Right ventricular failure (RVF) after left ventricular assist device (LVAD) placement is associated with increased morbidity and mortality. Echocardiography is a primary imaging method in the assessment of cardiac function; however, visualization of the right-sided heart is often technically difficult in patients with heart failure. We aimed to create a simple and generally applicable scoring system based on “left-sided echocardiographic parameters” to provide complementary information for predicting RVF after LVAD surgery. We reviewed 111 consecutive patients undergoing LVAD surgery from 2007 through 2010. Echocardiograms within 5 days before surgery were analyzed. RVF was defined as an unexpected RV assist devices requirement, nitric oxide inhalation >48 hours, and/or inotropic support >14 days. Thirty-five patients (32%) developed RVF. LV end-diastolic dimension (LVEDD) was smaller, LV ejection fraction was greater, and the left atrial diameter/LVEDD ratio was greater (p < 0.05 for all comparisons) in patients with RVF than in those without RVF. An RVF score (LV echocardiographic RVF score) was determined as a sum of points based on receiver operator characteristics analysis: LVEDD >78, 79 to 70, and <70 mm; LV ejection fraction ≤19%, 19% to 33%, and >33%; and left atrial diameter/LVEDD <0.63, 0.63 to 0.68, and >0.68; each variable was associated with 0 and 1 point and 2 points, respectively. LV echocardiographic RVF score ≥3 was associated with RVF with a sensitivity of 88.6% and score ≥5 with a specificity of 80.3%. In conclusion, patients with relatively small LV size, preserved LV contraction, and dilated left atrium were at higher risk for RVF after LVAD surgery. In conclusion, LV echocardiographic RVF score provides a novel tool to predict RVF after LVAD surgery, which does not involve invasive or technically complicated procedures.
Hemodynamic and echocardiographic studies have been performed to identify preoperative parameters associated with right ventricular failure (RVF) after left ventricular assist device (LVAD) surgery ; however, no consistently validated variables have been established across the previous studies. In addition, previously described RVF risk assessment strategies have contained several limitations in their general applicability. Some studies have involved invasive hemodynamic assessments, and some assessments have been based on a single measurement of a laboratory parameter, which can vary in the days immediately before surgery. Echocardiography is a primary imaging method in the assessment of cardiac structure and function. It can be performed at a patient’s bedside, and results are immediately available. Previously reported echocardiographic parameters associated with risk for developing RVF after LVAD implantation have included tricuspid annular motion and RV-to-LV end-diastolic diameter ratio. However, it can sometimes be technically challenging to obtain standardized RV images that allow quantitative assessment from patients with advanced heart failure, particularly if patients are severely congested, intubated, and/or have a markedly enlarged left ventricle that obscures the right ventricle. Preoperative transesophageal echocardiograms can provide better acoustic windows compared to transthoracic echocardiograms, but transesophageal echocardiography is limited by its invasive nature and technical requirements. Generally applicable, noninvasive, technically easy, and reproducible echocardiographic parameters to predict RVF after LVAD surgery would be clinically beneficial. In the present study, we focused on left-sided parameters derived from 2-dimensional transthoracic echocardiograms to create a simple scoring system to provide information for risk stratification of RVF development following LVAD surgery.
Methods
We retrospectively reviewed 111 patients (87 men, 24 women) with advanced heart failure who underwent LVAD implantation at Columbia University Medical Center from January 2007 through June 2010. Patients who required LVAD surgery because of acute cardiogenic shock or post-transplantation graft failure and those supported by preceding mechanical support including intra-aortic balloon pump, percutaneous cardiopulmonary support system, and/or any short-term device as bridge-to-bridge therapy were excluded from the study. Patients in acute cardiogenic shock were excluded from this study because such patients have a high frequency of undergoing biventricular assist device implantation regardless of their preoperative RV function. Echocardiograms, laboratory examinations, and hemodynamic variables within 5 days before LVAD placement were reviewed and compared between patients who developed RVF after LVAD surgery and those without RVF. For patients who had multiple examinations, data that were closest to the date of surgery were used in this analysis. RV stroke work index was calculated by the following equation: RV stroke work index = ([mean pulmonary artery pressure − mean right atrial pressure] × stroke volume index). RVF was defined as the need for an RV assist device, nitric oxide inhalation >48 hours, and/or inotropic support >14 days or restarting after 14 days. The present study was approved by the institutional review board of Columbia University Medical Center. All patients provided written informed consent before inclusion into the study.
All patients underwent transthoracic echocardiography preoperatively using the Sonos-5500 or Sonos-7500 machines (Philips Healthcare Corp., Andover, Massachusetts). In the present study, we focused only on left-sided heart parameters derived from 2-dimensional echocardiography to create a score for predicting RVF development (LV echocardiographic RVF score). Only for evaluating the reproducibility of right-sided echocardiographic parameters that were previously described to be associated with RVF development after LVAD were inter- and intraobserver viabilities for the tricuspid annular plane systolic excursion and RV-to-LV end-diastolic diameter ratio calculated for our cohort.
Left-sided 2-dimensional echocardiographic parameters used in this study included measurements of thickness of the ventricular septum and LV posterior wall, LV end-systolic diameter, LV end-diastolic diameter (LVEDD), and left atrial diameter (LAD) obtained from the apical 2- or 4-chamber view. The ratio of LAD to LVEDD was calculated. LV ejection fraction (LVEF) was assessed by the biplane Simpson method.
Data were presented as mean ± SD. Normality was evaluated for each variable based on normal distribution plots and histograms and by Kolmogorov–Smirnov test. Clinical characteristics and hemodynamic and laboratory data were compared between patients with and without RVF using Student’s unpaired 2-tailed t test for continuous variables or chi-square analysis for noncontinuous variables.
Stepwise forward regression analysis was performed to identify 2-dimensional echocardiographic parameters associated with RVF after LVAD placement. The optimal cut-off value of selected variables was determined using a receiver operator characteristics curve. The point of each echocardiographic parameter for RVF risk was determined according to the following formula: values that exceeded the value of 10% increase in specificity from the cut-off value were given 2 points, values between a 10% increase and a 10% decrease in specificity from the cut-off value were given 1 point, and values that did not reach 10% decrease in specificity from the cut-off value were given 0 point. Then, the total RVF risk points (LV echocardiographic RVF score) were calculated for each patient. The maximum LV echocardiographic RVF score is 6 and the minimum score is 0.
Furthermore, laboratory and hemodynamic variables that showed a significant difference between patients with and without RVF were added to the LV echocardiographic RVF score. Patients who had laboratory and hemodynamic variables higher than the median received an additional 1 point, and patients with such variables lower than its median received 0 point.
Sensitivity, specificity, and predictive accuracy were determined and expressed as percentages.
Inter- and intraobserver reproducibilities of echocardiographic measurements were evaluated by the interclass correlation coefficient. All statistical analyses were performed using JMP 7.0 (SAS Institute, Cary, North Carolina).
Results
Of 111 patients, 35 patients (31.5%) developed RVF after LVAD surgery, including 15 patients with an RV assist device requirement (12.6%), 17 patients with prolonged inotropic support (15.3%), and 3 patients with >48-hour nitric oxide inhalation (2.7%) postoperatively. Clinical characteristics of patients with and without RVF development after LVAD surgery are presented in Table 1 .
Variable | RVF | p Value | |
---|---|---|---|
Yes | No | ||
(n = 35) | (n = 76) | ||
Age at left ventricular assist device surgery (years) | 56.4 ± 14.6 | 55.4 ± 15.5 | 0.745 |
Men | 26 (74%) | 61 (80%) | 0.482 |
Body surface area at time of surgery (m 2 ) | 1.97 ± 0.25 | 1.99 ± 0.22 | 0.765 |
Reason for left ventricular assist device surgery | |||
Nonischemic cardiomyopathy | 23 (66%) | 52 (68%) | 0.778 |
Ischemic cardiomyopathy | 12 (34%) | 24 (32%) | 0.778 |
Treatment before left ventricular assist device surgery | |||
Inotropic dependency | 21 (60%) | 45 (59%) | 0.937 |
Loop diuretic | 34 (97%) | 67 (88%) | 0.092 |
β Blockers | 16 (46%) | 49 (65%) | 0.063 |
Angiotensin-converting enzyme inhibitors or angiotensin II receptor antagonists | 19 (54%) | 33 (43%) | 0.287 |
Aldosterone antagonist | 20 (57%) | 43 (57%) | 0.956 |
Type of left ventricular assist device | |||
Pulsatile-flow left ventricular assist device | 14 (40%) | 18 (24%) | 0.082 |
Continuous-flow left ventricular assist device | 21 (60%) | 58 (76%) | 0.082 |
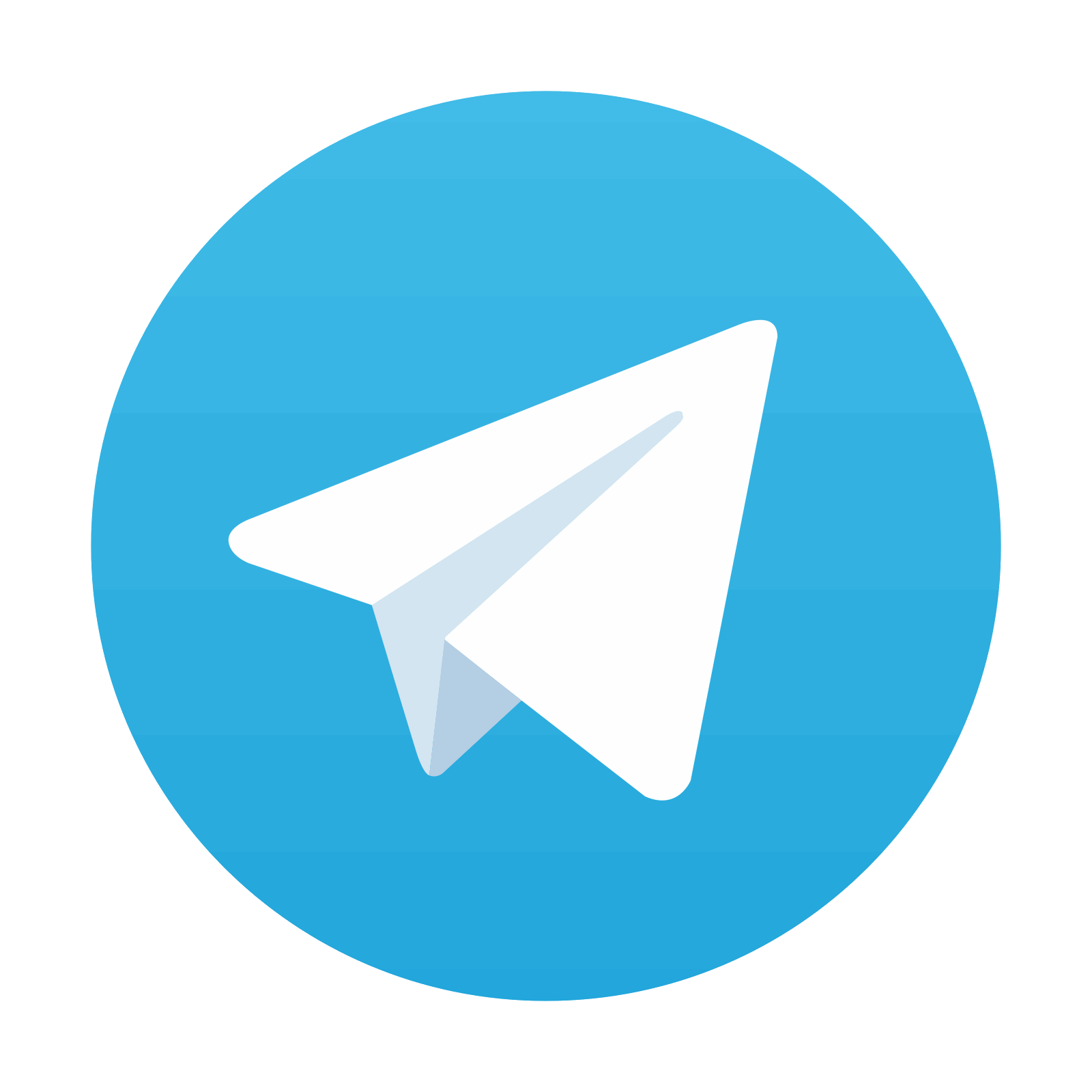
Stay updated, free articles. Join our Telegram channel

Full access? Get Clinical Tree
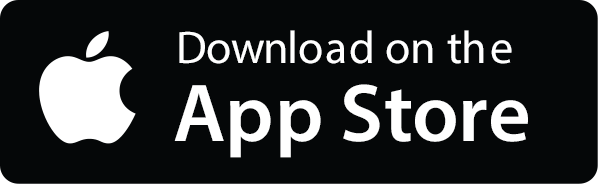
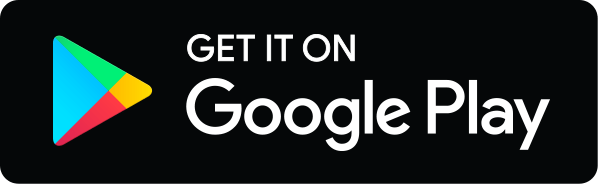
