Background
Right ventricular (RV) dysfunction after acute myocardial infarction (AMI) is frequent and associated with poor prognosis. The complex anatomy of the right ventricle makes its echocardiographic assessment challenging. Quantification of RV deformation by speckle-tracking echocardiography is a widely available and reproducible technique that readily provides an integrated analysis of all segments of the right ventricle. The aim of this study was to investigate the accuracy of conventional echocardiographic parameters and speckle-tracking echocardiographic strain parameters in assessing RV function after AMI, in comparison with cardiac magnetic resonance imaging (CMR).
Methods
A total of 135 patients admitted for AMI (73 anterior, 62 inferior) were prospectively studied. Right ventricular function was assessed by echocardiography and CMR within 2 to 4 days of hospital admission. Right ventricular dysfunction was defined as CMR RV ejection fraction < 50%. Right ventricular global peak longitudinal systolic strain (GLPSS) was calculated by averaging the strain values of the septal, lateral, and inferior walls.
Results
Right ventricular dysfunction was documented in 20 patients. Right ventricular GLPSS was the best echographic correlate of CMR RV ejection fraction ( r = −0.459, P < .0001) and possessed good diagnostic value for RV dysfunction (area under the receiver operating characteristic curve [AUROC], 0.724; 95% CI, 0.590–0.857), which was comparable with that of RV fractional area change (AUROC, 0.756; 95% CI, 0.647–0.866). In patients with inferior myocardial infarctions, the AUROCs for RV GLPSS (0.822) and inferolateral strain (0.877) were greater than that observed for RV fractional area change (0.760) Other conventional echocardiographic parameters performed poorly (all AUROCs < 0.700).
Conclusions
After AMI, RV GLPSS is the best correlate of CMR RV ejection fraction. In patients with inferior AMIs, RV GLPSS displays even higher diagnostic value than conventional echocardiographic parameters.
Right ventricular (RV) dysfunction is an independent predictor of adverse prognosis after acute myocardial infarction (AMI), even in the absence of clinically apparent RV infarction. Echocardiographic assessment of the right ventricular remains challenging because of its complex geometry, its marked load dependence, the limited definition of endocardial surface caused by the heavily trabeculated myocardium, and its retrosternal position. Contrast-enhanced cardiac magnetic resonance imaging (CMR) provides a completely noninvasive, highly accurate, and comprehensive evaluation of RV function without acoustic window limitations. For all of these reasons, CMR is now considered the gold standard for RV assessment. Nonetheless, echocardiography, being widely available and inexpensive, with no side effects and achievable at the bedside, remains the modality of choice for cardiac evaluation after AMI in routine clinical practice. There is currently significant enthusiasm for echocardiographic strain measurement derived from two-dimensional (2D) speckle-tracking echocardiography (STE), as it provides an objective quantification of myocardial mechanical function. Although strain was primarily developed for left ventricular (LV) evaluation, numerous studies have demonstrated its usefulness for subtle RV assessment in various pathologies, such as pulmonary arterial hypertension and congenital heart diseases. Strain analysis may also be useful for identifying segmental dysfunction, which may not be registered by a single-plane measure of RV fractional area change (RVFAC) or tricuspid annular plane systolic excursion (TAPSE). The clinical importance of RV dysfunction after AMI has long been underestimated, although meta-analyses have reported an increased risk for mortality with RV involvement. Moreover, in post-AMI patients with LV dysfunction, RV function is weakly correlated with LV function and is independently associated with an increased risk for mortality and adverse outcomes. In this context, a comprehensive assessment of RV function appears essential after AMI.
The objective of the present study was to compare the accuracy of conventional echocardiographic parameters and STE in assessing RV dysfunction, as measured by CMR, in patients with AMIs.
Methods
Study Population
The study population encompassed all patients with successfully reperfused first acute ST-elevation myocardial infarctions (STEMIs) included in a prospective monocentric cohort study (Relation between Aldosterone and Cardiac Remodeling after Myocardial Infarction [REMI]) performed in a university hospital between April 2010 and October 2013. Approval of the institutional review board and informed consent were obtained before inclusion. The REMI study was designed primarily to determine whether aldosterone blood concentration can predict cardiac remodeling after AMI. Here we present the results of an ancillary imaging study. Cardiac function was assessed by magnetic resonance imaging and echocardiography during the first 4 days after percutaneous transluminal coronary angioplasty (PTCA). Eligibility criteria were age ≥ 18 years, hospitalization for a first STEMI revascularized in the acute phase by primary PTCA, stable clinical state, and regular sinus rhythm. Exclusion criteria were any contraindication to CMR examination, severe claustrophobia, history of oversensitivity to gadolinium salts, nonischemic cardiomyopathy, cardiac surgery scheduled within 6 months, of childbearing age without effective contraception, and unwillingness or inability to participate in a long-term trial.
Standard 12-lead electrocardiograms were recorded before PTCA, immediately after revascularization, and twice daily until hospital discharge. Troponin Ic and creatine phosphokinase levels were assayed three times daily during the first 2 days to determine peak values.
A total of 145 patients with first STEMIs were enrolled in the study. However, 10 patients could not be analyzed, because of lack of echocardiographic assessment ( n = 4) and/or magnetic resonance imaging study ( n = 4) or STEMI strictly limited to the lateral leads ( n = 4).
To determine the pathologic threshold for STE-derived parameters (see “Statistical Analysis”), echocardiograms were obtained in 30 healthy subjects. These volunteers did not undergo CMR and were included in the STANISLAS study, a healthy volunteer cohort followed at Nancy University Hospital (ClinicalTrials.gov identifier NCT01391442 ).
Contrast-Enhanced CMR
Patients were scanned between day 2 and day 4 after STEMI in a supine position using a 3-T whole-body scanner (Signa Excite HD; GE Healthcare, Milwaukee, WI) equipped with an eight-element phased-array surface coil. A steady-state free precession pulse sequence was used to assess LV function in contiguous short-axis planes. Main acquisition parameters were as follows: slice-thickness, 8 mm; repetition time, 3.5 to 3.9 msec; 14 to 16 k-space lines per segment; 30 phases per cardiac cycle with view sharing; field of view, 32 to 38 cm; phase field of view, 0.9; and matrix size, 224 × 224.
LV myocardial delayed enhancement was assessed during a dedicated sequence, 10 to 15 min after intravenous gadoteric acid injection (0.15 mmol/kg) (Dotarem; Guerbet Laboratories, Roissy, France).
The endocardial contours of the right and left ventricles were obtained using dedicated software (Mass Analysis Plus version 4; Medis Medical Imaging, Leiden, The Netherlands) and a method already described and were assessed by our group, which required careful manual tracing of the endocardial borders of the right ventricle.
Echocardiography
Complete transthoracic echocardiography (TTE) was performed with patients in the left lateral decubitus position within ±48 hours of the completion of CMR. Images were obtained with a simultaneous electrocardiographic signal, using a commercially available system (Vivid 7 or Vivid 9; GE Vingmed Ultrasound AS, Horten, Norway). During breath-hold, M-mode and 2D images were acquired in cine-loop format from three consecutive beats. Echocardiographic images were obtained from the parasternal long-axis view, from short-axis views at the basal, median, and apical levels, and from the three standard LV apical views (four-chamber [4C], two-chamber [2C], and three-chamber views). All 2D images were acquired at a frame rate of 50 to 70 frames/sec. Measurements and analysis of echocardiographic images were performed offline (EchoPAC version 110.1.0; GE Vingmed Ultrasound AS), in accordance with existing guidelines. LV volumes and LV ejection fraction (LVEF) on TTE were calculated using the biplane Simpson technique. LV global longitudinal peak systolic strain (GLPSS) was assessed using automated function imaging from apical 4C and 2C views and the long-axis view, as previously described.
Right Ventricular Functional Analysis ( Figure 1 )
Conventional Parameters
RVFAC was calculated as the percentage of change in RV area between end-diastole and end-systole from the apical 4C view. TAPSE was obtained by placing an M-mode cursor through the tricuspid lateral annulus in a 4C view and measuring the amplitude of longitudinal motion of the annulus. RV myocardial performance index was obtained by recording tricuspid inflow and RV outflow tract ejection flow by pulsed-wave Doppler. Tricuspid annular peak systolic velocity (S′) was measured at the lateral corner at the tricuspid annulus by Doppler tissue imaging in the apical 4C view. RV function was also visually assessed by wall motion score index (WMSI). The lateral, septal, and inferior walls were divided into three segments, and the motion of each segment was scored on a scale ranging from −1 to 2 (−1 = dyskinetic, 0 = akinetic, 1 = hypokinetic, 2 = normal). The inferolateral RV WMSI was calculated as the sum of the scores of inferior and lateral walls divided by the number of segments analyzed. The global RV WMSI was calculated the same way but adding the septal wall.

Measurement of Strain by STE
For RV STE, cine loops from apical views (4C and RV 2C views) were recorded using a phased-array transducer from the apex at end-expiratory apnea. Three RV walls were analyzed: the lateral and septal walls from an apical 4C view and the inferior wall obtained from a modified apical 2C view focused on the right ventricle. This latest view is easy to obtain by rotating the probe counterclockwise with an angle of 90° from the 4C view centered on the right ventricle and displays the RV inferior wall as a straight wall that is easy to analyze with 2D strain echocardiographic analysis software. Each wall was divided into three segments (basal, middle, and apical) such that the right ventricle was ultimately divided into nine segments. Peak longitudinal systolic strain was measured using speckle-tracking offline analysis on a workstation using EchoPAC with Q-Analysis software. The operators were blinded to the localization of myocardial infarction (MI). All images were recorded at a frame rate of ≥50 frames/sec for reliable analysis, during three consecutive beats for each wall. The endocardial surface of the analyzed myocardial wall was manually traced using a point-to-click approach. An epicardial surface tracing was then automatically generated by the software, thus creating a region of interest that could be manually adjusted if necessary. The software then generated strain curves for each selected myocardial segment, and tracking quality was automatically scored as either acceptable or unacceptable. For each patient, measurements of the peak strain value of all RV segments were repeated and averaged on three consecutive cycles by the same operator. The mean lateral, septal, and inferior strain values were calculated as the averages of strain values of the three segments (basal, middle, and apical) of each corresponding RV wall, the mean 4C strain (average of strain values of the six segments of the lateral and septal walls), inferolateral strain (average of inferior and lateral strain), and RV GLPSS (average of strain values of the nine segments). RV inferolateral strain was measured to minimize the influence of the septum, which could reflect primarily LV dysfunction.
The various echocardiographic parameters could be obtained in 129 of 135 patients for lateral strain, 125 for inferior strain, 134 for septal strain, 129 for 4C strain, 122 for inferolateral and RV GLPSS, 135 for TAPSE, 117 for RV myocardial performance index, 133 for RVFAC, 131 for S′, and 131 for inferolateral and global RV WMSI.
Statistical Analysis
Continuous data are presented as mean ± SD for normally distributed variables and as median (interquartile range) for variables not normally distributed. Categorical data are presented as frequencies and percentages. Patients were divided into two subgroups according to the location of the MI: inferior MI (including inferolateral MI) and anterior MI (including anterolateral MI). Patients were also divided according to RV function (patients with or without RV dysfunction, i.e., RV ejection fraction [RVEF] by CMR < 50% or ≥ 50%). Between-group differences were assessed using unpaired Student’s t tests and χ 2 tests. The associations of conventional and STE-derived variables with LVEF by CMR and RVEF by CMR were assessed using Pearson correlation analysis. The diagnostic value of the different parameters was evaluated by receiver operating characteristic (ROC) curves. Cutoff values for sensitivity and specificity evaluation were derived from data collected from healthy subjects (STANISLAS cohort; ClinicalTrials.gov identifier NCT01391442 ) and were defined as all values less than the mean − 2 SDs. In addition, to test the information gain for predicting RV dysfunction when adding strain-derived variables to conventional echocardiographic variables, we compared nested logistic regression models with RVEF by CMR < 50% as the dependent variable. Models including standard echocardiographic variables were compared with models including strain-derived variables in addition to conventional echocardiographic variables using likelihood ratio tests.
Interobserver and intraobserver (i.e., test-retest variability) reproducibility of RV strain measurements was tested by two observers (O.H., C.S.-S.) for 50 patients. All measurements were made by each observer blinded to the measurements of the other observer. Intraclass correlation coefficients (ICCs) were used to explore the variability of strain measurements.
All statistical analyses were performed with SPSS version 21.0 (SPSS, Inc, Chicago, IL). All statistical tests were two sided, and a P value < .05 was considered to indicate statistical significance.
Results
Baseline Characteristics
Baseline population characteristics are listed in Table 1 . The mean age was 55.2 ± 10.6 years, with the majority of patients being men (117 of 135 [87%]). Sixty-two patients (45.9%) had inferior MIs, of whom nine had inferolateral MIs, and 73 patients (54.1%) had anterior MIs, of whom 36 had anterolateral AMIs.
Variable | All patients ( n = 135) | Inferior and inferolateral AMI ( n = 62) | Anterior and anterolateral AMI ( n = 73) | P |
---|---|---|---|---|
Age (y) | 55.2 ± 10.6 | 54.9 ± 9.4 | 55.5 ± 11.5 | .73 |
Men/women | 117 (87%)/18 (13%) | 55 (88.7%)/7 (11.3%) | 62 (84.9%)/11 (15.1%) | .52 |
Culprit lesion | ||||
LCX/Mg/RI | 8/3/1 (8.9%) | 8/3/0 | 0/0/1 | |
RCA | 51 (37.8%) | 51 | — | |
LAD/Dg | 69/3 (53.3%) | — | 72 | |
Maximum total creatine kinase (IU/L) | 2,639 ± 1,763 | 2,264 ± 1,491 | 2,967 ± 19,521 | .02 |
Blood pressure at admission (mm Hg) | ||||
Systolic | 125 ± 20 | 121 ± 20 | 128 ± 20 | .04 |
Diastolic | 74 ± 11 | 71 ± 12 | 77 ± 13 | .004 |
Killip class | ||||
I | 121 (89.6%) | 58 (93.5%) | 63 (86.3%) | .32 |
II | 13 (9.6%) | 4 (6.5%) | 9 (12.3%) | |
III/IV | 1 (0.7%) | 0 (0%) | 1 (1.4%) |
Reperfusion therapy was achieved with a median delay of 180 min (interquartile range, 150–330 min) after symptom onset. The mean peak creatine phosphokinase level was 2,639 ± 1,763 IU/L. Thrombolysis In Myocardial Infarction flow grade 3 was obtained in all patients after PTCA, as required by the inclusion criteria. Most patients had one-vessel disease.
Patients with anterior AMIs had higher peak creatine phosphokinase levels and higher systolic and diastolic blood pressures.
Reproducibility
When considering the interobserver reproducibility of RV strain measurements, ICCs were always >0.80; the mean ICC in the nine segments was 0.9. Lower values were found in apical segments of each wall.
Mean intercycle reproducibility was good, with an ICC > 0.94. Whatever the segment considered, ICCs were always >0.88. Lower values were found in the basal segments of each wall.
Left Ventricular Functional Assessment
CMR and TTE were performed without complications in all patients 2.7 ± 0.8 and 3.1 ± 1.2 days, respectively, after reperfusion therapy. Most of the time, the two examinations were performed consecutively, allowing a median time between CMR and TTE of 155 min (interquartile range, 40–1,440 min). The overall mean LVEF by CMR of our population was 46.5 ± 8.5%. The mean LVEF by TTE was 49.4 ± 8.5%, and mean global LV longitudinal peak systolic strain was −14.3 ± 3.1%. Patients with anterior MIs had a more severely impaired LV systolic function, regardless of the imaging technique ( Table 2 ).
Variable | All patients ( n = 135) | Inferior AMI ( n = 62) | Anterior AMI ( n = 73) | P (inferior vs anterior AMI) |
---|---|---|---|---|
LV function | ||||
CMR | ||||
LVEF (%) | 46.5 ± 8.5 | 49.9 ± 7.5 | 43.6 ± 8.3 | <.0001 |
LVEDVi (mL/m 2 ) | 86.9 ± 14.6 | 85.1 ± 13.2 | 88.4 ± 15.5 | .18 |
TTE | ||||
LVEF (%) | 49.4 ± 8.5 | 52.0 ± 7.4 | 47.2 ± 8.9 | .001 |
LV GLPSS (%) | −14.3 ± 3.1 | −15.8 ± 2.8 | −13.0 ± 2.8 | <.0001 |
RV function | ||||
CMR | ||||
RVEF (%) | 54.8 ± 6.2 | 53.3 ± 6.6 | 56.0 ± 5.4 | .01 |
RVEDVi (mL/m 2 ) | 68.1 ± 12.8 | 72.4 ± 11.0 | 64.4 ± 13.1 | <.0001 |
TTE | ||||
STE-derived parameters | ||||
Lateral strain (%) | −24.5 ± 6.5 | −21.7 ± 6.5 | −26.8 ± 5.5 | <.0001 |
Inferior strain (%) | −21.7 ± 6.5 | −18.7 ± 6.3 | −24.1 ± 5.6 | <.0001 |
Septal strain (%) | −14.6 ± 3.4 | −14.7 ± 3.5 | −14.6 ± 3.4 | .76 |
4C strain (%) | −19.6 ± 4.0 | −18.3 ± 4.1 | −20.6 ± 3.7 | .001 |
Inferolateral strain (%) | −23.2 ± 5.4 | −20.2 ± 5.1 | −25.5 ± 4.4 | <.0001 |
RV GLPSS (%) | −20.4 ± 3.9 | −18.4 ± 3.8 | −21.9 ± 3.3 | <.0001 |
Conventional parameters | ||||
TAPSE (mm) | 21.8 ± 4.5 | 21.6 ± 4.4 | 21.9 ± 4.6 | .71 |
RVMPI | 0.31 ± 0.17 | 0.31 ± 0.17 | 0.31 ± .17 | .88 |
RVFAC (%) | 43.8 ± 9.6 | 39.6 ± 10.5 | 47.4 ± 7.1 | <.0001 |
S′ (cm/sec) | 12.8 ± 2.5 | 12.7 ± 2.2 | 12.8 ± 2.7 | .86 |
TR Vmax (m/sec) | 2.34 ± 0.35 | 2.26 ± 0.34 | 2.41 ± 0.34 | .04 |
Inferolateral RV WMSI | 1.95 ± 0.20 | 1.89 ± 0.30 | 2.00 ± 0.00 | NA |
Global RV WMSI | 1.86 ± 0.22 | 1.91 ± 0.23 | 1.77 ± 0.18 | <.0001 |
Right Ventricular Functional Assessment
Comparison between Inferior and Anterior MI
The mean RVEF by CMR was 54.8 ± 6.2% ( Table 2 ). There were 20 patients with RVEF by CMR < 50%, of whom 12 (19%) had inferior MIs and eight (11%) had anterior MIs. RVEF by CMR was lower in patients with inferior MIs compared with those with anterior MIs (mean, 53.3 ± 6.6% vs 56.0 ± 5.4%, P = .01). Among the 20 patients with RV dysfunction, mean RVEF by CMR was not significantly different between patients with inferior and anterior AMIs (43.4 ± 5.5% vs 46.5 ± 1.8%, P = .15). RV end-diastolic volume index was markedly higher in patients with inferior MIs (72.4 ± 11.0 vs 64.4 ± 13.1 mL/m 2 , P < .0001). Among the conventional echocardiographic parameters of RV function, only RVFAC and global RV WMSI differed between the two populations. In contrast, all STE-derived parameters except septal strain were significantly decreased in patients with inferior MIs (e.g., RV GLPSS , −18.4 ± 3.8 vs −20.5 ± 3.7%, P < .0001). RV afterload, estimated by tricuspid regurgitation peak velocity, was significantly higher in patients with anterior MIs (2.26 ± 0.34 vs 2.41 ± 0.34 m/sec, P = .04). Inferior wall abnormal contractility by visual analysis was found in 10 patients, of whom five also had RV lateral wall abnormal contractility. Among these 10 patients, all had inferior MIs. Septal contractility was altered in 61 patients.
Echocardiographic Variables in Patients with or without RV Dysfunction
Among the conventional parameters, RVFAC was significantly decreased in patients with versus those without RV dysfunction (35.7 ± 11.0 vs 45.3 ± 8.7, P < .0001). Among STE-derived parameters, all but septal strain were significantly different between patients with and those without RV dysfunction ( P ≤ .001 for all) ( Supplemental Table 1 ).
Correlations between Echocardiographic and CMR Parameters
In the whole population, inferolateral RV WMSI and RVFAC were the best correlates of RVEF by CMR among conventional parameters ( Table 3 ). Nevertheless, correlations were weak ( r = 0.407 and r = 0.368, respectively, P < .0001 for both). As a reminder, RVFAC was obtained in a single-plane approach from the 4C view. Conversely, two STE-derived variables demonstrated correlations with RVEF by CMR > −0.45 (inferolateral strain, r = −0.450, P < 0.0001; and RV GLPSS, r = −0.459, P < .0001).
Variable | Correlation (Pearson) | All patients | P | Inferior AMI | P |
---|---|---|---|---|---|
LVEF by CMR | LVEF by TTE | 0.723 | <.0001 | 0.689 | <.0001 |
LV GLPSS | −0.722 | <.0001 | −0.690 | <.0001 | |
RVEF by CMR | 0.420 | <.0001 | 0.577 | <.0001 | |
RVEF by CMR | |||||
STE-derived parameters | Lateral strain | −0.353 | <.0001 | −0.459 | <.0001 |
Inferior strain | −0.368 | <.0001 | −0.389 | .003 | |
Septal strain | −0.168 | .06 | −0.229 | .08 | |
4C strain | −0.354 | <.0001 | −0.460 | <.0001 | |
Inferolateral strain | −0.450 | <.0001 | −0.559 | <.0001 | |
RV GLPSS | −0.459 | <.0001 | −0.581 | <.0001 | |
Conventional parameters | TAPSE | 0.267 | .002 | 0.300 | .02 |
RVMPI | −0.279 | .002 | −0.336 | .01 | |
RVFAC | 0.368 | <.0001 | 0.388 | .002 | |
S′ | 0.180 | .04 | 0.277 | .03 | |
Inferolateral RV WMSI | 0.407 | <.0001 | 0.515 | <.0001 | |
Global RV WMSI | 0.262 | .003 | 0.505 | <.0001 |
The correlations between STE-derived variables and RVEF by CMR were higher in patients with inferior AMIs than in those with anterior AMIs ( Table 3 and Supplemental Table 2 ). Of note, in patients with inferior AMIs, RV GLPSS was still the best correlate of RVEF by CMR ( r = −0.581, P < .0001).
As a sensitivity analysis, we evaluated correlations between RVEF by CMR and transthoracic echocardiographic parameters according to the delay between the two imaging techniques. Whatever the delay between CMR and transthoracic echocardiographic assessments, STE-derived parameters (especially RV GLPSS and inferolateral strain) remained better correlates of RVEF by CMR than conventional parameters ( Supplemental Table 3 ).
Diagnostic Value of Echocardiographic and CMR Parameters
The accuracy of echocardiographic parameters in discriminating patients with normal and those with altered RV function (RVEF by CMR < 50% or ≥ 50%) was evaluated by ROC curve analysis ( Table 4 , Figure 2 ). Cutoff values were derived from healthy subjects and are presented in Supplemental Table 4 . Among conventional variables, RVFAC had the highest area under the ROC curve (AUROC) (0.756; 95% CI, 0.647–0.866) in the whole population. All other conventional parameters had AUROCs < 0.70. RV myocardial performance index and RV WMSI had the lowest AUROCs.
All patients | Inferior AMI | |||||
---|---|---|---|---|---|---|
AUROC (%) | 95% CI | P | AUROC (%) | 95% CI | P | |
STE-derived parameters | ||||||
Lateral strain | 0.748 | 0.621−0.874 | <.001 | 0.861 | 0.763−0.959 | <.001 |
Inferior strain | 0.697 | 0.558−0.836 | .006 | 0.752 | 0.595−0.908 | .01 |
Septal strain | 0.534 | 0.378−0.691 | .62 | 0.561 | 0.365−0.758 | .51 |
4C strain | 0.709 | 0.578−0.839 | .003 | 0.789 | 0.633−0.945 | .002 |
Inferolateral strain | 0.753 | 0.623−0.883 | <.001 | 0.877 | 0.775−0.980 | <.001 |
RV GLPSS | 0.724 | 0.590−0.857 | .002 | 0.825 | 0.675−0.974 | .001 |
Conventional parameters | ||||||
TAPSE | 0.652 | 0.501−0.803 | .03 | 0.778 | 0.630−0.926 | .003 |
RVMPI | 0.602 | 0.450−0.755 | .18 | 0.622 | 0.409−0.834 | .23 |
RVFAC | 0.756 | 0.647−0.866 | <.001 | 0.760 | 0.611−0.909 | .006 |
S′ | 0.689 | 0.573−0.804 | .007 | 0.728 | 0.581−0.875 | .02 |
Inferolateral RV WMSI | 0.607 | 0.457−0.756 | .13 | 0.671 | 0.476−0.866 | .07 |
Global RV WMSI | 0.605 | 0.449−0.761 | .13 | 0.658 | 0.459−0.858 | .09 |
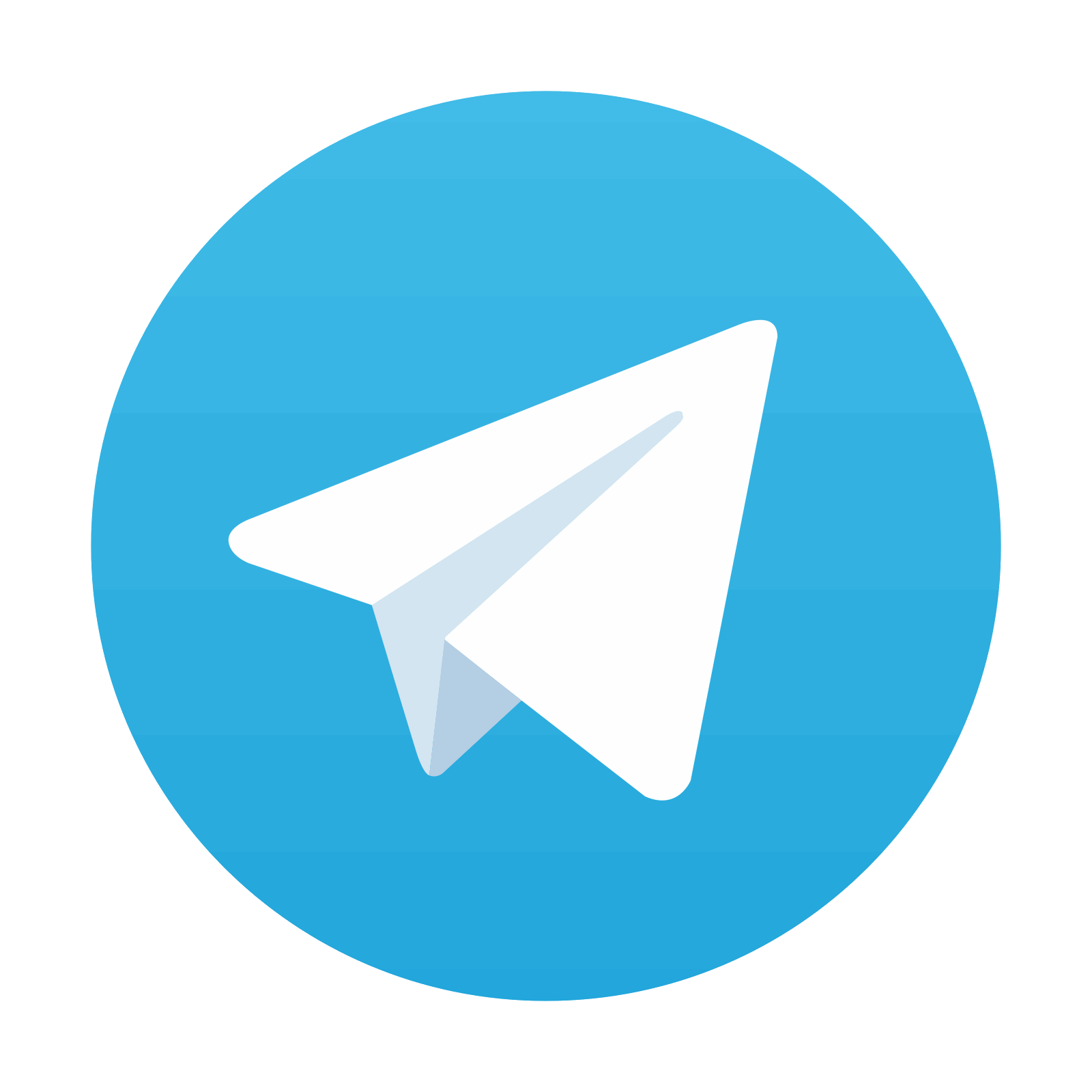
Stay updated, free articles. Join our Telegram channel

Full access? Get Clinical Tree
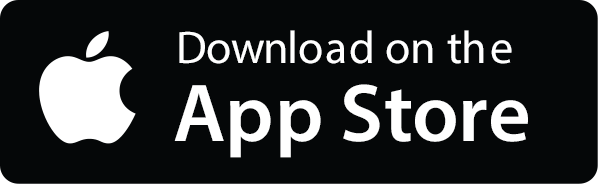
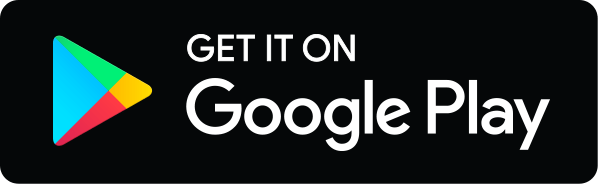
