Patients with type 2 diabetes mellitus (T2DM) might have subclinical myocardial dysfunction identified at rest or unmasked during exercise. We examined the correlates of the myocardial exercise response in patients with T2DM. Myocardial dysfunction was sought during at rest and exercise echocardiography in 167 healthy patients with T2DM (97 men, 55 ± 10 years). Myocardial ischemia was excluded using stress echocardiography. Standard echocardiography and color tissue Doppler imaging measures (early diastolic tissue velocity [Em], strain, and strain rate) were acquired at baseline and peak stress. The calibrated integrated backscatter was calculated from the at rest parasternal long-axis view. The longitudinal diastolic functional reserve index after exercise was defined as ΔEm [1 − (1/Em base )]. The clinical, anthropometric, and metabolic data were collected at rest and stress. Subclinical myocardial dysfunction at baseline (n = 24) was independently associated with weight (odds ratio [OR] 1.02, p = 0.04) and hemoglobin A1c (OR 1.36, p = 0.03). This group displayed an impaired exercise response that was independently associated with a reduced exercise capacity (OR 0.84, p = 0.034) and longitudinal diastolic functional reserve index (OR 0.69, p = 0.001). Inducible myocardial dysfunction (stress Em <−9.9 cm/s) was identified after exercise in 70 of the remaining 143 subjects. This finding was associated with calibrated integrated backscatter (OR 1.08, p = 0.04) and lower peak heart rate (OR 0.97, p = 0.002) but not metabolic control. The intensity of the metabolic derangement in patients with T2DM was associated with subclinical at rest myocardial dysfunction, but not with the myocardial exercise response. In conclusion, the association of an abnormal stress response with nonmetabolic factors, including backscatter and blunted peak heart rate, suggests potential roles for myocardial fibrosis and cardiac autonomic neuropathy in patients with nonischemic diabetic heart disease.
In addition to hastening atherosclerosis, type 2 diabetes mellitus (T2DM) and the metabolic syndrome have been linked to myocardial disease in the absence of ischemic heart disease and hypertension. This is likely multifactorial, secondary to the accumulation of advanced glycated end products, myocardial fibrosis, microvascular disease, and autonomic neuropathy. Diabetic heart disease is initially asymptomatic; however, nonspecific symptoms of fatigue, dyspnea, or reduced exercise tolerance will gradually develop. Early detection might facilitate measures to prevent disease progression. Tissue velocity and deformation imaging can detect myocardial dysfunction when the conventional 2-dimensional echocardiographic parameters are normal. In early diabetic heart disease, myocardial function might be preserved at rest, with exercise unmasking a blunting of contraction and relaxation, indicative of an abnormal functional reserve. Longitudinal function is typically reduced initially, reflective of the early involvement of the subendocardial fibers. Impairment in the at rest and peak exercise systolic tissue velocity has been associated with common metabolic risk factors in asymptomatic patients. We sought to identify whether early diastolic tissue velocity (Em), deformation imaging, and tissue characterization could identify diabetic heart disease not apparent at rest and examined the correlates of myocardial dysfunction with exercise.
Methods
A total of 167 apparently healthy subjects with T2DM (97 men, 55 ± 10 years) and no macro- or microvascular complications of T2DM or history of hypertension or valvular, congenital, or ischemic heart disease were recruited from the hospital clinics of the Princess Alexandra Hospital and its local community. Sinus rhythm and normal renal function were required for inclusion. Antihypertensive medications were withheld for ≥12 hours before testing. The human research ethics committees of Princess Alexandra Hospital and the University of Queensland (Brisbane, Australia) approved the present study.
Clinical data were collected regarding subject age, gender, weight, height, waist and hip circumference, smoking status, and duration of T2DM. Venesection was performed before exercise after the subjects had fasted for ≥8 hours. The tested parameters included fasting glucose, hemoglobin A1c (HbA1c), creatinine, and lipid profile. Microalbuminuria, a marker of microvascular disease, was quantified using a random urinary albumin/creatinine ratio and defined as ≥2.5 g/mol for men and ≥3.5 g/mol for women. The heart rate and blood pressure were measured at baseline, throughout exercise, and during recovery. The peak exercise capacity was estimated in METs according to the duration of exercise using the equation: METs = [speed × (0.1 + [grade × 1.8]) + 3.5]/3.5.
Standard commercially available cardiac ultrasound machines (Vivid 7, General Electric Medical Systems, Milwaukee, WI) were used to perform M-mode and 2-dimensional echocardiography to assess the chamber wall thickness, valvular morphology, and chamber volumes. Baseline parasternal and apical images were acquired in gray scale and color tissue Doppler imaging (TDI) formats. These were used as the at rest reference images for comparison of the stress echocardiographic images in the same views and to enable off-line TDI parameter measurement. Stress echocardiography was performed to exclude inducible segmental myocardial dysfunction indicative of underlying hemodynamically significant epicardial coronary artery disease. The subjects underwent treadmill exercise using the Bruce protocol, and the peak images in the parasternal and apical views using the same formats were acquired. The images were digitally saved for off-line analysis.
The left ventricular end-systolic and end-diastolic volumes were quantified at rest and peak to calculate the ejection fraction using the modified Simpson biplane method. Conventional apical views (4-chamber, 2-chamber, and long-axis) in color TDI format at rest and peak were used to obtain the tissue velocity, strain, and strain rate curves from the 6 basal segments using standard commercial software (Echopac, GE Vingmed). The peak systolic tissue velocity (Sm) and peak Em were calculated from the tissue velocity curves by placing a sample volume at the annulus of the mitral valve. An average value from 3 consecutive tissue velocity curves was established. On some stress images, the transmitral and TDI diastolic velocity curve peaks were fused. In these instances, we waited until the heart rate had decreased and they had separated.
Abnormal diastolic function at rest was defined as an at rest septal Em <2 SD of normal for age. Normal age-adjusted values for peak exercise stress Em have not yet been defined. Hence, abnormal exercise diastolic function was identified when the stress septal Em was <−9.9 cm/s, the mean value in a nondiabetic control population. Longitudinal left ventricular diastolic functional reserve index, a measure of augmentation in diastolic relaxation during exercise, was calculated using the previously defined equation: longitudinal left ventricular diastolic function reserve index = ΔEm [1 − (1/Em base )], where ΔEm was the change in Em from baseline to peak exercise and Em base was the early diastolic tissue velocity at baseline measured at the septal mitral annulus. This normalization equation was used to express the ΔEm with respect to the baseline Em at rest. According to previous studies, the preserved contractile reserve was defined as augmentation of the ejection fraction ≥4% and/or an increase in the stress Sm from the baseline at rest Sm (ΔSm) ≥2.4 cm/s. The strain and strain rate curves were derived from the apical views in color TDI format by placing sample volumes in the mid-myocardial layer of the 6 basal segments and tracking the position of the sample volume throughout the cardiac cycle. An average peak value from 3 consecutive curves was used to calculate the strain and strain rate. The angle of incidence between the transducer and the wall of interest was maintained at <20° to not underestimate the degree of deformation. The calibrated integrated backscatter (cIB) was calculated by measuring the tissue intensity of the pericardium, posterior wall, and anterior septum in the parasternal long-axis view. Automated tissue tracking enabled maintenance of the sample volume of interest within the designated myocardial segment throughout the cardiac cycle. An integrated backscatter curve was derived using commercial software (Echopac, GE Vingmed). The mean cIB was calculated by subtracting the pericardial integrated backscatter intensity at end-diastole from the integrated backscatter intensity of the posterior wall and the anterior septum, which were then averaged.
Interobserver and intraobserver variability were assessed by repeat measurement of the tissue velocity, strain, strain rate, and cIB on the at rest and peak stress images from 10 randomly selected subjects. The original observer (C.J.) was unaware of the previous measurements performed ≥4 weeks earlier to evaluate the reproducibility. A second observer (R.L.) was unaware of the first observer’s results to assess the variability. In addition to the absolute difference between the measurements, the intraclass correlation coefficient was used to determine overall variability. This analysis of reliability was performed using a 2-way random effects model to assess absolute agreement. The results are expressed as the mean ± SD. The analysis between defined categorical groups (normal vs abnormal) was performed using Student’s independent t test for continuous variables and the chi-square test for categorical variables. Independent associations between the echocardiographic and metabolic parameters were sought with a stepwise selection method to build logistic or linear regression models of the independent variables. Candidate variables for the models were selected from the unadjusted correlates listed in Table 1 that were significant at p <0.10 and not co-linear. Statistical analysis was performed using standard software (Statistical Package for Social Sciences, version 16, SPSS, Chicago, Illinois). p Values = 0.05 were statistically significant.
Variable | At Rest Em | Stress Em | ||||
---|---|---|---|---|---|---|
Normal (n = 143) | Abnormal (n = 24) | p Value | Normal (n = 73) | Abnormal (n = 70) | p Value | |
Age (years) | 55 ± 10 | 53 ± 11 | 0.389 | 53 ± 9 | 58 ± 10 | 0.007 |
Type 2 diabetes mellitus duration (years) | 5.3 ± 5.5 | 9.7 ± 8.9 | 0.024 | 5.1 ± 5.4 | 6.1 ± 6.3 | 0.310 |
Weight (kg) | 89.5 ± 17.0 | 98.5 ± 28.5 | 0.035 | 89.7 ± 17.4 | 90.2 ± 16.9 | 0.848 |
Body mass index (kg/m 2 ) | 31.3 ± 5.4 | 33.7 ± 7.2 | 0.054 | 31.5 ± 5.6 | 31.2 ± 5.3 | 0.768 |
Fasting glucose (mmol/L) | 8.1 ± 2.8 | 9.6 ± 3.8 | 0.030 | 8.2 ± 3.0 | 8.2 ± 2.8 | 0.873 |
Hemoglobin A1c (%) | 7.4 ± 1.4 | 8.1 ± 1.6 | 0.024 | 7.5 ± 1.4 | 7.4 ± 1.4 | 0.458 |
Total cholesterol | 0.105 | 0.019 | ||||
mmol/L | 4.8 ± 0.9 | 4.5 ± 0.8 | 5.0 ± 0.8 | 4.7 ± 1.0 | ||
mg/dl | 186 ± 35 | 174 ± 31 | 193 ± 31 | 182 ± 39 | ||
Low-density lipoprotein cholesterol | 0.035 | 0.084 | ||||
mmol/L | 2.7 ± 0.8 | 2.3 ± 0.8 | 2.8 ± 0.8 | 2.6 ± 0.9 | ||
mg/dl | 104 ± 31 | 89 ± 31 | 108 ± 31 | 101 ± 35 | ||
Creatinine (mmol/L) | 79 ± 18 | 77 ± 24 | 0.624 | 78 ± 17 | 80 ± 19 | 0.459 |
Microalbuminuria (%) [albumin/creatinine (g/mol)] | 16 (11%); 0.7 ⁎ ; IQR 0.9 | 6 (25%); 1.1 ⁎ ; IQR 2.2 | 0.064 | 7 (10%); 0.8 ⁎ ; IQR 0.7 | 8 (11%); 0.7 ⁎ ; IQR 0.9 | 0.720 |
Statin therapy | 55 (38%) | 16 (67%) | 0.010 | 24 (33%) | 32 (46%) | 0.116 |
Angiotensin-converting enzyme inhibitors and/or angiotensin-receptor blockers | 58 (41%) | 18 (75%) | 0.005 | 28 (39%) | 30 (43%) | 0.474 |
β Blockers | 9 (6%) | 1 (4%) | 0.684 | 1 (1%) | 8 (11%) | 0.013 |
At rest heart rate (beats/min) | 85 ± 13 | 88 ± 16 | 0.330 | 88 ± 12 | 81 ± 13 | 0.001 |
At rest systolic blood pressure (mm Hg) | 133 ± 17 | 138 ± 17 | 0.259 | 132 ± 16 | 134 ± 17 | 0.572 |
At rest diastolic blood pressure (mm Hg) | 81 ± 10 | 86 ± 8 | 0.033 | 81 ± 10 | 81 ± 9 | 0.949 |
Peak heart rate (beats/min) | 163 ± 20 | 159 ± 19 | 0.363 | 170 ± 17 | 155 ± 20 | <0.001 |
Exercise capacity (METs) | 9.2 ± 3.2 | 7.5 ± 2.5 | 0.013 | 9.4 ± 3.3 | 9.0 ± 3.0 | 0.439 |
At rest end-systolic volume (ml) | 25 ± 10 | 29 ± 12 | 0.137 | 26 ± 10 | 25 ± 10 | 0.314 |
At rest end-diastolic volume (ml) | 73 ± 19 | 78 ± 26 | 0.210 | 72 ± 20 | 73 ± 19 | 0.753 |
At rest ejection fraction (%) | 65 ± 7 | 64 ± 8 | 0.241 | 64 ± 6 | 67 ± 7 | 0.017 |
Stress end-systolic volume (ml) | 18 ± 7 | 10 ± 8 | 0.224 | 18 ± 7 | 18 ± 7 | 0.906 |
Stress end-diastolic volume (ml) | 69 ± 19 | 71 ± 23 | 0.590 | 69 ± 18 | 70 ± 21 | 0.718 |
Stress ejection fraction (%) | 74 ± 5 | 73 ± 6 | 0.553 | 74 ± 5 | 74 ± 6 | 0.852 |
Change in ejection fraction (%) | 9 ± 6 | 9 ± 7 | 0.825 | 10 ± 6 | 7 ± 7 | 0.014 |
At rest early diastolic tissue velocity (cm/s) | −5.7 ± 1.5 | −3.1 ± 1.0 | <0.001 | −5.8 ± 1.4 | −5.5 ± 1.6 | 0.365 |
At rest systolic tissue velocity (cm/s) | 4.9 ± 1.1 | 2.9 ± 1.4 | <0.001 | 4.9 ± 1.1 | 4.8 ± 1.2 | 0.614 |
Stress early diastolic tissue velocity (cm/s) | −9.9 ± 2.5 | −6.3 ± 4.5 | <0.001 | −11.5 ± 1.9 | −8.1 ± 1.7 | <0.001 |
Stress systolic tissue velocity (cm/s) | 8.0 ± 2.0 | 5.4 ± 2.6 | <0.001 | 8.3 ± 1.9 | 7.5 ± 2.2 | 0.012 |
Change in systolic tissue velocity (cm/s) | 3.1 ± 1.9 | 2.5 ± 1.6 | 0.184 | 3.5 ± 1.8 | 2.6 ± 1.9 | 0.006 |
Left ventricular longitudinal functional reserve index | 3.5 ± 2.5 | 1.3 ± 2.2 | <0.001 | 5.3 ± 1.7 | 1.6 ± 1.7 | <0.001 |
At rest strain (%) | −20.8 ± 3.1 | −19.6 ± 3.7 | 0.09 | −20.6 ± 3.0 | −20.8 ± 3.2 | 0.605 |
Stress strain (%) | −21.2 ± 3.5 | −21.2 ± 3.6 | 0.978 | −20.6 ± 3.7 | −22.0 ± 3.2 | 0.020 |
At rest strain rate (s −1 ) | −1.3 ± 0.3 | −1.4 ± 0.3 | 0.160 | −1.3 ± 0.3 | −1.3 ± 0.3 | 0.728 |
Stress strain rate (s −1 ) | −1.9 ± 0.5 | −2.0 ± 0.5 | 0.181 | −1.9 ± 0.5 | −1.9 ± 0.4 | 0.692 |
Calibrated integrated backscatter (dB) | −17.1 ± 5.4 | −16.3 ± 5.7 | 0.539 | −18.0 ± 4.9 | −15.9 ± 5.8 | 0.019 |
Height-indexed left ventricular mass (g/m 2.7 ) | 51.0 ± 16.7 | 45.2 ± 16.6 | 0.125 | 52.4 ± 18.1 | 49.6 ± 15.0 | 0.360 |
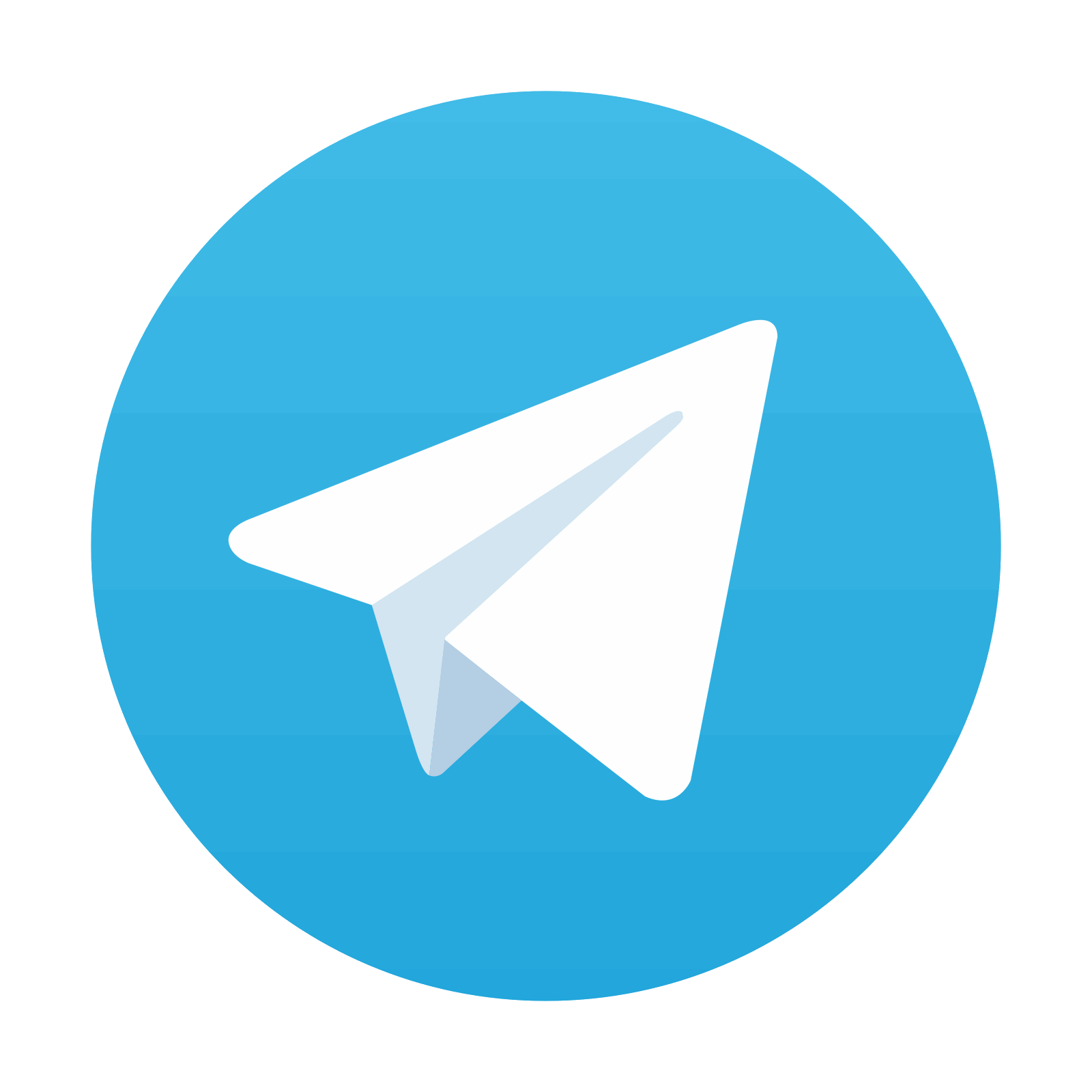
Stay updated, free articles. Join our Telegram channel

Full access? Get Clinical Tree
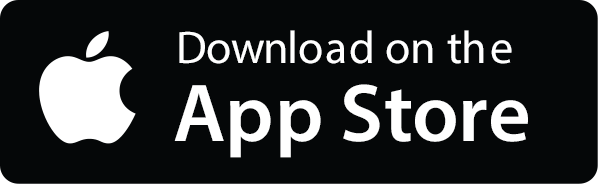
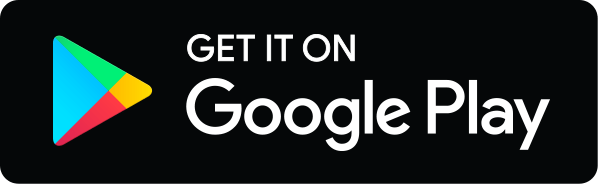
