Left ventricular (LV) mass and geometry are associated with risk of cardiovascular disease (CVD). We sought to determine whether LV mass and geometry contribute to risk prediction for CVD in adults aged ≥65 years of the Cardiovascular Health Study. We indexed LV mass to body size, denoted as LV mass index (echo-LVMI), and we defined LV geometry as normal, concentric remodeling, and eccentric or concentric LV hypertrophy. We added echo-LVMI and LV geometry to separate 10-year risk prediction models containing traditional risk factors and determined the net reclassification improvement (NRI) for incident coronary heart disease (CHD), CVD (CHD, heart failure [HF], and stroke), and HF alone. Over 10 years of follow-up in 2,577 participants (64% women, 15% black, mean age 72 years) for CHD and CVD, the adjusted hazards ratios for a 1-SD higher echo-LVMI were 1.25 (95% CI 1.14 to 1.37), 1.24 (1.15 to 1.33), and 1.51 (1.40 to 1.62), respectively. Addition of echo-LVMI to the standard model for CHD resulted in an event NRI of −0.011 (95% CI −0.037 to 0.028) and nonevent NRI of 0.034 (95% CI 0.008 to 0.076). Addition of echo-LVMI and LV geometry to the standard model for CVD resulted in an event NRI of 0.013 (95% CI −0.0335 to 0.0311) and a nonevent NRI of 0.043 (95% CI 0.011 to 0.09). The nonevent NRI was also significant with addition of echo-LVMI for HF risk prediction (0.10, 95% CI 0.057 to 0.16). In conclusion, in adults aged ≥65 years, echo-LVMI improved risk prediction for CHD, CVD, and HF, driven primarily by improved reclassification of nonevents.
Left ventricular (LV) hypertrophy is an independent predictor and potentially modifiable risk factor for incident cardiovascular disease (CVD). Although previous data demonstrate a strong association between LV mass (LVM)/hypertrophy and increased cardiovascular risk, other statistical methods may be more useful in determining the utility of a biomarker to aid in clinical decision making. Net reclassification improvement (NRI) determines the number of subjects who are appropriately reclassified into higher or lower risk with addition of a new biomarker to an existing risk algorithm. LVM tends to increase, and LV hypertrophy becomes more prevalent with increasing age, so these measurements may be particularly important for risk assessment. We sought to determine the extent to which LVM and geometry determined by echocardiography and electrocardiography improves risk prediction for coronary heart disease (CHD), heart failure (HF), stroke, and global CVD outcomes, beyond models based on traditional risk factors.
Methods
The Cardiovascular Health Study (CHS) is a prospective study sponsored by the National Institutes of Health; details of the study design have been previously published. Participants were recruited from the following 4 communities: Washington County, Maryland; Pittsburgh, Pennsylvania; Forsyth County, North Carolina; and Sacramento County, California. CHS includes 5,201 community-dwelling men and women aged ≥65 years, recruited in 1989 to 1990; an additional cohort of 687 African-Americans was recruited from 1992 to 1993. Echocardiograms were recorded in 1989 to 1990 for the original cohort and 1994 to 1995 for the African-American cohort. In the present analysis, “baseline” refers to the 1989 to 1990 examination for the initial cohort and the 1994 to 1995 examination for the second cohort. Figure 1 shows the exclusion criteria for the CHD and CVD analyses, which we refer to as cohort A. For the CHD and CVD analyses, we excluded participants who had a previous diagnosis of CHD, HF, stroke, or atrial fibrillation (total with clinical CVD = 1,529), missing indexed echo LVM (n = 1,486), missing key covariates at the baseline examination (n = 51), and moderate or severe valvular stenosis or regurgitation (n = 245). After exclusions, 2,577 participants were available for analyses.
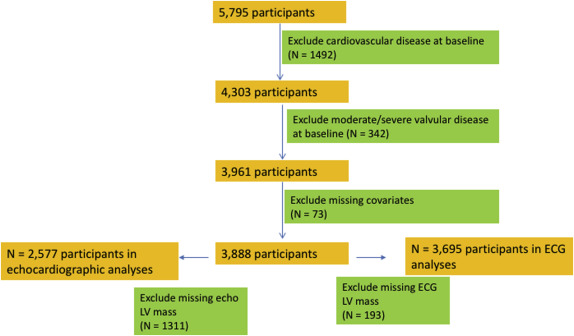
Figure 2 shows the exclusion criteria for the HF analyses, which we refer to as cohort B. In cohort B, we used inclusion criteria that are similar to those previously described by Butler et al in the Health ABC Study. We excluded participants with HF at baseline (n = 297), missing indexed echo-LVM (n = 1,958), and other missing key covariates or baseline data (n = 82). The final cohort B included 3,551 participants. We then conducted a sensitivity analysis for the HF outcome after additionally excluding participants with LV ejection fraction <45% (n = 75). Of note, unlike cohort A, cohort B does not exclude participants with a history of CHD and stroke.
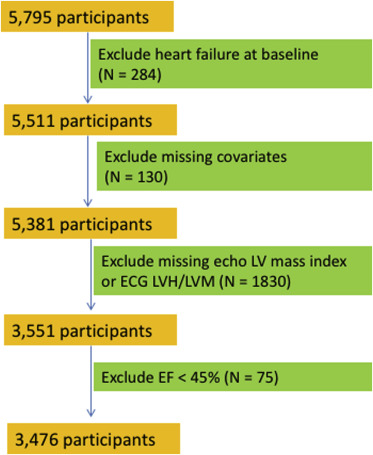
Electrocardiographic left ventricular hypertrophy (ECG-LVH) was considered present if the following Minnesota Codes were present: 3-1, 3-3, 4-1 to 4-3, and 5-1 to 5-3. ECG-LVM was calculated according to the race- and sex-specific formulas and adjusted for body size, as described by Rautaharju et al previously in CHS. Echo-LVM was calculated from 2-dimensionally guided M-mode echocardiograms using a method that has been described in detail previously. We used the following formula described by Devereux to calculate the unadjusted LV mass: LV mass (grams) = 0.80 × 1.04 × ([VSTd + LVIDd + PWTd] 3 − [LVIDd] 3 ) + 0.6; where VSTd refers to the ventricular septal thickness in end diastole, LVIDd refers to LV internal diameter in end diastole, and PWTd refers to the posterior wall thickness in end diastole.
The echo-LVM value obtained from the Devereux equation was adjusted for height, weight, and gender based on a method previously described from the Multi-Ethnic Study of Atherosclerosis. A healthy subgroup (without a history of coronary disease, HF, stroke, hypertension, diabetes, obesity, or significant subclinical disease by carotid ultrasound and ankle-brachial index) within CHS was used to define reference equations for LVM and wall thickness, adjusted for height, weight, and gender. We used a linear regression model with log-transformed LVM as the outcome and gender and log-transformed height and weight as the predictors. We then defined the indexed value of the echo-LV mass (echo-LVMI) for the entire population using the coefficients from the healthy participants. We divided LV mass by height raised to the power of the height coefficient, weight raised to the power of the weight coefficient, and the exponentiated intercept for men and the exponentiated sum of the intercept and gender coefficient for women. Indexed wall thickness was created similarly with log-transformed relative wall thickness (RWT) as the outcome variable. We determined the cutoff for LVH to be 1.44 and the cutoff for increased RWT to be 1.31 based on the 95th percentile of these measures on echos from healthy CHS participants. LV geometry was defined as follows: normal: normal LVMI, normal RWT; concentric remodeling: normal LVMI, increased RWT; eccentric LVH: increased LVMI, normal RWT; or concentric hypertrophy: increased LVMI, increased RWT.
Based on the risk prediction model developed by D’Agostino, we used the following covariates from the baseline examination for modeling risk associated with CVD, CHD, and stroke: age, gender, race, systolic blood pressure, diabetes status, antihypertensive medication, and smoking status (current vs not). Based on the HF risk prediction model developed by Butler, we used the following covariates from the baseline examination for modeling risk associated with HF: age, gender, race, systolic blood pressure, heart rate, smoking status (current, former, and never), albumin, fasting glucose, creatinine, and prevalent CHD. Changes to the risk factors in the models established by Butler are that we added race and gender; we also removed ECG-LVH from the Butler model to evaluate risks associated with echo parameters. Total and high-density lipoprotein cholesterol, albumin, creatinine, and fasting glucose were taken from CHS baseline measurements (1992 to 1993 for the African-American cohort). Missing data on systolic blood pressure, diabetes status, antihypertensive medication, heart rate, and smoking status for the second cohort were imputed with values carried forward from the previous 2 years, when available. Participants were classified as having diabetes mellitus if any of the following conditions were met: (1) use of insulin or an oral hypoglycemic agent; (2) fasting glucose level of ≥7 mmol/L (126 mg/dl); or (3) a nonfasting glucose level of ≥11.1 mmol/L (200 mg/dl).
Outcomes were adjudicated by trained physicians, as has been described previously. The CHS Events Committee is composed of a panel of trained physicians and adjudicated all clinical outcomes. Myocardial infarction was defined based on a combination of clinical history, ECG abnormalities, and cardiac enzymes. The Events Committee classified all deaths into 1 of the following 5 groups: (1) atherosclerotic CHD; (2) cerebrovascular disease; (3) atherosclerotic disease other than CHD (e.g., abdominal aortic aneurysm or ischemic bowel) ; other CVD (such as valvular heart disease or pulmonary embolism); and all other deaths. Only group 1 was included for the CHD outcome and groups 1, 2, 3, and 4 were included for the CVD outcome. Cerebrovascular disease was defined on the basis of clinical symptoms of stroke and imaging findings; both fatal and nonfatal stroke were included in the CVD outcome. We also analyzed HF and stroke separately; for the HF analyses, we excluded participants who had LV ejection fraction <45% at the baseline examination as a secondary analysis (n = 75).
Covariates were described as the mean and SD for continuous variables and as n (%) for dichotomous variables, stratified by echo-LVH. To use previously validated risk categories for CHD and CVD, our follow-up time was 10 years. We used Cox proportional hazards regression to calculate 10-year predicted risk for CHD, HF, stroke, and all CVD using CHS-specific coefficients for traditional risk factors as previously described. The exclusion criteria are shown in Figure 1 for CHD, CVD, and stroke and Figure 2 for HF. Subjects were censored for lack of follow-up, death, or at 10 years. We evaluated the addition of echo-LVMI (denoted continuous echo-LVMI, model 2), ECG-LVM (model 3), echo-LVMI plus LV geometry (model 4), and echo-LVH (model 5) to the base model. The ECG analyses included participants with complete ECG data, even if echocardiographic data were incomplete, so the sample size is greater for the ECG analyses. We tested the proportional hazards assumption using Schoenfeld residuals and found no violations. To assess the discrimination of the models to classify subjects according to event status, we calculated the C-statistic and compared each model to the base model.
We also evaluated the risk classification of these models with NRI using the following categories of predicted risk: <10%, 10% to 20%, and ≥20% for CHD similar to previous risk assessment frameworks. The risk categories for all CVD were <20%, 20% to 30%, and ≥30%, and <5%, 5% to 10%, 10% to 20%, and ≥20% for HF and stroke separately. The categorical NRI incorporates the user-defined categories and only counts a participant if they are reclassified into a different risk group and was calculated separately for those who experience an event and those who did not. The event NRI was calculated as ([number of events reclassified higher − number of events reclassified lower]/number of events), and similarly the nonevent NRI was calculated as ([number of nonevents reclassified lower − number of nonevents reclassified higher]/number of nonevents). Due to recent concerns about the validity of the combined categorical NRI, we report the event and nonevent NRI separately with bootstrapped CIs. We reported the continuous NRI which incorporates both events and nonevents as has been done in previous studies and to facilitate comparisons of different biomarkers.
Sensitivity analyses included repeating the previously mentioned analyses with further exclusion of those with ejection fraction <45% (n = 75) for the HF outcome. Statistical significance was established at a 2-sided p value <0.05. Analyses were performed using Stata, version 12.1 (Stata Corp, College Station, Texas).
Results
Among the 2,577 participants with complete indexed echo data in cohort A, 11.8% (n = 305) had echo-LVH. As listed in Table 1 , participants with LVH were older, more likely to be men, and less likely to be black. Multivariable-adjusted hazards ratios for incident CHD and incident total CVD are listed in Table 2 . For a 1-SD increase in echo-LVMI, the hazards ratios for CHD and CVD were 1.25 (95% CI 1.14 to 1.37, p <0.001) and 1.24 (1.15 to 1.33, p <0.001), respectively. The hazards ratios for CHD and CVD for a 1-SD increase in ECG-LVM were more modest than for echo-LVMI but remained statistically significant. Table 2 also lists hazards ratios for incident CHD and CVD as a function of LV geometry. CHS participants with concentric remodeling and eccentric LVH were significantly more likely to experience CHD and CVD during the follow-up period. Participants with concentric LVH had an almost twofold greater risk for CHD than those with normal geometry.
Characteristic | No LVH (N = 2272) | LVH (N = 305) |
---|---|---|
Age (years) | 71.9 (5.0) | 73.2 (5.6) |
Black race (N, %) | 342 (15.1%) | 39 (12.8%) |
Male sex (N, %) | 779 (34.3%) | 160 (52.5%) |
Total cholesterol (mg/dl) | 215.2 (38.7) | 204.5 (35.6) |
Systolic blood pressure (mm Hg) | 135 (20) | 143 (24) |
High-density lipoprotein cholesterol (mg/dl) | 56.6 (15.8) | 54.0 (15.8) |
Electrocardiographic left ventricular mass (g) | 147.1 (27.4) | 164.1 (39.4) |
Echocardiographic left ventricular mass (g) | 134.7 (33.3) | 224.7 (49.8) |
Anti-hypertensive medication (N, %) | 798 (35.1%) | 146 (47.9%) |
Current smoker (N, %) | 262 (11.5%) | 47 (15.4%) |
Diabetes mellitus (N, %) | 264 (11.6%) | 50 (16.4%) |
Parameter | Coronary Heart Disease | Cardiovascular Disease | Heart Failure |
---|---|---|---|
Echo-LVH (compared to no LVH) | 1.47 (1.17-1.85) ∗ | 1.50 (1.24-1.81) ∗ | 2.57 (2.14-3.08) ∗ |
Echo-LVMI (per 1-SD increase) | 1.24 (1.14-1.37) ∗ | 1.26 (1.15-1.33) ∗ | 1.52 (1.41-1.63) ∗ |
ECG-LVM (per 1-SD increase) | 1.14 (1.04-1.25) ∗ | 1.15 (1.07-1.24) ∗ | 1.38 (1.30-1.47) ∗ |
GEOMETRY | |||
Normal geometry | 1.0 (ref) | 1.0 (ref) | 1.0 (ref) |
Concentric remodeling | 1.47 (1.03-2.09) ∗ | 1.37 (1.02-1.85) ∗ | 1.24 (0.86-1.78) |
Eccentric LVH | 1.44 (1.12-1.85) ∗ | 1.54 (1.26-1.89) ∗ | 2.67 (2.20-3.23) ∗ |
Concentric LVH | 1.95 (1.17-3.24) ∗ | 1.47 (0.93-2.31) | 2.24 (1.43-3.50) ∗ |
Table 3 lists the cross tabulations for observed and predicted CHD events with and without continuous echo-LVMI. The C-statistics for the baseline model and with the addition of echo-LVMI were 0.654 and 0.661, respectively (p value for difference = 0.09). We found that addition of echo-LVMI reclassified 13.6% (n = 351) of participants. The continuous NRI was 0.12 (p = 0.01), the categorical NRI for CHD events was 0.011 (bootstrapped 95% CI −0.037 to 0.028), and the categorical NRI for nonevents was 0.034 (bootstrapped 95% CI 0.008 to 0.076). With the addition of categorical echo-LVH to the model based on traditional risk factors, we observed that the continuous NRI was 0.10 (p = 0.03), and the categorical NRI for events and nonevents was not significant.
10-year risk in model with echocardiographic left ventricular mass index added to standard model | ||||
---|---|---|---|---|
10-year risk with standard model | < 10% | 10-20% | ≥ 20% | Total |
< 10% | 91 | 22 | 0 | 113 |
Cases (N, %) | 10 (11%) | 1 (5%) | 0 | 11 (10%) |
10-20% | 60 | 922 | 116 | 1098 |
Cases (N, %) | 5 (8%) | 108 (12%) | 27 (23%) | 140 (13%) |
≥ 20% | 0 | 153 | 1213 | 1366 |
Cases | 0 | 29 (19%) | 360 (30%) | 389 (28%) |
TOTAL (Events, %) | 151 (15, 10%) | 1097 (138, 13%) | 1329 (387, 29%) | 2577 (540, 21%) |
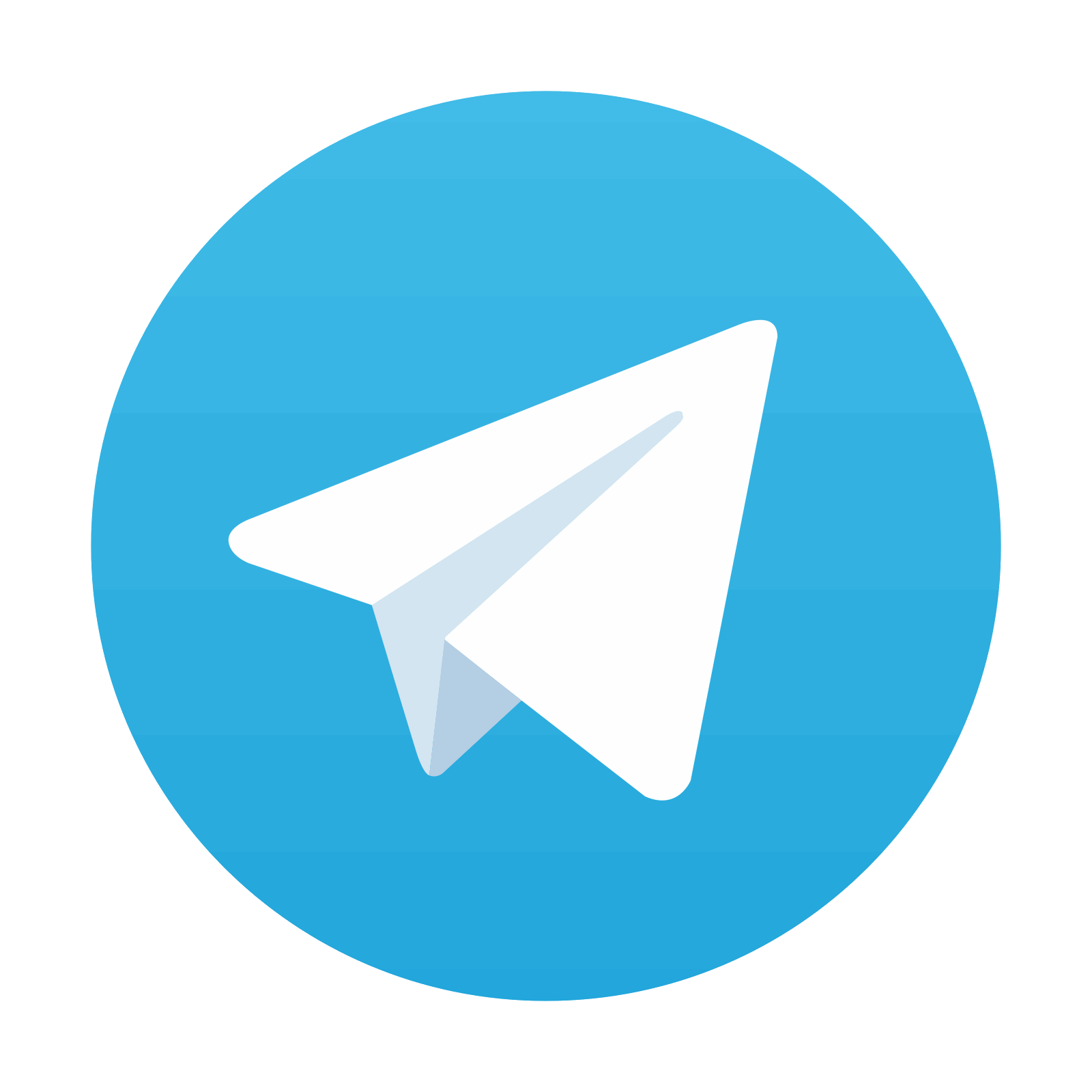
Stay updated, free articles. Join our Telegram channel

Full access? Get Clinical Tree
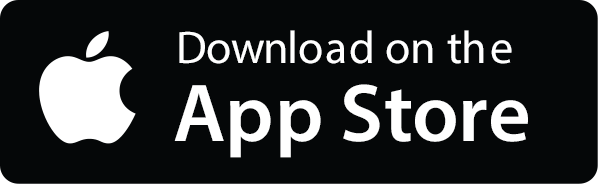
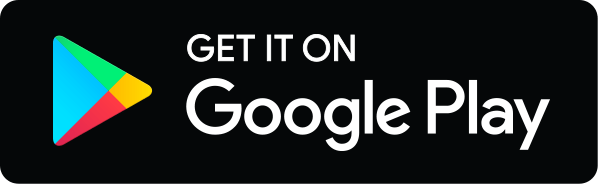
