Cardiovascular magnetic resonance (CMR) imaging can predict hemodynamically significant coarctation of the aorta (CoA) with a high degree of discrimination. However, the ability of CMR to predict important clinical outcomes in this patient population is unknown. Therefore, we sought to define the ability of CMR to predict the need for surgical or transcatheter intervention in patients with CoA. We retrospectively reviewed the data from 133 consecutive patients who had undergone CMR for the evaluation of known or suspected CoA. The characteristics of the CMR-derived variables predicting the need for surgical or transcatheter intervention for CoA within 1 year were determined through logistic regression analysis. Therapeutic aortic intervention was performed in 41 (31%) of the 133 patients during the study period. The indexed minimum aortic cross-sectional area was the strongest predictor of subsequent intervention (area under the receiver operating characteristic curve 0.975) followed by heart rate-corrected deceleration time in the descending aorta (area under the receiver operating characteristic curve 0.951), and the percentage of flow increase (area under the receiver operating characteristic curve 0.867). The combination of the indexed minimum aortic cross-sectional area and rate-corrected deceleration time in the descending aorta provided the best predictive model (area under the receiver operating characteristic curve 0.986). In conclusion, CMR findings can predict the need for subsequent intervention in CoA. These findings reinforce the “gate-keeper role” of CMR to cardiac catheterization by providing valuable diagnostic and powerful prognostic information and could guide additional treatment of patients with CoA with the final intent of reducing the number of diagnostic catheterizations in such patients.
Cardiovascular magnetic resonance (CMR) has become the preferred noninvasive diagnostic tool for the evaluation of patients with coarctation of the aorta (CoA). In contrast to nearly all other imaging modalities, CMR allows simultaneous evaluation of the morphology and function of the systemic ventricle and entire aorta, including visualization of the collateral vessels, measurement of the collateral flow, and assessment of the diastolic flow decay in the descending aorta, all of which can provide important information about the hemodynamic relevance of CoA. Recently, much effort has been devoted to exploring the interrelation of several CMR-derived variables and the hemodynamic severity of CoA as assessed against the reference standard of cardiac catheterization. The final intention is to develop an accurate and reliable noninvasive method to evaluate and classify patients with CoA, thereby reducing the number of unnecessary diagnostic catheterizations in such patients. With this intent, it becomes important to define the role of CMR in predicting the need for intervention in patients undergoing an initial evaluation for, or surveillance of, CoA. The indexed minimum aortic cross-sectional area, abnormal flow deceleration in the descending aorta, and amount of collateral flow as assessed with CMR have been shown to predict the CoA systolic pressure gradient. Therefore, we analyzed the value of these CMR-derived variables in predicting the need for intervention because of aortic arch obstruction.
Methods
The present study was approved by the institutional committee of human research and in accordance with the Health Insurance Portability and Accountability Act regulations. The present study was a retrospective, single-center, cohort study. The data from all consecutive patients (n = 161) who underwent CMR for the evaluation of suspected or known CoA at our institution from January 2003 through September 2009 were retrospectively reviewed. Missing contrast-enhanced 3-dimensional CMR angiography (n = 4), missing flow quantification in the descending aorta using phase-contrast CMR (n = 12), extra-anatomic repair with an ascending to descending aortic conduit (n = 5), the presence of ductus arteriosus (n = 1), and missing follow-up data after CMR (n = 6) were predefined exclusion criteria. Therefore, the final population consisted of 133 patients. Hemodynamic data were available only for patients undergoing subsequent catheterization (n = 39). In all 39 patients, the catheterization was performed after CMR (median interval between CMR and cardiac catheterization 82 days, range 0 to 350).
For statistical analysis, the outcome of intervention was dichotomized, with aortic arch intervention defined as any surgical or transcatheter intervention aimed at relieving aortic arch obstruction within 1 year of the initial CMR evaluation. Outcome status was determined by systematic review of the hospital and departmental databases or direct interview with the referring physician when necessary.
Scans were acquired with 2 different 1.5-Tesla scanners: Achieva (Philips Medical Systems, Best, The Netherlands), using a 5-channel cardiac coil (n = 92), or Signa CV/I (General Electric Medical Systems, Milwaukee, Wisconsin), using a 8-channel cardiac coil (n = 41). All patients underwent phase-contrast CMR in planes perpendicular to the proximal descending aorta just distal to the isthmus and the distal descending aorta at the level of the diaphragm, as previously described. On the Philips scanner, a 16-phase, free breath sequence was performed in 79 patients. The typical imaging parameters were as follows: echo time (TE) 3.2 to 6.6 ms, repetition time (TR) 5.4 to 10.0 ms, matrix 96 to 256 × 256, field of view (FOV) 240 to 320 mm, slice thickness 5 to 10 mm, and number of excitations 3. A 40-phase breath-hold sequence was used in 14 patients. The typical imaging parameters were TE 2.9 to 3.1 ms, TR 4.8 to 5.0 ms, matrix 128 × 256, FOV 320 mm, slice thickness 8 mm, and number of excitations 1. On the GE scanner, a 16-phase breath-hold sequence was performed in 2 patients (TE 5.7 to 5.9 ms, TR 5.4 to 10.0 ms, matrix 128 × 256, FOV 320 mm, slice thickness 8 mm, and number of excitations 1) and a 30-phase breath-hold sequence in 38 patients (TE 2.7 to 2.9 ms, TR 7.3 to 7.9 ms, matrix 128 × 256, FOV 320 mm, slice thickness 8 mm, and number of excitations 1). The velocity encoding value was individually adjusted according to the velocity of blood flow in the proximal descending aorta (200 to 600 cm/s). A fixed encoding velocity of 200 cm/s was used for the distal descending aorta. Three-dimensional CMR contrast-enhanced angiography in the parasagittal plane was performed in all patients after administration of gadopentetate dimeglumine IV (0.1 mmol/kg body weight, Magnevist, Bayer Schering, Berlin-Wedding, Germany) at a rate of 2.0 ml/s. The typical imaging parameters for the Philips scanner were TE 1.55 ms, TR 5.3 ms, matrix 173 × 400, FOV 340 × 400, and number of excitations = 1 and for the GE scanner were TE 2.7 ms, TR 5.5 ms, matrix 160 × 256, FOV 320 × 320, and number of excitations 0.5). The time delay between the start of the contrast injection and image acquisition was determined by bolus tracking. Two breath-hold sequential acquisitions were performed, each lasting from 20 to 30 seconds.
Flow analyses were performed on dedicated workstations (vPRO, Philips Medical Systems, and ReportCARD, General Electric Medical Systems, respectively). The following measurements were used: estimation of the amount of collateral flow and measurement of the diastolic flow deceleration time at the level of the distal descending aorta. The amount of collateral flow is expressed as the percentage of flow increase from the proximal descending aorta to the distal descending aorta: [percentage of flow increase = (amount of collateral flow/net forward flow proximal descending aorta) × 100], as previously described. The diastolic flow decay at the level of the distal descending aorta was characterized by measuring the deceleration time, which was adjusted to the heart rate using Bazett’s formula: 1/(RR interval) 0.5 , as previously described ( Figure 1 ).
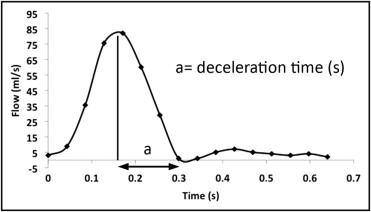
From the 3-dimensional contrast angiographic findings, the minimum cross-sectional area at the site of CoA was measured by planimetry and adjusted to the body surface area, as previously described by Nielsen et al. All measurements were performed by a single observer (S.M.), with 2 years of experience in CMR, who was unaware of the outcomes data.
Statistical analysis was performed using a commercially available statistical package (SPSS, version 16.0., Chicago, Illinois). Summary statistics for continuous variables are presented as the median (range). Categorical variables are presented as numbers and percentages. The relation between the CMR predictor variables and the outcome of aortic arch intervention was explored through univariate and multiple variable logistic regression analyses. Predictor variables were specified a priori and included the indexed minimum aortic cross-sectional area, rate-corrected deceleration time in the descending aorta, and percentage of flow increase, because these variables have been previously shown to predict the CoA systolic pressure gradient.
Results
The characteristics of the study cohort are listed in Table 1 . Notably, <1/3 of the patients had native or suspected native CoA, and the others had already undergone surgical or transcatheter repair. Of the 133 patients, 40 underwent aortic intervention during the study period. Of these 40 patients, 11 underwent surgical repair and 29 transcatheter balloon angioplasty or endovascular stenting. Most of these 40 patients had native CoA (n = 23, 58%); 15 patients (37%) had undergone previous surgery with evidence of recurrent arch obstruction, and 2 (5%) had previously been treated with balloon angioplasty without stenting. One additional patient was found to require intervention after CMR and cardiac catheterization; however, intervention was rejected because of the anatomic complexity of the lesion and the global co-morbidity profile. For the present analysis, this patient was allocated to the group of patients in need of intervention. Therefore, 41 (31%) of 133 patients either underwent intervention for aortic arch obstruction or met the criteria but did not undergo intervention.
Variable | Value |
---|---|
Age (years) | |
Median | 21.9 |
Range | 0.63–58.9 |
Men | 86 (65%) |
Body surface area (m 2 ) | |
Median | 1.78 |
Range | 0.50–2.50 |
Heart rate (beats/min) | |
Median | 71 |
Range | 43–111 |
Native coarctation ⁎ | 41 (31%) |
Type of previous repair ⁎ | |
Surgical repair | 76 (58%) |
Stent angioplasty | 11 (8%) |
Balloon angioplasty | 3 (2%) |
Of the 133 patients, 39 underwent cardiac catheterization after diagnostic CMR, of whom 29 underwent transcatheter intervention, 1 underwent surgical repair, and 9 were followed up conservatively. The peak systolic pressure gradient was significantly greater in patients undergoing invasive treatment compared to that in the others (31 ± 15 mm Hg vs 7 ± 4 mm Hg; p <0.01). Of the 11 patients who underwent surgical intervention, only 1 underwent catheterization before the intervention. The patients undergoing catheterization after CMR were more likely to have a native CoA (47% vs 24%) and to meet the criteria for significant CoA by CMR, including a smaller indexed minimum aortic cross-sectional area (0.70 ± 0.33 cm 2 /m 2 vs 1.28 ± 0.49 cm 2 /m 2 ), a longer rate-corrected deceleration time (0.37 ± 0.14 seconds 0.5 vs 0.27 ± 0.11 seconds 0.5 ), and more collateral flow (median −2%, interquartile range −10% to 4%, vs median 16%, interquartile range 0% to 33%; p <0.05 for all). No significant differences were found concerning age, gender, and body surface area.
Notably, although this was a retrospective study and the decision concerning the treatment strategy was taken by the treating physician and without study-related prespecified criteria, we established a posteriori the indication for intervention using the most recent guidelines for all patients (n = 30) with available hemodynamic data. Twenty-five patients had a peak pressure gradient of ≥20 mm Hg. Of the remaining 5 patients with a systolic pressure gradient <20 mm Hg, 3 had relevant collaterals, as assessed by CMR (percentage of flow increase 21%, 66%, and 33%, respectively). In the last 2 patients, intervention was motivated by either poorly controlled hypertension or evidence of impaired left ventricular systolic function.
The minimum indexed aortic cross-sectional area, rate-corrected deceleration time, and percentage of collateral flow all predicted the need for intervention ( Table 2 and Figure 2 ). The predictive value of these CMR-derived variables was similar in the subset of 39 patients, who had undergone cardiac catheterization after the CMR examination ( Table 3 and Figure 3 ). A logistic model combining the minimum aortic cross-sectional area and rate-corrected deceleration time resulted in the best predictive characteristics, as given by log [p/1 − p] = −3.658 + 0.394 × (rate-corrected deceleration time) − 0.875 × (minimum indexed aortic cross-sectional area), where p is the probability of aortic arch intervention. Using a cutoff of probability of >0.5, this model correctly classified 96% of the patients, with a sensitivity of 88%, a specificity of 100%, and an area under the receiver operating characteristic curve of 0.986 (p = 0.22 for comparison with the area under the receiver operating characteristic curve of the indexed minimum aortic cross-sectional area). Applying this predictive role to the subset of patients (n = 39) undergoing cardiac catheterization after the CMR study, 34 (87%) would have been classified correctly in respect to the final treatment strategy, which was decided after implementation of the diagnostic information provided by cardiac catheterization.
