div epub:type=”chapter” role=”doc-chapter”>
25. The Use of Preoperative Imaging for Planning Endovascular and Hybrid Procedures
Endovascular and hybrid procedures have become the mainstay minimally invasive treatment options for complex vascular disorders. Advanced imaging is crucial for planning and followup of such procedures. The most common preoperative imaging modalities for this planning are ultrasound (US), computed tomography angiography (CTA), and magnetic resonance angiography (MRA). This chapter focuses on the relative practical value and benefits of these modalities in common vascular surgical indications. The physical principles on which these modalities are based and other technical details are well described elsewhere [1] and are not discussed in this text.
Ultrasound
Ultrasonography enables live imaging for both diagnosis and real-time guidance of interventions in various disease processes. Ultrasonography’s advantage in vascular diseases relates to its ability to assess vascular flow patterns using spectral and color Doppler imaging, in addition to gray-scale cross-sectional imaging and real-time guidance, which is of crucial importance in vascular interventions. Though simple in principle, its potential has greatly expanded with advancements in technology.
Surface Ultrasonography
The most commonly used ultrasound modality is in the form of a hand-held surface probe. The probe comes in a variety of shapes, the most common of which are curvilinear, phased array, and linear probes. Low-frequency probes (1–5 MHz) are better suited for evaluating deeper structures such as intraabdominal organs. High-frequency probes (5–10 MHz) are better adapted for examining superficial vascular structures. Several imaging modes—including two-dimensional (2D), three-dimensional (3D), spectral Doppler, color flow, and power mode—provide the user with different information. The 2D mode allows for soft-tissue characterization, including anatomic assessment of aneurysmal and atherosclerotic disease. Ultrasound imaging can provide crucial information pertaining to vascular anatomy, relationships, and orientation of the territory being imaged. The spectral Doppler mode allows for the quantitative assessment of velocities, allowing for detection of significant stenosis. The color flow mode detects the direction of blood flow.
Because of its versatility, noninvasiveness, portability, and relatively low cost, ultrasound has become a key imaging modality in vascular surgery. It can be used to diagnose vascular disease, such as stenosis, occlusions, and dissection. It also can help to characterize the lesion and determine whether the morphology is better suited for endovascular or open repair. Intraoperatively, ultrasound is also beneficial in ensuring safe vascular access into vessels can be instrumental when obtaining access into a pulseless vessel, in scarred groins, or in heavily diseased or calcified access vessels. It can help reduce the risk of complications in difficult and variant anatomy by locating the level of the femoral bifurcation, to ensure that the puncture is into a non-diseased segment of the common femoral artery. Its use also can enhance the safety of vascular closure devices, especially in patients with hostile or distorted anatomy. Post-procedure, surface ultrasound is routinely used on followup to detect any recurrent disease. As a result, the use of surface ultrasound allows versatility preoperatively, intraoperatively, and postoperatively. Ultrasound can also be helpful in deciding on making a targeted incision over a diseased vessel, sometimes referred to as precision surgery. For example, by marking the location of the carotid bifurcation, it becomes possible to make a small incision instead of a large incision from ear to sternum. A similarly small groin incision can be used to tackle an occluded common femoral artery.
Using surface ultrasound through a hand-held probe has numerous advantages. It is an accessible, quick, and portable modality with many modes and settings that equip skilled users with real-time imaging and knowledge. It is also an inexpensive and benign method of imaging, without the radiation exposure of CT scans. Disadvantages include limitations from the anatomy itself, such as when there are significant differences in acoustic impedance in tissues. Deeper structures, especially in obese patients, also are not as easily penetrated by ultrasound probes with a restricted range of frequencies. Most importantly, its use is operator-dependent. A high level of skill and experience are needed to obtain good-quality diagnostic images, but these are certainly obtainable with training and practice.
Several studies have shown the role of contrast-enhanced ultrasound in surveillance following endovascular aortic repair (EVAR). Compared with CT angiography, it showed very good diagnostic performance, absence of renal impairment, absence of radiation exposure, and low costs [2, 3].
Ultrasound is a versatile tool in vascular surgery, which can be employed both extravascularly and intravascularly to assist with diagnosis, treatment, and surveillance of vascular disease. With the constant advancements in technology, the use of ultrasound will continue to expand, and vascular surgeons will need to keep up with technology in order to take advantage of its full potential in treating patients.
Intravascular Ultrasound
Intravascular ultrasound (IVUS) has gained popularity, especially for complex aortic dissections [4]. It enables continuous real-time characterization of the vessel from within the vessel itself. There are two types of catheters: a multi-element phase-array catheter and a catheter with a mechanically rotating element. The multi-element phase-array catheter utilizes a miniature integrated circuit at its tip, whereas the mechanically rotating type employs a rotating transducer at the tip. During intraoperative use, the catheter allows evaluation of vessel wall integrity, angulation of vessels, branching points, ostial disease, thrombus, or dissection.
IVUS is employed most frequently as an adjunct during endovascular cases. When treating atherosclerotic disease, IVUS provides accurate measurements of luminal dimensions and lengths of landing zones. With an accurate assessment of anatomy, IVUS enables proper stent selection, sizing, and placement to treat atherosclerotic vascular disease. It also can be used to evaluate stent-to-vessel apposition. When optimized, all these factors promote long-term patency, which is supported by the literature. IVUS is now considered a mainstay diagnostic tool in managing iliac venous compression syndrome and guiding associated interventions. It also has become a key imaging modality during endovascular management of aortic dissections. It can provide precise information regarding the extent of the dissection and can help differentiate between the true and false lumens. This information in turn dictates whether the anatomy is conducive to endovascular repair, maps out how to do so, aids in the selection of disease-free fixation points, and ensures that other branching vessels have not been compromised. The IVUS-tipped catheter is fed over the guidewire and advanced past the area of evaluation. Then it is gradually pulled back through the area of interest, usually a stenosis or dissection flap. Images taken with the transducer are delivered to an external computer for real-time display of the endovascular and wall morphology.
The main advantages of IVUS include real-time visualization of vessel luminal anatomy and lesion-specific features not evaluable on fluoroscopic imaging or preoperative cross-sectional imaging. IVUS is also a useful intervention-guidance modality that can be used to guide many endovascular interventions. It also serves as an accurate modality for vessel diameter sizing and for post-intervention assessment, including assessment of dissection after percutaneous transluminal angioplasty (PTA) or assessment of stent expansion and stent-wall apposition, all of which have important implications for procedural outcome and long-term patency [5, 6]. The ability to perform intraluminal measurements is critical in choosing the right equipment for treating the disease. Studies have reported accuracy of luminal dimensions and wall thickness to within 0.05 mm [7, 8]. With direct visualization using IVUS, the amount of contrast and time of fluoroscopy can be decreased significantly. The use of IVUS and a standalone guidance modality during thoracic EVAR (TEVAR) and EVAR enables these procedures to be performed safely and eliminates or minimizes the need for iodinated contrast agents [9, 10]. One study demonstrated that that actual vessel size and lumen diameter were underestimated 62% of the time by arteriography, and that 40% of stents placed in the iliac arterial system were under-deployed, which may be related to treatment failure [11, 12]. Disadvantages of IVUS include its high expense and lengthening of procedure time. As with other ultrasound devices, it is operator-dependent and should be used cautiously even in the hands of a skilled interventionalist, to avoid potential embolization of plaque, dissection, or rupture of the vessel. Moreover, the accuracy of cross-sectional measurements may sometimes be limited, with underestimation in the elliptical lumens present in severely diseased vessels, in thoracic aneurysms, or in tortuous vessels [13–16].
Computed Tomography Angiography

Commonly used volumetric reformatting methods in CT or MR angiography: maximum intensity projections (MIP) and volume rendering

MIP versus multiplanar reconstruction (MPR) in visualization of a focal, non-occlusive, web-like stenosis in the distal left limb of an aortobifemoral bypass. Volume averaging in MIP results in blurring of focal details; the lesion becomes obscured
Neurovascular Circulation
Prediction of Carotid Stenosis Using CT Angiography
Severity | Lumen Diameter | Stenosis | Sensitivity | Specificity |
---|---|---|---|---|
Mild | >2.2 mm | <50% | 95.2% | 93.2% |
Moderate | 2.2 – >1.3 mm | 50–69% | 75.0% | 93.8% |
Severe | ≤1.3 mm | ≥70% | 88.2% | 92.4% |
Peripheral Vasculature
Compared with angiography, CTA has high sensitivity, identifying 86.3% of all infrainguinal lesions. When separating the lesions into those above or below the popliteal trifurcation, the accuracy of CTA was 87.3% and 80.2% [23]. CTA can be used for preoperative planning of endovascular interventions by assessing appropriate access sites and target vessel intervention; in open interventions, it can help to decide appropriate inflow and outflow for peripheral bypass. CTA is often used in conjunction with ultrasound for peripheral arterial disease; it is comparable to angiography but is less invasive.
Aorta and Viscera
Ultrasound surveillance is generally recommended for monitoring of aortic disease, but if there is concern that the size of an abdominal aortic aneurysm (AAA) is approaching 5.0 cm or greater, CTA may be used for further evaluation to determine whether surgical intervention is appropriate [24]. CTA also can be appropriate for monitoring if the patient is obese and ultrasound imaging cannot be obtained or is unreliable.
If open repair is planned, imaging is important to assess the aneurysm to determine the operative approach, extent, and clamp location. For endovascular repair, CTA imaging is used in both the preoperative and postoperative settings. In the preoperative setting, it is used for operative planning to determine suitability for endovascular repair and the type of endovascular repair (infrarenal EVAR or fenestrate/branch EVAR), depending on whether the aneurysm is infrarenal, juxtarenal/pararenal, or suprarenal. CTA yields important variables such as the length of the aneurysm, the diameter of the proximal and distal seal and fixation sites, and qualities of landing zones, such as calcification, thrombus, and non-parallel wall configuration. These measurements are used to select the size of the endograft. CTA also can contribute to preoperative planning by evaluating hypogastric patency, evaluating for synchronous aneurysmal disease, and evaluating access sites. In the postoperative setting, CTA is utilized to monitor for stent failure and to help with preoperative planning should the patient require an additional intervention, but research and evidence indicate that ultrasound plays an important and appropriate role in postoperative monitoring, particularly once the aneurysm sac has stabilized, as CTA is associated with radiation and risk from contrast.
Magnetic Resonance Angiography
Magnetic resonance angiography (MRA) has usually required the administration of diamagnetic or ferromagnetic contrast agents to outline the vascular lumen, much like the iodinated contrast agents used in CTA [18, 25–27]. MRA also can be obtained without intravascular contrast, however, by utilizing flow-based techniques. Recent advances in MR imaging has allowed these flow-based enhancement techniques to offer alternative vascular imaging options in patient who cannot tolerate iodinated contrast agents or magnetic contrast agents [28–30]. Drawbacks include the susceptibility of MRI to motion artifact and the inability to use it in patients who have pacemakers or automatic defibrillators. Metal-related artifacts also limit its value in proximity to metallic implants.
The imaging processing of MRA is similar to that of CTA and includes both MIP and volume rendering [1, 18]. With MIP, the data can be manipulated to analyze maximum intensities along different rays, similar to volume rendering. The problem with this technique is that there are not as many rays, so there is potential for missing small differences like stenoses or dissections [31].
Neurovascular Circulation
Opinions are mixed as to the appropriateness of MRA as a confirmatory test. Studies have identified varying sensitivity and specificity of MRA for carotid disease; Layton et al. demonstrated that the sensitivity of MRA for identifying carotid stenosis was highly dependent upon how many normal carotid arteries were included in the study [32]. Another concern regarding MRA (although controversial) is that it may overestimate stenosis in carotid disease.
Peripheral Vasculature
MRA is a well-recognized modality for preoperative planning in patients with peripheral vascular disease [33]. MRA is also accurate for postoperative bypass assessment after bypass surgery, though it suffers from moderately lower specificity than CTA; when assessing stenosis within the native artery, its sensitivity was 96.0% and its specificity was 87.6%. MRA is also less helpful for preoperative assessment of vascular calcification, but this drawback can be advantageous in allowing better assessment of the flow lumen (compared with CTA) in the presence of heavy calcifications. MRA is appropriate for postoperative monitoring or as an adjunct to preoperative evaluation for patients requiring an additional intervention to improve the secondary patency rate of a bypass.
Aorta and Visceral Arteries
Currently there are no well-established guidelines regarding MRA use to evaluate aortic disease in the surveillance period or the preoperative or postoperative setting, though some studies do support MRA. Gadolinium-enhanced MRA allows imaging of the abdominal aorta down to the groins using a breath hold. MRA imaging has been shown to be inferior to CTA for evaluating the renal arteries, with a tendency to exaggerated stenosis severity and susceptibility to motion artifact [34]. MRA is particularly useful, however, in patients with contrast allergies who are unable to undergo CTA or angiography; it has been shown to be superior to CO2 angiography.
Conclusions: Preoperative Imaging Modalities
CTA is an appropriate modality for imaging of the vascular system and is crucial to preoperative and postoperative evaluation of carotid, aortic, and peripheral vascular disease [22, 23, 35–38]. However, CTA is inappropriate for the many patients who have concurrent renal disease resulting in a significant risk of kidney injury.
MRA can be an alternative option if CTA imaging is not appropriate. Though MRA is not widely used, there is evidence of its efficacy and reliability for the preoperative and postoperative evaluation of carotid, aortic, and peripheral vascular disease [32–34, 39–44]. Some negatives to using MRA include its cost, lack of accessibility, lack of previous operator use, and potential overestimation of stenotic lesions, as previously described.
The gold standard for any vascular imaging remains digital subtraction angiography, but this modality is invasive, depends upon contrast, and must be performed by an interventionalist. Thus, it is worth considering the other good options that are now available.
Advanced Adjuncts
Three-Dimensional Imaging
Three-dimensional (3D) imaging uses advanced image analysis to enable accurate multidimensional assessment of vascular anatomy and dimensions. It also can reveal key relationships to other relevant anatomic landmarks. The analysis of 3D vascular imaging has become key in presurgical analysis to help plan complex endovascular interventions. It is particularly indispensable for the planning of aortic aneurysm repair, especially for procedures requiring custom stent-graft designs or in fenestrated/branched endovascular aortic repair (F/BEVAR).

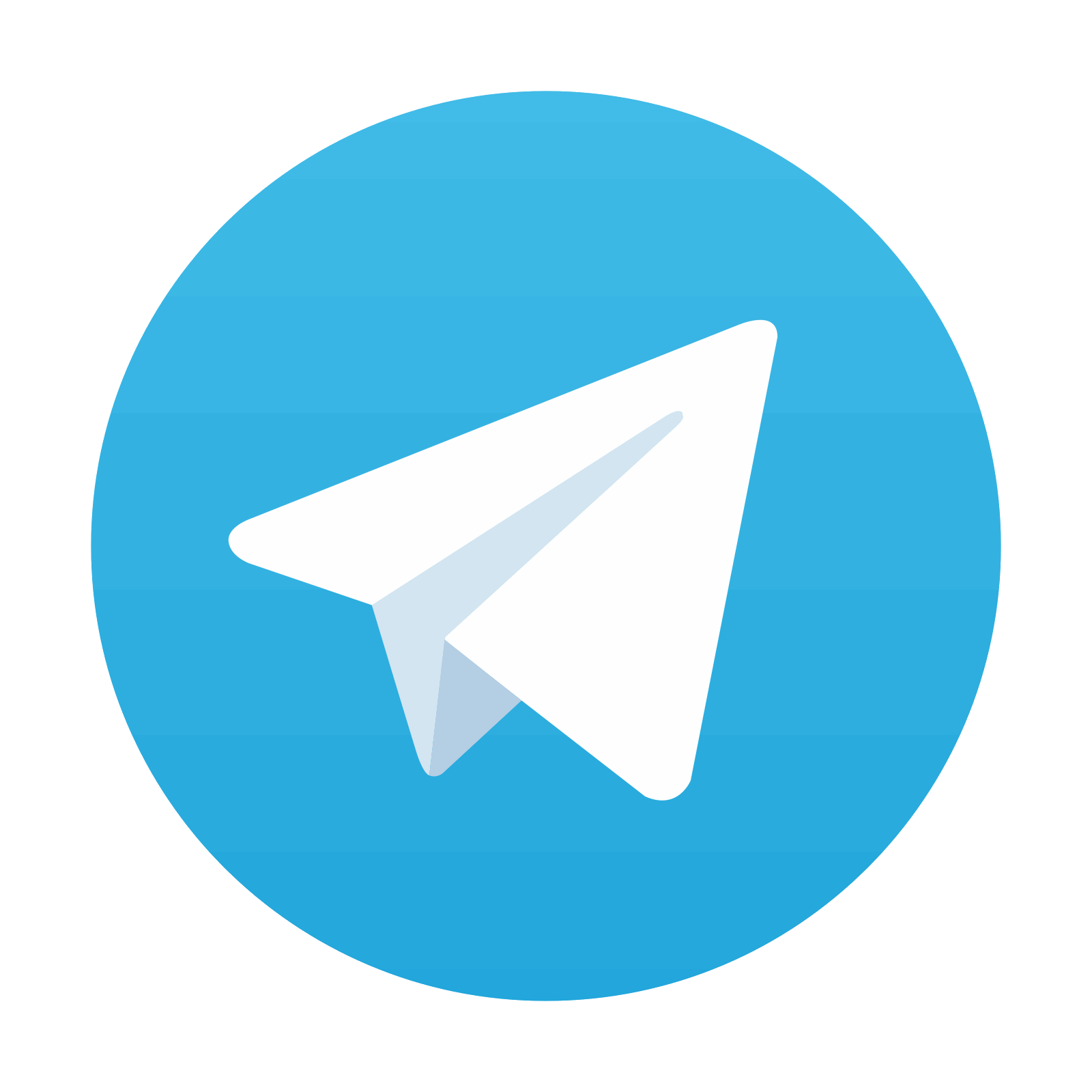
Stay updated, free articles. Join our Telegram channel

Full access? Get Clinical Tree
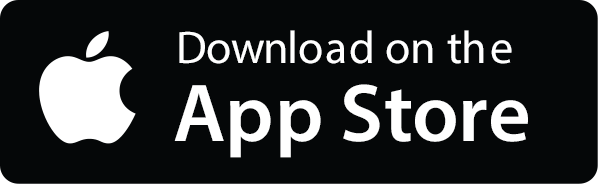
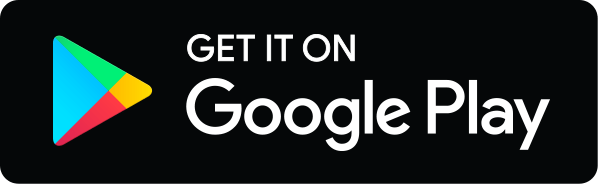