Laser
Wavelength (nm)
Delivery device
Scope
Depth of penetration
Uses
Coagulation
Cutting
Nd:YAG
1,060
Quartz fiber
RB/FB
0.5–1.5 cm
+++
+
CO2
10,600
Coupler and waveguide
RB
0.23 mm
+
+++
Argon
516
Quartz fiber
RB/FB
1.0–2.0 mm
++
+
KTP
532
Quartz fiber
RB/FB
1.0 mm
++
+
Nd:YAP
1,340
Quartz fiber
RB/FB
++++
+
How Do Lasers Work?
For the emission of laser light, a stimulus is used to apply energy to the lasing medium. The stimulus that excites the medium can be another laser, a flash lamp, or an intense electrical current. Energy is transferred from the stimulus to the lasing medium leading to movement of electrons to a more unstable, excited state. With the drop in energy of the electron back to its original state, photons, or small light particles, are emitted with a frequency and wavelength that are characteristic to the lasing medium. This emission of photons is the laser light which is directional, in phase, and monochromatic. The lasing medium is placed into a cavity with mirrors at either end, one that is completely reflective and one that is partially reflective (Fig. 35.1). As the photons bounce back and forth between the mirrors and through the medium, increasing numbers of excited electrons emit even more photons at the same wavelength and frequency. The percentage of coherent light emitted is an intrinsic property of the partially refractive mirror and varies between lasers.
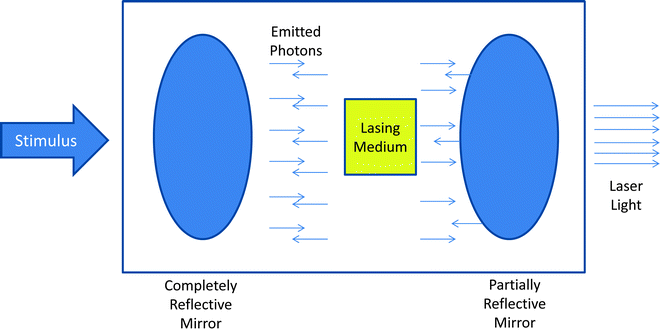
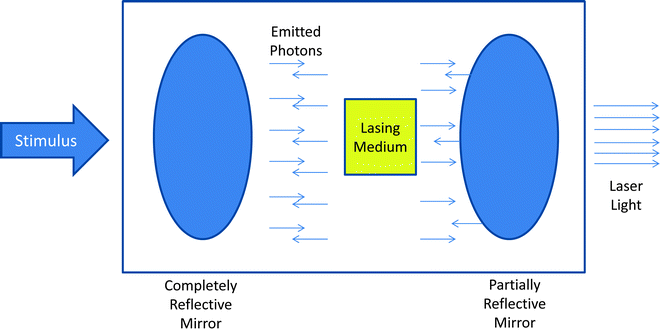
Fig. 35.1
Schematic of a theoretical laser system. As stimulus is applied through the lasing medium, exciting electrons which in turn release photons. These photons move in phase toward a partially reflective mirror. As some photons are reflected back through the lasing medium, there is amplification of the laser light that is generated. The resultant laser light emitted from the device is monochromatic, collimated, and coherent
Lasers can have thermal, photodynamic, and electromagnetic effects on the tissues they encounter, providing a tailored approach to the physical and clinical effects. Predominantly, laser bronchoscopy utilizes the thermal effects of light being transformed into heat as the laser interacts with living tissue. These effects are determined by the physical properties of the laser itself (i.e., wavelength) as well as the power density of the applied laser light. The power density (power in watts/area of application x time of pulse s) similarly determines the tissue–laser interaction. Lasers can be used for coagulation, leading to pale discoloration of the tissue associated with hemostatic effects. With more intense tissue interactions, carbonization occurs with blackish discoloration of the tissue and more robust destruction takes place. With even higher power densities, tissue vaporization can be achieved, although poor control of tissue effects ensues. Other forms of tissue destruction seen with medical laser use include photoablation, or removal of surface tissue or material, through thermal effects (i.e., CO2 lasers) versus a mode of destruction termed photodecomposition as achieved with ultraviolet-emitting excimer lasers that have the benefit of better control of hemostasis. Obviously, the properties of the laser employed yield distinct effects. Therefore, the selection of the proper laser for a given desired effect requires an understanding of the particular laser–tissue interaction.
A tissue property defining a laser’s ability to achieve hemostasis is related to the thermal relaxation time. The thermal relaxation time is the time by which a cylindrical structure (i.e., blood vessel) cools by diffusion. This is mathematically described by the following equation:


The importance of this equation is related to the operation of lasers used with intention of hemostasis. Essentially, if the pulse is longer than the cooling time, there will be a significant amount of heat that diffuses out of the target, and thus, destruction of surrounding tissues. Confined damage is best if laser pulse time is shorter than target cooling time.
Types of Lasers Used in Bronchoscopy
As previously stated, the particular characteristics of lasers are related to their lasing medium. In particular, the emitted wavelengths are discrete which determines the properties and their uses. Understanding of particular wavelength–tissue interaction is important in translation to clinical uses. Table 35.1 and Fig. 35.2 describe features of the different lasers used in airway interventions. Several features of the Nd:YAG (neodymium-doped yttrium aluminum garnet) laser make it ideal for use in the airway. With a wavelength of 1,060 nm, Nd:YAG lasers can be used through a flexible quartz fiber, which is a feature not available to the much longer wavelength CO2 (10,600 nm) laser.
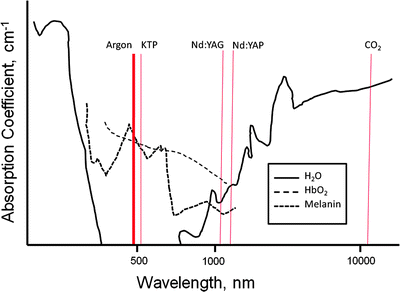
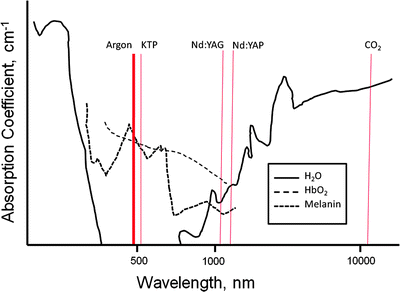
Fig. 35.2
Absorption coefficients for water, oxyhemoglobin, and melanin throughout the spectrum of medical lasers. The most frequently used medical laser wavelengths are indicated
In the original description, utilizing laser for control of central airway disease made use of the CO2 laser. Early in airway interventional laser therapy, this was used most often, but there are some difficulties inherent to this system. As the wavelength of CO2 lasers is high, it cannot be passed through flexible quartz. Since the CO2 laser is not “bendable,” it requires use of a rather cumbersome articulating arm system with multiple mirrors with closed ventilation. It has uses in central airway obstruction, but requires rigid bronchoscopy and is not able to reach upper lobe disease and can be difficult to aim depending on anatomy. Recently, a photonic bandgap fiber has been developed for use with a CO2 laser; however, experience with this system is limited at this time. The CO2 laser has applications as a precision cutting tool with visible penetration that can be predictable based on power density. This technique can help control vessels up to 0.5 mm. Systems for CO2 lasers utilize an articulating arm-mirror system with closed ventilation. It is most often used in conjunction with general anesthesia and rigid bronchoscopy. The lasing medium in CO2 lasers is a mixture of CO2, helium, and nitrogen. Most are equipped with a low-power red helium–neon aiming laser. Typically, the CO2 laser is used with a power output of 10–15 W with a pulse duration of 0.5–1 s.
The Nd:YAG (neodymium-doped yttrium aluminum garnet) laser with a wavelength of 1,060 nm can be passed through flexible quartz fibers. This laser utilizes an yttrium–garnet glass coated with neodymium. This laser has poor affinity for hemoglobin and water; therefore, it has deeper penetration and is more efficient for coagulation. Due to the scattering of laser energy associated with the Nd:YAG, the volume of adjacent tissue affected is determined by the power settings and the duration of firing. Carbonization and vaporization can be accomplished with higher power settings. This type of laser is good for much larger vessels up to 0.5 cm in diameter. Dark color (blue or black) increases absorption, and light surface colors enhance penetration. The power density (wattage per unit area) is below the surface of tissue and depends on color of the surface. The Nd:YAG laser in contrast to the CO2 laser functions more as a radiator with volumetric heating with better absorption in dark tissues. This type of laser is applied through the use of a flexible quartz system with variable quartz fibers (ranging from 1.9 to 2.4 mm). Fibers may be cooled through continuous jet of air or CO2 gas, but modern fibers may be used without cooling. With the Nd:YAG laser, there is a divergence of less than 10 ° with working distance of 5–10 mm between fiber tip and target area. This type of laser has better “tip control” through the use of the flexible fiber, thus upper airway treatments are possible as is steering. The downside of this control is a less predictable depth of penetration.
Argon lasers with a wavelength of 516 nm (blue green in visible spectrum) can similarly be used through a flexible quartz fiber, but suffers from much lower power; therefore, it is less useful for significant obstructing airway lesions. As it is highly absorbed by hemoglobin, its depth of penetration is attenuated, limiting its use for debulking airway masses; however, this leads to uses in airway coagulation which is outside the scope of this chapter and will be discussed elsewhere. There are other lasers that have been applied to airway therapeutics including combination lasers such as the CO2:Nd:YAG, KTP (potassium titanyl phosphate) lasers, holmium:YAG laser, and the Nd:YAP (neodymiumdoped yttrium aluminum perovskite) laser, but limited experience with these platforms in airway therapies exists.
Notable Issues and Modifiers of Lasers
As many lasers are not in the visible light spectrum, coaxial lasers with visible light (i.e., HeNe laser) can be utilized to help aim these devices.
Some laser systems require cooling. Coaxial flow of air (or nitrogen or CO2) has been used as a cooling source and to keep the laser tip clean. There are also internal (more mobile) or external (greater power at continuous exposure settings) water cooling sources for thermal control of lasers. A possible benefit of gas coolant systems is that they provide better visibility than fluid systems. Coolant systems can offer further safeguards through monitoring flow. If it is diminished by obstruction, controls can provide a safety measure to keep laser from firing. Inadequate flow leads to charring and fracturing of probes from fiber tip in contact probes. Modern fibers used with the Nd:YAG laser are often employed without the use of cooling systems.
Contact probes are an adjunct to laser therapies that provide tactile feedback to the operator and allow for decreased power through the use of direct contact of the laser through artificial sapphire crystal tips that are in direct contact with the tissue of interest. The tissue effects are augmented by direct contact, thus thermal gradients and power densities achieve therapeutic effects with lower overall power. The greater efficiency of energy utilization and greater control are potentially beneficial; however, cooling is required to decrease thermal damage to surrounding tissues, and visibility can be limited by close contact.
Type of Scope
Although there is an ability to use particular medical lasers through a flexible bronchoscope, utilization of rigid systems provide more secure control and immediate ability to intervene in the setting of potentially life-threatening complications. Significant debate has ensued surrounding the most appropriate scope to be used. Advantages of rigid systems include superior airway control, ease of removal of necrotic tissue, ease of ventilation, ability to tamponade areas of hemorrhage, improved visibility, and shorter operation time. Ventilation during intervention (out of the scope of this chapter) can be controlled better through rigid systems, and this approach provides larger visibility through greater working space. In the setting of high-grade lesions with a high propensity to bleed, a rigid system offers a greater ability to achieve hemostasis with preserved visibility. Also, flash fires have been reported much less frequently with rigid systems than with flexible scope use. Flexible systems do offer an ability to reach some distal lesions that are not approachable through rigid systems as well as a potential to “steer” the fiber tip with finer control. That being said, the use of combined flexible scopes when needed to reach distal disease through a rigid scope-secured airway offers the operator the most robust control. Appropriate uses of flexible systems include granulation tissue, distal disease, and nonobstructing lesions with the need for photocoagulation related to recurrent hemoptysis or in conjunction with a rigid intubation, namely, processes which are more readily controllable. At the present time, the overwhelming consensus is to perform laser airway therapy under general anesthesia with the use of rigid instrumentation if at all possible.
Indications, Contraindications, and Complications of Laser Bronchoscopy
The indication for laser airway therapy can be distilled to a simple concept, namely, to reduce airway obstruction to improve airflow and thus improve ventilation or palliate symptoms. Symptoms associated with obstruction are typically dyspnea, but cough, postobstructive infections, and hemoptysis are not infrequently encountered. The specific mechanism of obstruction can be quite varied and divided into malignant and nonmalignant processes. The term “benign” is often used to describe a non-neoplastic process; however, the course for many of these patients is far from benign. There are case reports and series describing laser use for nearly every potential cause of central airway obstruction from cancer as well as several processes that compromise airway lumen (Table 35.2). The types of lesions that are most amenable to laser intervention included polypoid endobronchial tumors with minimal submucosal infiltration or external compression. Table 35.3 outlines characteristics of airway lesions amenable to laser intervention.
Table 35.2
Indications for laser therapy in airway disease
Benign conditions | Malignant conditions | |
---|---|---|
Subglottic and tracheal stenosis | Cauterization of hemorrhage | |
Endobronchial granuloma | Adenocarcinoma | |
Broncholiths | Squamous cell carcinoma | |
Benign tumors/hamartomas | Bronchogenic carcinoma | |
Endobronchial inflammatory polyps | Carcinoid tumor | |
Endobronchial amyloidosis | Large-cell carcinoma | |
Tracheoesophageal fistulae | Small-cell carcinoma | |
Hemangiomas | Tumors metastatic to the airway: | |
Granulation tissue at transplant anastamosis | Thyroid | Thymus |
Tubercular bronchial stenosis | Colon | Uterus |
Dysplasia | Kidney | Testis |
Bronchial stenosis after sleeve resection | Esophagus | Bone |
Foreign body removal: | Melanoma | Larynx |
Retained sutures | Breast | Liver |
Tissue-embedded foreign bodies | Ovary |
Table 35.3
General characteristics of malignant lesions amenable to laser therapy and descriptions of favorable and unfavorable lesions
Tumor is not responsive to other reasonable therapeutic interventions | |
Tumor should protrude into the airway lumen without obvious extension beyond cartilage | |
Tumor length is small (<4 cm) | |
The lumen is visible | |
There is functional lung parenchyma distal to obstruction | |
The symptoms of are predominately respiratory | |
Favorable lesions | Unfavorable lesions |
Polypoid, pedunculated | External compression causing obstruction |
Large endobronchial component | Long, tapering obstruction |
Distal lumen visible | Extensive submucosal disease |
Lesion confined to trachea and mainstem bronchi | Total obstruction |
Short lesion length (<4 cm) | Upper lobe and segmental lesions |
Functional lung distal to obstruction | Chronic collapse |
Short duration of distal collapse | Longer duration of distal collapse |
Benign Uses
Ideally, there should be precise laser–tissue interactions through noncontact modes with adequate hemostasis. These are typically located in the subglottic larynx and trachea. CO2 lasers with very controlled depth of penetration, precise tissue interactions, and very little scatter are ideal. The relative inability to use them through fiber-optic systems however can be limiting. Also, due to the hypovascular nature of webs, scars, amyloid deposits, hemangiomas, and papillomas, the CO2 laser that does not require pigment for absorption can be useful. Also, there are less collateral effects. Treatment of stenosis of the subglottic region is typically more favorable than tracheal lesions, but both typically require repeated treatments. The Nd:YAG laser is often employed in benign conditions obstructing the airway at lower power settings with positive effects.
Malignant Uses
Nd:YAG lasers have characteristics beneficial to use in malignant central airway-obstructing lesions. The depth of penetration permits coagulation of vessels that are several millimeters in diameter. The laser causes thermal destruction of tumor cells as well as vaporization. Debulking of large lesions is possible with coincident coagulation which is vitally important due to hemorrhage developing from tumor necrosis and perforation of large vessels. Indications for treatment of distal airway disease include obstructive pneumonitis and recurrent or persistent hemoptysis.
Contraindications
The most important contraindication to laser bronchoscopy is the absence of an adequately trained operator. The technique can be performed very safely with significant efficacy in the well-trained operator’s hands; however, without appropriate knowledge of the technique, disastrous consequences may be realized. From an anatomical standpoint, a few specific attributes of the airway obstruction may yield laser airway therapy ineffective with one exception. There is no role for medical lasers in the treatment of lesions causing external compression of the central airways (i.e., bulky mediastinal tumor, mediastinal fibrosis). Extensive airway involvement of bulky tumors or locations providing aiming difficulty prevents successful application. Total luminal obstruction without visualization of distal airway and upper lobe disease are relative contraindications due to risk for perforation secondary to uncertain direction of distal airways. In the setting of profound or refractory hypoxemia, unstable cardiovascular status, or coagulopathy, operator discretion is advisable.
Complications of Laser Airway Interventions
Overall, complications of laser airway treatments are uncommon in the well-trained hand, but significant issues may result from improper pretreatment assessments of anatomical boundaries, failure to control the airway, inadequate visibility, or failure to control the surrounding environment (Table 35.4). Most commonly, respiratory compromise is encountered due to hemorrhage or destroyed tissue in the airway or anesthesia effects. Patients may experience rapid changes in PaO2 and PaCO2 with the limitations in FiO2 required for firing and suctioning and decreased airway luminal area due to instrumentation. Appropriate continuous monitoring is paramount to patient safety. Subsequent cardiovascular compromise is possible and accounts for most of the laser therapy-associated mortalities.
Table 35.4
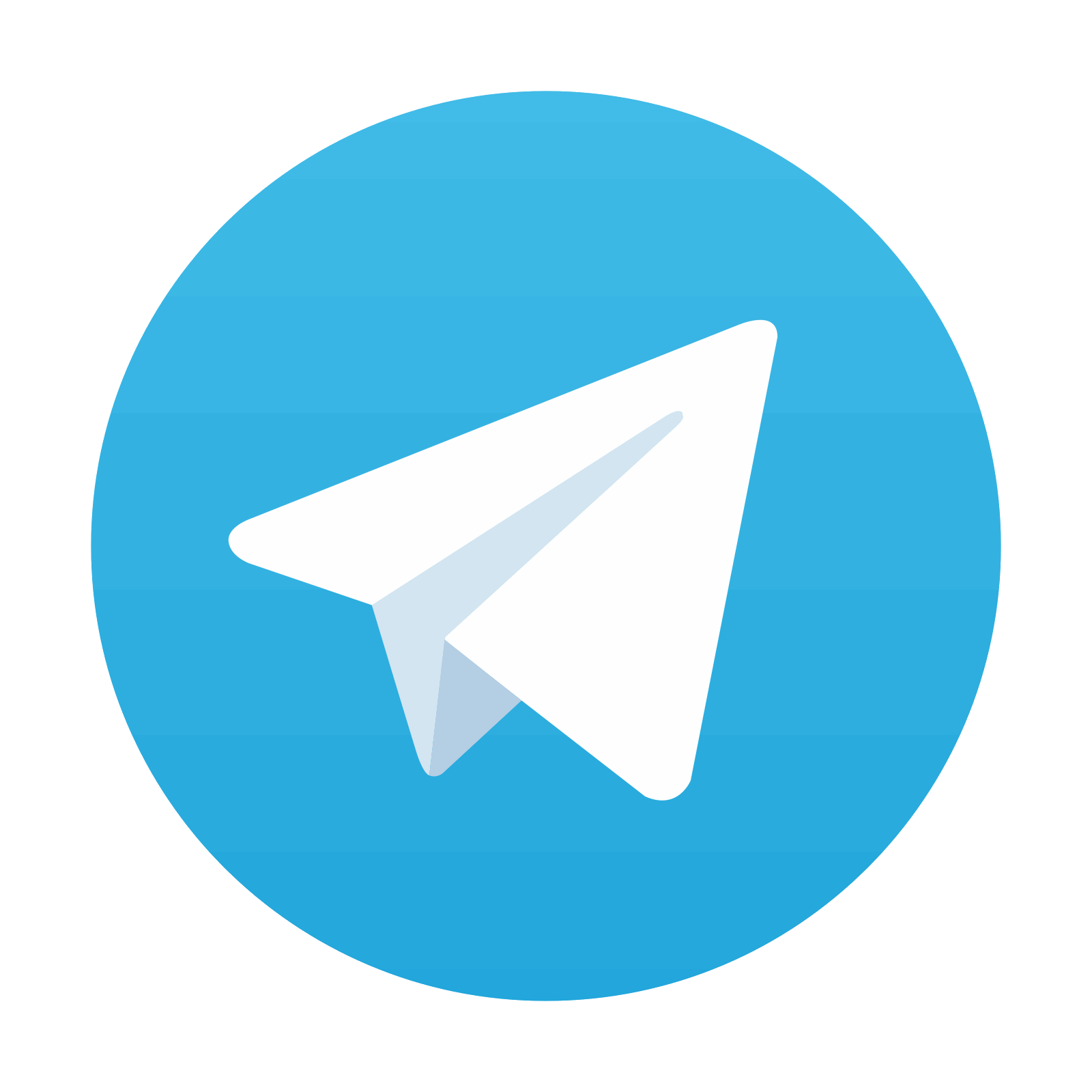
Complications of laser airway therapy
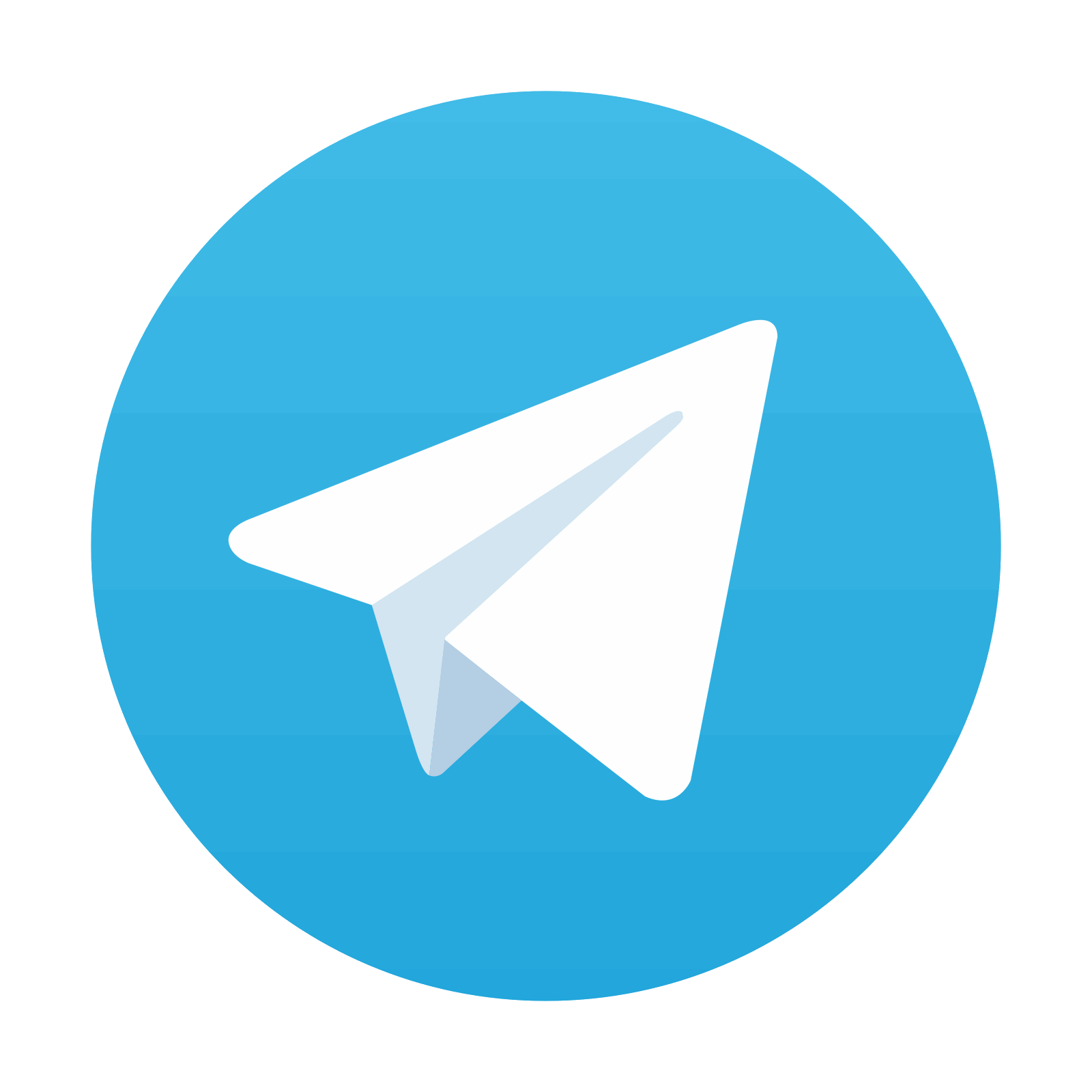
Stay updated, free articles. Join our Telegram channel

Full access? Get Clinical Tree
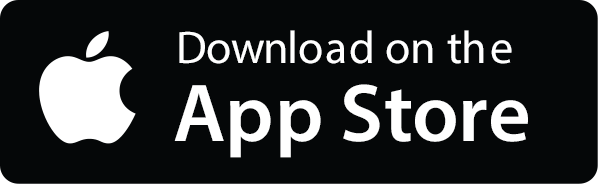
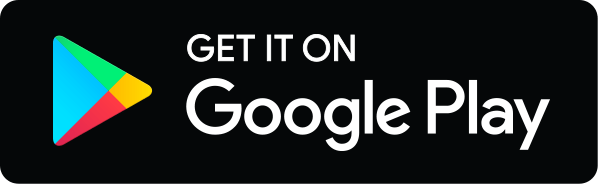
