imaging modality due to its cost, availability, and portability [77, 80]. Characteristic findings of constrictive pericarditis on echocardiography include early diastolic filling with rapid with elevation and equalization of intrachamber diastolic pressures [77, 80]. Abnormal septal motion and absent inspiratory collapse of IVC are secondary signs suggestive of constrictive pericarditis [77, 80]. Given limitations and decreased sensitivity and specificity of TTE and limited scanned field of view of TEE, echocardiograph is limited in assessment of constrictive pericarditis. CT and MRI provide a more complete evaluation [73–80]. On CCTA, combination of pericardial thickening (greater than 4–6 mm), pericardial enhancement, plus peri-/epicardial fat stranding raise the possibility of pericarditis [75, 76]. Impaired ventricular filling (presence of dilated superior and inferior vena cava along with tubular ventricles) is suggestive of constrictive physiology [75, 76]. The presence of calcifications further support the diagnosis of constrictive pericarditis, especially if greater than fifty percent of pericardium is calcified [75, 76]. Pericardial calcifications are best seen on CCTA as hyperdense foci which may be linear or nodular and may be segmental or circumferential in distribution [75, 76]. CCTA can also demonstrate extracardiac manifestations of constrictive pericarditis, including pulmonary vascular congestion, pleural and pericardial effusions, hepatic venous congestion, ascites, etc. [73–80]. CT can help characterize pericardial effusion. Hemopericardium on unenhanced images appear as hyperdense fluid measuring 40–60 Hounsfield Units. Pericardial effusion measuring less than 20 Hounsfield units is likely simple and transudative. Pericardial effusion ranging from 20 to 40 Hounsfield units is indeterminate, can be transudative or exudative, and requires clinical correlation [75, 76]. MRI can demonstrate the aforementioned with variable degree of sensitivity and specificity. MRI is more sensitive and specific in characterizing pericardial effusion and detect presence of myocardial involvement as compared to CCTA and echocardiography. MRI and echocardiography are less sensitive techniques for detection of pericardial calcification and are generally not used to evaluate pericardial calcifications [73–77, 79, 80]. Reversible and/or modifiable factors (uremia, inflammation, infections, etc.) are managed medically. Stable, asymptomatic patients without constrictive physiology are generally observed. Severely symptomatic patients or those with constrictive physiology undergo surgical stripping of the pericardium [73–80].
5.13 Pericardial Cyst
Pericardial cyst is a benign congenital mediastinal cyst attached to the pericardium [75, 76]. These cysts can be congenital (resulting from the embryologic defect in the coelomic cavity) or acquired (posttraumatic, sequela of pericarditis) [75, 76, 81–83]. It is usually an incidental finding. Size of the cyst varies from 2 cm up to 30 cm [75, 76, 81–83]. On imaging, it presents as a smoothly marginated fluid collection adjacent to the heart, usually in the right anterior cardiophrenic angle [75, 76, 81–83]. Echocardiography can demonstrate an anechoic mass in the pericardial space. Presence of internal septations help discriminate unilocular from a multilocular cyst [75, 76, 81–83]. On CCTA, the cyst appears as a low attenuation (less than 20 Hounsfield units) smooth homogenous mass with imperceptible walls, without internal enhancement, calcifications, and with or without internal septations [75, 76, 81–83]. MRI is the most sensitive and specific modality; however, cost and image scan time have limited the use of MRI as first line. At present, MRI is generally used for problem solving and to exclude neoplasm [75, 76, 81–84]. For example, MRI is used in cases where the cyst may contain equivocal internal echoes or Doppler flow on ultrasound, equivocal internal, peripheral or septal enhancement of the cyst [84]. Cases where MRI findings are equivocal or nondiagnostic, close follow-up and/or biopsy can be performed to exclude cystic neoplasm [84].
< div class='tao-gold-member'>
Only gold members can continue reading. Log In or Register a > to continue
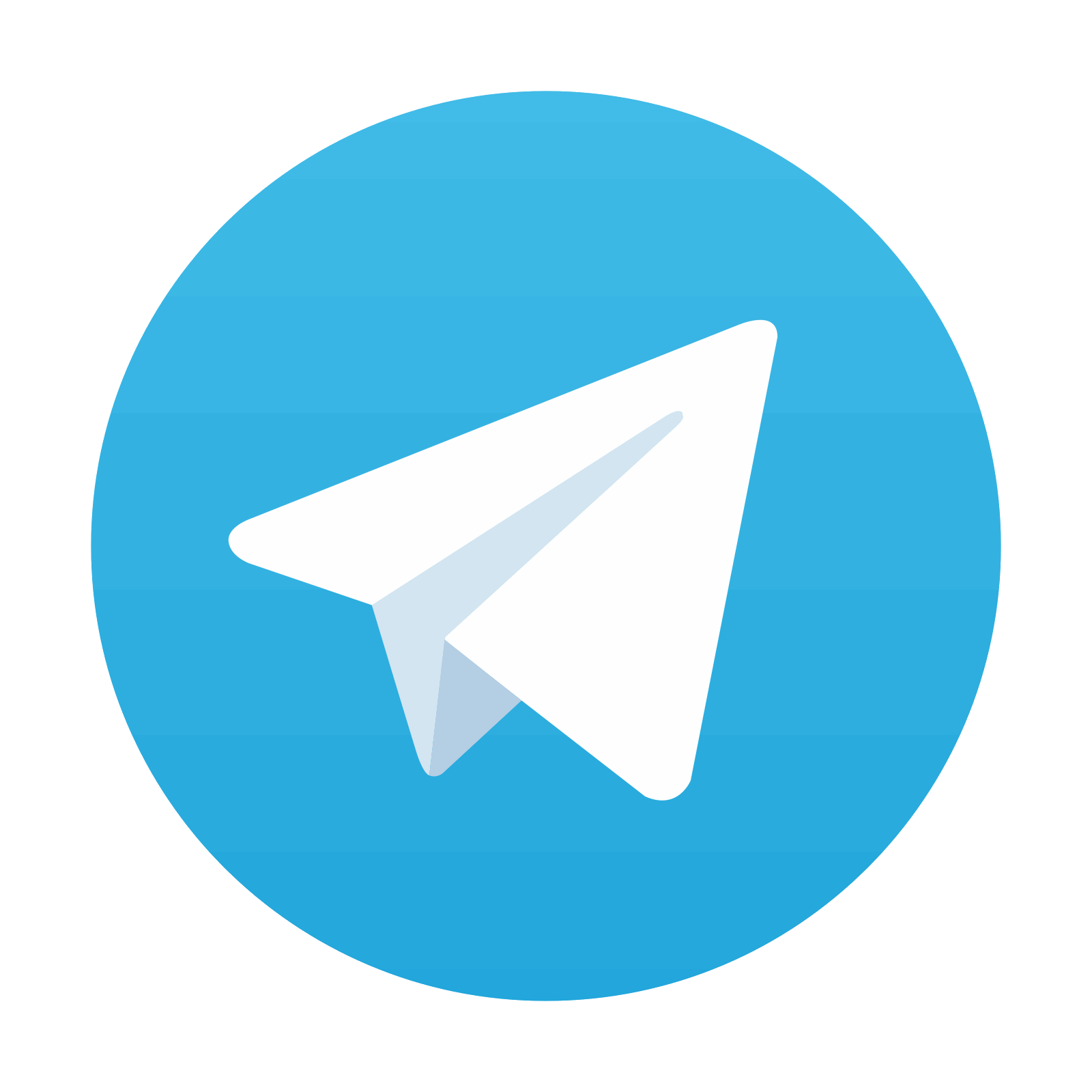
Stay updated, free articles. Join our Telegram channel

Full access? Get Clinical Tree
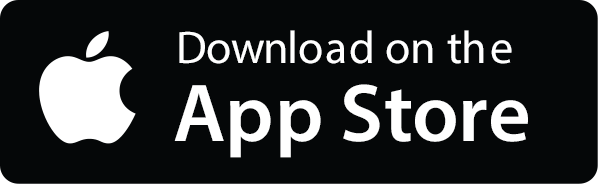
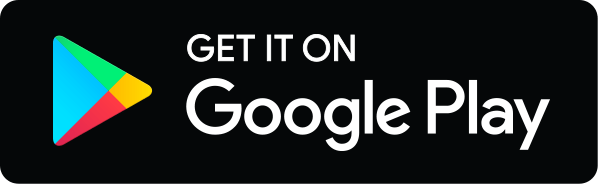