Cardiopulmonary exercise testing (CPX) might aid in the diagnosis of coronary artery disease. However, a heterogeneous clinical population without previous workup bias has not been studied nor has a more extensive list of CPX variables. A total of 303 subjects (age 49.9 ± 11.6 years, 157 men) with symptoms suggestive of coronary artery disease underwent CPX and a single photon emission computed tomographic myocardial perfusion study (MPS). Ventilatory efficiency was calculated using the oxygen uptake efficiency slope (OUES). The change in the OUES was calculated by subtracting the OUES response during the first 50% of CPX from the OUES obtained during the last 25% of CPX. A negative change in the OUES (<0) from the first 50% to the last 25% of CPX was predictive of positive MPS findings only in the male subjects. The diagnostic significance of the change in OUES in men was found for any level (including equivocal studies) of positive MPS findings (area under the curve 0.67, 95% confidence interval 0.59 to 0.76, p <0.0001) and was even stronger in those with a more definitive (excluding equivocal studies) perfusion defect (area under the curve 0.76, 95% confidence interval 0.67 to 0.85; relative risk 5.4, 95% confidence interval 2.1 to 13.8, p <0.0001). In conclusion, this is the first time that a change in ventilatory efficiency, assessed using the OUES, has been shown to be predictive of positive MPS findings However, the OUES change only provided diagnostic information for men, a finding that warrants additional analysis.
Currently, only one published investigation has focused on the ability of cardiopulmonary exercise testing (CPX) variables to detect exercise-induced myocardial ischemia in a large cohort (i.e., >200 subjects). Belardinelli et al prospectively studied 202 patients (86% men) with confirmed coronary artery disease (CAD) and compared the results of CPX and the traditional exercise test variables to those of myocardial perfusion studies (MPSs). The independent predictors of a reversible perfusion defect were the duration of oxygen pulse flattening (measured as the interval between the occurrence of the maximum oxygen pulse and the total exercise time) and flattening of oxygen consumption–work rate slope. Although promising, additional research is needed to more clearly elucidate the value of CPX in the evaluation of those with suspected CAD. To date, research has included subjects who were either exclusively or predominantly diagnosed with CAD, introducing an evaluation bias as a potential confounding factor. Several large studies have confirmed that in today’s clinical environment, the patient population referred for exercise testing is more heterogeneous, with most at low risk and presenting with signs and/or symptoms merely suggestive of CAD. In addition, a comprehensive assessment of all potentially valuable CPX variables in the diagnosis of exercise-induced myocardial ischemia has not been performed. For example, abnormal changes in the trajectory of ventilatory efficiency might prove valuable. The most common expressions of ventilatory efficiency are the minute ventilation/carbon dioxide production (VE/VCO 2 ) slope and the oxygen uptake efficiency slope (OUES). Given these gaps in the published data, the objectives of the present study were to assess the diagnostic value of CPX in a group of subjects undergoing clinical assessment for CAD without a previous evaluation bias and to more comprehensively assess the utility of all relevant CPX variables in identifying reversible myocardial perfusion defects quantified by nuclear imaging.
Methods
The present investigation prospectively assessed 303 patients who had presented to the noninvasive stress laboratory of Virginia Commonwealth University Medical Center from May 2009 to February 2010. The subjects had presented with symptoms suggestive of myocardial ischemia and/or a history of CAD. Common indications included chest pain (65.3%), dyspnea (8%), a history of CAD (5%), syncope (1.3%), palpitations (3%), and/or abnormal electrocardiographic findings (2.4%). The exclusion criteria were myocardial infarction or percutaneous coronary intervention within 3 months of testing, a previous diagnosis of congestive heart failure, a known left ventricular ejection fraction of <35%, evidence of moderate lung disease by pulmonary function testing, moderate or severe aortic or mitral valve stenosis, unstable angina or uncontrolled hypertension, previous pacemaker implantation or coronary artery bypass grafting, orthopedic/neurologic conditions that limited exercise performance, and/or an inability to collect interpretable electrocardiographic and nuclear imaging data. The traditional cardiac risk factors (hypertension, lipid profile, tobacco use, family history of early coronary disease, physical activity, and diabetes) were recorded. Physical activity frequency, duration, and intensity were measured by patient self-report. The institutional review board at Virginia Commonwealth University approved the protocol. All subjects provided written informed consent before inclusion in the study.
All subjects underwent an at rest and a stress single photon emission computed tomographic MPS in conjunction with the standard exercise test with ventilatory expired gas analysis. All single photon emission computed tomographic MPSs were done with electrocardiographic gating (16 time frames in the heart cycle) to allow for determination of global and segmental left ventricular function and detection of perfusion abnormalities.
Each result was categorized as the presence and extent of reversibility according to the following: 0 or normal, no evidence of attenuation or reversibility or fixed defect likely due to soft tissue attenuation; 1 or equivocal, small size and low-grade reversible defect; 2 or mildly abnormal, small size and moderate grade or moderate size and low-grade reversible defect; 3 or moderately abnormal, moderate size and moderate-grade reversible defect; and 4 or severely abnormal, moderate size and high-grade or large size and high-grade reversible defect.
All exercise tests were done using a motorized treadmill (GE Healthcare Series 2000, Waukesha, Wisconsin) using the Bruce protocol. The exercise testing procedures outlined by the American Heart Association were followed for all assessments. All patients were continuously monitored with 12-lead electrocardiography (GE Marquette 12SL, Waukesha, Wisconsin). Blood pressure was measured using an automated sphygmomanometer (SunTech Tango+, Morrisville, NC), with auditory confirmation at the end of each stage. Age-predicted maximum heart rate was calculated as follows: age-predicted maximum heart rate = 220 − age. The following exercise test termination criteria were used: onset of severe typical angina, arrhythmias, hypotension, dyspnea, intermittent claudication, marked hypertension, >2 mm of horizontal or downsloping ST-segment depression or ST-segment elevation of ≥1 mm, and patient request to stop or an inability to keep up with the treadmill.
Dyspnea and angina were measured using 4-point scales. For the level of exertion, the 6 to 20 Borg Rating of Perceived Exertion scale was used.
Ventilatory expired gas was collected for each test using a metabolic cart (Vmax Encore, SensorMedics, Yorba Linda, California). Before each test, the equipment was calibrated in standard fashion using reference gases and a 3-L syringe. Ventilatory expired gas analysis data collection began at rest and continued throughout the duration of the test. The first minute was used to familiarize the subject with the equipment, to normalize the ventilatory pattern (respiratory exchange ratio target <0.90), and to allow the collection of baseline data. A minimum of 1 minute of baseline data were collected once the respiratory exchange ratio target had been achieved.
VE, oxygen consumption, and VCO 2 were acquired breath-by-breath and averaged for 10-second intervals. The peak oxygen consumption was expressed as the greatest 30-second averaged sample obtained during the exercise test in ml O 2 · kg −1 · min −1 . The peak respiratory exchange ratio was expressed as the greatest averaged sample obtained during the exercise test. For the OUES, VE, averaged for 10-second intervals, was transformed into its logarithmic equivalent. The OUES was determined using least-squares linear regression analysis (oxygen consumption = a log 10 VE + b, with oxygen consumption and VE expressed in liters per minute) using spreadsheet software (Microsoft Excel, Microsoft, Bellevue, Washington). All exercise data were used to calculate the OUES. The change in the OUES was calculated by subtracting the OUES response during the first 50% of the CPX from the OUES obtained during the last 25% of CPX (OUES50-75). VE and VCO 2 values, acquired from the initiation of exercise to peak, were entered into the spreadsheet software (Microsoft Excel) to calculate the VE/VCO 2 slope using least squares linear regression analysis (y = mx + b, with m = slope). A change in the VE/VCO 2 slope (VE/VCO 2 50-75) was assessed in the same manner as described for OUES. The oxygen pulse was calculated in 30-second intervals by dividing the oxygen consumption by the heart rate at rest and throughout exercise. The peak oxygen pulse was the greatest 30-second averaged value during the exercise bout. The oxygen pulse flattening duration, initially described by Belardinelli et al, was calculated as the total exercise time minus the time at which the peak oxygen pulse occurred. The change in oxygen pulse was calculated as the difference between the greatest oxygen pulse during exercise and the value at rest.
Statistical analysis was performed using the Statistical Package for Social Sciences, version 17.0 (SPSS, Chicago, Illinois). All values are expressed as the mean ± SD. Unpaired Student’s t test was used to compare continuous variables of interest between subjects. One-way analysis of variance was used to compare the clinical, hemodynamic, and metabolic interval data among the groups of subjects stratified according to the 3 levels of MPS results (negative, equivocal, and mild to severe). When a significant difference was detected by analysis of variance, a post hoc analysis was performed using the Tukey test. The Kruskal-Wallis test was used to compare the differences in ordinal data (i.e., rating of perceived exertion, dyspnea, angina scales) and chi-square analysis to assess differences in nominal data (i.e., gender, race) among the 3 groups. Receiver operator characteristic curve analysis was used to assess the predictive ability of key electrocardiographic stress test and CPX variables. When an area under the curve was significant and an optimal threshold value was identified, a 2-by-2 contingency table was constructed to determine the relative risk ratio and 95% confidence interval of that given threshold. Because of the known effect of β-blocking agents on the heart rate response during exercise, a subgroup analysis was performed on the diagnostic utility of key variables in subjects who had not been prescribed a β-blocking agent. Finally, the ability of the standard exercise test and ventilatory expired gas variables to identify subjects with a reversible myocardial perfusion defect was performed separately for men and women. All statistical tests with p <0.05 were considered significant.
Results
A total of 303 subjects (157 men and 146 women) successfully completed all assessment procedures. Most subjects (86.1%) had no history of heart disease; 10% of subjects had a history of CAD (8.9% had undergone previous percutaneous coronary intervention and 1.1% had had a myocardial infarction without intervention). Electrical abnormalities and mild valvular disorders were present in 3.9% of the subjects. Most (73.6%) were taking prescribed medications on the day of the test. Of these, slightly more than ½ (55.0%) were taking an antihypertensive drug, 31.4% were taking a lipid-lowering agent, 28.1% were taking aspirin, and 11.2% were taking a medication for diabetes. More than ½ (57.4%) reported no regular physical activity. Another 27.7% reported exercising <3 days per week, and only 14.8% reported participation in exercise on ≥4 days per week. The mean number of cardiovascular risk factors was 3.0 ± 1.5.
The heart rate at rest was not different between the men and women (72.2 ± 15.0 vs 74.8 ± 14.1 beats/min, respectively; p = 0.11). However, the blood pressure at rest was significantly greater in the men than in the women (137.3/84.1 ± 21.4/13.1 vs 130.8/80.7 ± 18.6/9.4 mm Hg, respectively; p <0.05).
Of the 303 subjects, 202 (66.7%) had normal MPS findings, 69 (22.8%) had equivocal findings, and 32 (10.5%) had mildly, moderately, or severely abnormal stress MPS findings. Of those with an abnormal result, the percentage of myocardium involved was 1% to 14% (mean 4.39 ± 3.31%). Most of the defects were small (72.5%) and low grade (84.2%). Wall motion abnormalities were present in 7.6% of those with abnormal stress MPS findings. The frequency of negative, equivocal, and positive MPS findings was different among men and women (93, 42, and 22 vs 109, 27, and 10, respectively; p <0.01).
No difference was seen in age between each of the MPS groups ( Table 1 ). A greater percentage of men were in the mild to severe group and the equivocal MPS group than in the negative group. Patients with a mild to severe defect had a significantly greater body mass index than the negative group and more risk factors than either the equivocal or negative group. Patients in the mild to severe group were more likely to have typical chest pain or shortness of breath than those in the equivocal or negative group. The treadmill time was significantly less in the mild to severe group than in the negative perfusion group and was significantly less in the equivocal group than in the mild to severe group. Finally, OUES50-75 was significantly different in the mild to severe perfusion defect group compared to that in the negative group.
Variable | Negative (n = 202) | Equivocal (n = 69) | Mild to Severe (n = 32) |
---|---|---|---|
Age (years) | 49.5 ± 10.8 | 50.23 ± 13.1 | 51.8 ± 12.6 |
Men | 46% | 61% ⁎ | 69% † |
Body mass index (kg/m 2 ) | 29.5 ± 6.2 | 31.5 ± 6.2 | 33.7 ± 7.8 † |
Number of risk factors | 2.9 ± 1.5 | 3.2 ± 1.4 ‡ | 3.7 ± 1.5 † |
Coronary artery disease | 6.9% | 14.5% ⁎ | 21.9% † |
Chief complaint | |||
Atypical chest pain | 67.2% | 66.1% | 51.6% † |
Typical chest pain | 14.0% | 13.2% | 25.8% † |
Dyspnea | 8.5% | 4.4% ‡ | 13.0% † |
At rest hemodynamic data | |||
Heart rate (beats/min) | 73.6 ± 14.4 | 71.6 ± 14.5 | 76.6 ± 14.5 |
Systolic blood pressure (mm Hg) | 133.3 ± 18.9 | 135.1 ± 17.2 | 137.7 ± 32.0 |
Diastolic blood pressure (mm Hg) | 82.6 ± 10.7 | 82.1 ± 9.9 | 82.5 ± 11.6 |
Exercise hemodynamic and ventilatory data | |||
Maximum heart rate (beats/min) | 152.0 ± 18.9 | 145.5 ± 20.2 ⁎ | 146.4 ± 14.9 |
Percentage of predicted maximum heart rate (%) | 89.2 ± 9.8 | 85.9 ± 11.2 | 87.4 ± 9.7 |
Maximum systolic blood pressure (mm Hg) | 185.2 ± 25.8 | 182.8 ± 20.9 | 193.3 ± 28.5 |
Maximum diastolic blood pressure (mm Hg) | 88.1 ± 14.8 | 87.0 ± 17.2 | 90.0 ± 17.0 |
Rate pressure product § | 28,148.2 ± 5,103.0 | 26,671.9 ± 5,001.2 | 28,369.1 ± 5,385.5 |
Treadmill time (seconds) | 435.8 ± 167.0 | 442.7 ± 159.6 ‡ | 357.6 ± 146.2 † |
Rating of perceived exertion | 14.6 ± 2.7 | 14.7 ± 2.2 | 14.4 ± 3.9 |
Dyspnea on exertion | 1.7 ± 1.3 | 1.8 ± 1.3 | 2.0 ± 1.5 |
Chest pain | 0.3 ± 0.8 | 0.5 ± 1.0 | 0.41 ± 1.0 |
Peak respiratory exchange ratio | 1.10 ± 0.1 | 1.09 ± 0.2 | 1.12 ± 0.2 |
Peak oxygen consumption (ml O 2 × kg −1 × min −1 ) | 23.2 ± 7.0 | 22.32 ± 5.7 | 20.7 ± 7.0 |
Oxygen pulse flattening duration (seconds) | 70.73 ± 79.0 | 79.3 ± 97.4 | 61.34 ± 73.0 |
Oxygen pulse between peak and at rest | 9.7 ± 3.3 | 10.5 ± 3.3 | 9.7 ± 3.9 |
Minute ventilation/carbon dioxide production slope | 22.0 ± 3.0 | 22.3 ± 3.7 | 23.2 ± 4.5 |
Minute ventilation/carbon dioxide production slope 50-75 | 5.2 ± 6.3 | 5.2 ± 6.7 | 4.4 ± 6.9 |
Oxygen uptake efficiency slope | 2.5 ± 0.76 | 2.6 ± 0.66 | 2.6 ± 1.0 |
Oxygen uptake efficiency slope 50-75 | 0.27 ± 1.1 | 0.09 ± 1.1 | −0.4 ± 1.3 † |
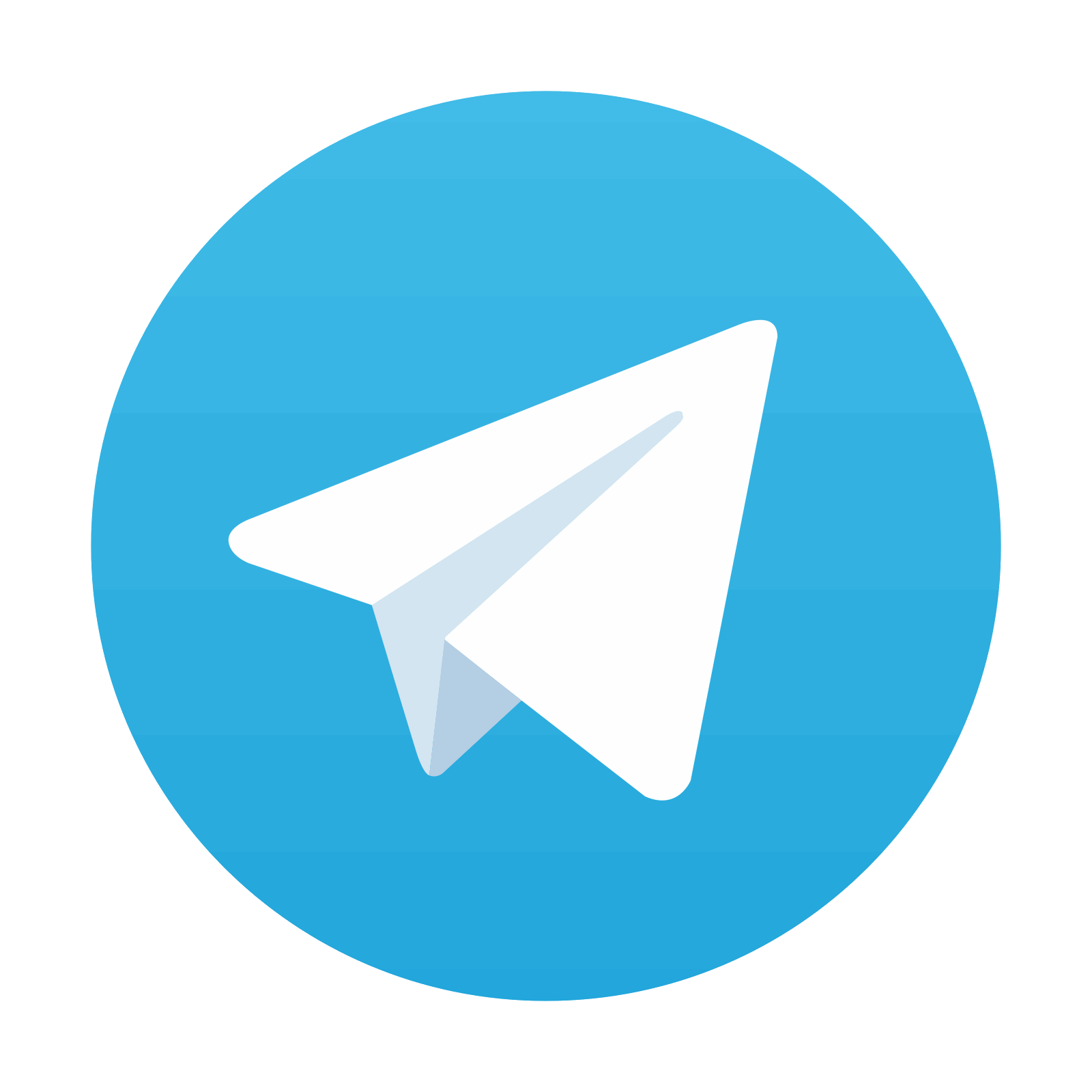
Stay updated, free articles. Join our Telegram channel

Full access? Get Clinical Tree
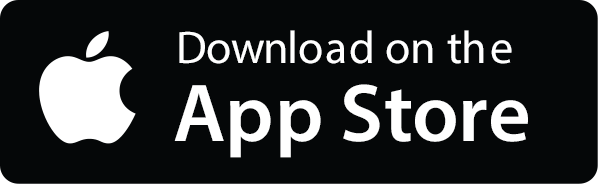
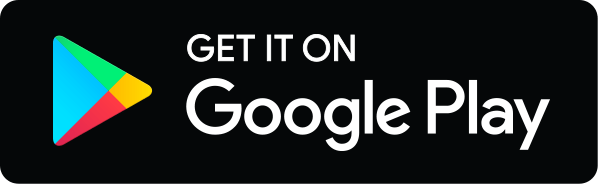
